Abstract
Butylated hydroxyanisole (BHA) is a synthetic phenolic compound consisting of a mixture of two isomeric organic compounds: 2-tert-butyl-4-hydroxyanisole and 3-tert-butyl-4-hydroxyanisole. We examined the effect of BHA against hydrogen peroxide (H2O2)-induced apoptosis in primary cultured mouse hepatocytes. Cell viability was significantly decreased by H2O2 in a dose-dependent manner. Additionally, H2O2 treatment increased Bax, decreased Bcl-2, and promoted PARP-1 cleavage in a dose-dependent manner. Pretreatment with BHA before exposure to H2O2 significantly attenuated the H2O2-induced decrease of cell viability. H2O2 exposure resulted in an increase of intracellular reactive oxygen species (ROS) generation that was significantly inhibited by pretreatment with BHA or N-acetyl-cysteine (NAC, an ROS scavenger). H2O2-induced decrease of cell viability was also attenuated by pretreatment with BHA and NAC. Furthermore, H2O2-induced increase of Bax, decrease of Bcl-2, and PARP-1 cleavage was also inhibited by BHA. Taken together, results of this investigation demonstrated that BHA protects primary cultured mouse hepatocytes against H2O2-induced apoptosis by inhibiting ROS generation.
Previous experimental evidences have indicated that the mechanisms underlying various types of hepatic injuries are mediated by robust generation of intracellular reactive oxygen species (ROS) [15,33]. Although ROS play normal physiological roles such as second messengers for normal cellular signaling, excessive ROS generation is known to cause cell damage and tissue injury due to an imbalance between pro- and antioxidants [19,38]. Generation of ROS during metabolism and other activities that exceeds the antioxidant capacity of a biological system gives rise to oxidative stress. Many researches have demonstrated that ROS, such as hydrogen peroxide (H2O2), superoxide anions, and singlet oxygen, are involved in many pathological conditions [21,25], and apoptotic cell death could be also promoted depending on the time of exposure and/or concentration of ROS [4,9]. Extracellular H2O2 has been used by investigators to inflict oxidative injury in a variety of cell types including neuronal progenitor cells, HepG2 cells, and hepatocytes at concentration ranging from 100 to 1,000 µM [16,22,29]. Previous studies indicated that extracellular H2O2 increases intracellular ROS levels via multiple mechanisms including loss of intracellular ROS antioxidants such as glutathione (GSH) [23] or decreased mitochondrial membrane permeability followed by mitochondrial ROS release [8]. Therefore, we used H2O2 to induce oxidative injury in primary mouse hepatocytes.
Recent reports suggest that several endogenous and exogenous antioxidants neutralize free radicals and protect the body by maintaining redox balance [27,34]. Antioxidants can perform protective roles against free radicals through a variety of different mechanism including catalytic systems to neutralize or divert ROS, binding or inactivation of metal ions to prevent ROS generation through a Harber-weiss reaction, scavenging and destroying ROS or absorbing energy and electron, and quenching of ROS [28]. Phenolic compounds commonly found in both edible and inedible plants have been reported to have multiple biological or medicinal properties as well as beneficial effects on health [2,17]. Accumulating evidence has indicated that phenolic compounds exert antioxidant effects via a number of mechanisms such as ROS-scavenging activity, regulation of ROS-removing enzymes, antioxidant-synthesizing enzymes, or ROS-forming enzymes [31]. Butylated hydroxyanisole (BHA; 3-tert-butyl 4-hydroxyanisole) is one of the most widely used synthetic phenolic compounds and is regarded as an effective food additive [14]. BHA is known to have various beneficial activities such as antioxidant activity, anti-inflammatory effects, and anticancer potential although some reports suggested that this reagent induces cytotoxicity [26,30,35,39]. However, direct evidence of the effect of BHA on ROS-induced hepatocyte apoptosis has not been obtained. Therefore, we investigated the ability of BHA to protect primary cultured mouse hepatocytes against H2O2-induced apoptosis.
Eight-week-old male ICR mice were purchased from Daehan Bio Link (Korea). All animal management procedures were conducted in accordance with the standard operation protocols established by Kyungpook National University (permission no. KNU 2012-119). A Cell Counting Kit-8 (CCK-8) was purchased from Dojindo Laboratories (Japan). BHA, H2O2, N-acetyl-Lcysteine (NAC), collagen, recombinant human insulin, dexamethasone, Williams' E medium and type IV collagenase were obtained from Sigma-Aldrich (USA). 5-(and 6)-chloromethyl-2',7'-dichlorodihydrofluorescein diacetate, acetyl ester (CM-H2DCFDA) were purchased from Life Technologies (USA). Fetal bovine serum (FBS) was acquired from Thermo Scientific (USA). Antibodies against Bax and Bcl-2 were obtained from New England BioLabs (USA). Anti-PARP-1, goat anti-rabbit IgG, and goat anti-mouse IgG antibodies were supplied by Santa Cruz Biotechnology (USA).
Primary hepatocytes were isolated from mouse liver using the two-step EDTA and collagenase perfusion method. After the mouse was anesthetized, the liver was perfused with Krebs-Henseleit buffer without Ca2 + or SO4 2 - (115 mM NaCl, 25 mM NaHCO3, 5.9 mM KCl, 1.18 mM MgCl2, 1.23 mM NaH2PO4, 6 mM glucose, and 0.1 mM EDTA) through the hepatic portal vein to remove the blood (flow rate of 7~9 mL/min for 5 min). The liver was then perfused with Krebs-Henseleit buffer without Ca2 + or SO4 2 - containing 0.02% collagenase and 0.1 mM CaCl2 until the liver appeared soft. Next, the liver was removed and gently minced, and the released cells were dispersed in Dulbecco's modified Eagle medium (DMEM; Life Technologies) containing 10% FBS and 1% penicillin/streptomycin (Life Technologies). The solution containing the mixed cells and debris was passed through a 100-µm cell strainer (BD Bioscience, USA). Subsequently, the filtrate was centrifuged at 50 × g for 3 min at 4℃. The isolated cells were washed three times with DMEM and then seeded in collagen-coated plates. The cells were maintained in DMEM containing high glucose (4.5 g/L) supplemented with 10% FBS, 1% penicillin/streptomycin, 1 µg/mL insulin, and 10- 12 M dexamethasone for 24 h at 37℃ in a humidified atmosphere (5% CO2). The cells were then incubated with fresh Williams' E medium without FBS 24 h prior to the experiments.
Cell viability was measured using a CCK-8 assay. Mouse hepatocytes were cultured in 96-well plates (BD Bioscience) in triplicate for each group. The cells were treated with H2O2 with or without pretreatment with BHA or NAC for 30 min and cultured at 37℃ for 24 h. And 10 µL of CCK-8 solution was added to each well followed by further incubation at 37℃ for 3 h. Absorbance was measured at 450 nm using a microplate reader (BioTek Instruments, USA). All values are expressed as the mean ± standard error (SE) of triplicate experiments. The values were converted from absolute counts to a percentage of the control.
Cells were directly lysed in culture dishes with RIPA buffer (Boston BioProducts, USA) supplemented with proteinase and phosphatase inhibitor cocktail mixture (Thermo Scientific). Cell lysates (30 µg) were separated using 10% or 12% SDS-polyacrylamide gel electrophoresis and then transferred to polyvinylidene fluoride (PVDF) membranes (Millipore, Germany). The blots were then washed with Tris-buffered solution containing Tween-20 (TBST; 10 mM Tris-HCl [pH 7.6], 150 mM NaCl, and 0.1% Tween-20), blocked with 5% skimmed milk in TBST for 1 h at room temperature, and incubated for 12 h at 4℃ with the appropriate primary antibodies against Bax, Bcl-2, and PARP-1 at a 1:1,000 dilution. The membranes were then washed with TBST and incubated with horseradish peroxidase-conjugated goat anti-rabbit or goat anti mouse IgG antibodies (1:5,000 dilution) for 12 h at 4℃. The bands were visualized using an enhanced chemiluminescence detection system (Thermo Scientific) according to the manufacturer's protocols.
CM-H2DCFDA (DCF-DA), which functions as a ROSsensitive fluorophore, was used to detect intracellular ROS generation. Mouse hepatocytes on 35-mm cell culture dishes (BD Bioscience) were treated with H2O2 with or without pretreatment with BHA or NAC for 30 min and cultured at 37℃ for 2 h. The cells were moved into the dark and incubated with 5 µM DCF-DA for 30 min at 37℃. Next, the cells were washed three times with PBS and viewed using fluorescence microscopy (DM IL LED Fluo; Leica, Germany) at 100 × magnification. Fluorescence intensity was measured using Tecan Infinite M200 Pro (Life Technologies).
To investigate the effect of H2O2 on primary mouse hepatocytes, cell viability was measured with a CCK-8 assay. Fig. 1A shows that treatment of mouse hepatocytes with H2O2 at concentrations ranging from 100 to 1,000 µM for 24 h led to a significant decrease in cell viability in a dose-dependent manner. We also evaluated the expression of apoptotic marker proteins. H2O2 promoted Bax expression, decreased Bcl-2 levels, and increased PARP-1 cleavage. The Bax/Bcl-2 ratio also significantly increased in a dose-dependent manner.
To determine the effect of BHA (panel A in Fig. 2) on decreased cell viability caused by H2O2, cells were pretreated with various concentrations (0~10 µM) of BHA for 30 min and then treated with 1,000 µM H2O2. BHA prevented the decreases in cell viability caused by H2O2 (panel B in Fig. 2). These results indicate that BHA pretreatment protects primary cultured mouse hepatocytes from H2O2-induced apoptosis.
In order to determine whether the protective effects of BHA were associated with antioxidant activities in H2O2-treated mouse hepatocytes, intracellular ROS levels were measured. As shown in panels A-G of Fig. 3, H2O2 significantly increased intracellular ROS levels. This was significantly attenuated by pretreatment with BHA (5 µM) or NAC, a common ROS scavenger (1,000 µM). NAC was used as a positive control.
As shown in panel A of Fig. 4, decreased cell viability due to H2O2 was significantly inhibited by pretreatment with BHA or NAC. These results were confirmed by observing cell morphology (panel B in Fig. 4). Increased Bax levels, decreased Bcl-2 expression, and cleavage of PARP-1 promoted by H2O2 were attenuated by pretreatment with BHA (panel C in Fig. 4). Additionally, increases of the Bax/Bcl-2 ratio due to H2O2 treatment were also decreased by BHA (panel D in Fig. 4).
Oxidative stress-induced cell damage has been implicated in various disorders. In particular, oxidative stress in the liver is related to apoptotic cell death that is associated with the development of liver disease [5]. H2O2 is a major ROS produced intracellularly during many physiological and pathological processes, and causes oxidative damage. This compound has been extensively used as an inducer of oxidative stress for in vitro models. Therefore, H2O2 was selected to promote oxidative damage in the current investigation.
It has been reported that BHA has an antioxidant effect possibly related to the attenuation of oxidative stress [11]. However, the mechanism underlying the hepatoprotective effect of BHA remains unclear. In the present study, it was demonstrated that BHA protected primary cultured mouse hepatocytes against H2O2-induced cytotoxicity by preventing oxidative stress and apoptosis.
Our results showed that H2O2 treatment significantly decreased cell viability while increasing Bax expression and reducing Bcl-2 levels. Members of the Bcl-2 family, which includes Bcl-2, Bcl-xL, Bad, and Bax, are important regulators of various apoptotic pathways [32]. It has been shown that Bax exerts pro-apoptotic effects whereas Bcl-2 possesses anti-apoptotic activity [37]. In general, Bcl-2 inhibits apoptosis by negatively regulating the apoptotic activity of Bax and forming Bcl-2/Bax heterodimers. The Bcl-2/Bax ratio can help determine whether a cell will live or die after being exposed to an apoptotic stimulus. In this study, a remarkable increase of the Bax/Bcl-2 ratio was observed after H2O2 treatment. Additionally, PARP-1 cleavage increased after H2O2 treatment. The cleavage of PARP is a hallmark of apoptosis [20]. PARP is known to be required for DNA repair. Pro-PARP is cleaved by activated apoptotic enzymes including caspase-3, a major mediator of apoptosis [6,13].
In this study, we demonstrated that H2O2 markedly increased cytotoxicity and intracellular ROS generation in mouse hepatocytes. Pretreatment with BHA significantly inhibited the decrease of cell viability as well as the increased intracellular ROS concentration. Additionally, our results showed that treatment with BHA down-regulates the H2O2-induced increase of the Bax/Bcl-2 ratio and PARP-1 cleavage. Previous studies have clearly shown that ROS induce apoptosis in hepatocytes [10,40]. ROS participate and regulate a diverse number of downstream signaling pathways that govern specific cellular functions [1,12] such as growth, metabolism, cell division, necrosis, apoptosis, and aging [7,18,24]. However, an imbalance in the formation and neutralization of ROS leads to oxidative stress [3]. Oxidative stress caused by ROS is responsible for a wide variety of cellular damage and is the most widely recognized mechanism of secondary injury [36]. Following oxidative stress, the overproduction of ROS and subsequent antioxidant depletion results in a total breakdown of the endogenous antioxidant defense mechanisms, culminating in the failure to protect cells from oxidative damage [16].
In conclusion, data from this study demonstrated that BHA possesses antioxidant activity. Additionally, pretreatment with BHA prevented apoptotic cell death induced by oxidative stress in mouse hepatocytes. Therefore, BHA may be beneficial for treating liver diseases caused by oxidative stress.
Figures and Tables
Fig. 1
Effect of hydrogen peroxide (H2O2) on the viability of primary cultured mouse hepatocytes. (A) Mouse hepatocytes were incubated with H2O2 at the indicated concentrations for 24 h. Cell viability was then measured by a CCK-8 assay. Values are expressed as the mean ± standard error (SE) of three independent experiments with triplicate dishes. *p < 0.05 vs. the control. (B) Cells were incubated with H2O2 at the indicated concentrations for 24 h. Cell lysates were subjected to Western blotting to measure the levels of Bax, Bcl-2, and PARP-1 expression. (C) The densities of bands corresponding to Bax and Bcl-2 protein were measured and the Bax/Bcl-2 ratio was calculated. Values are expressed as the mean ± SE of three independent experiments. *p < 0.05 vs. the control.
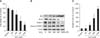
Fig. 2
Effect of butylated hydroxylanisole (BHA) on H2O2-induced cytotoxicity. (A) Chemical structure of BHA. (B) Mouse hepatocytes were incubated with 1,000 µM H2O2 for 24 h with or without BHA pretreatment at the indicated concentrations for 30 min. Cell viability was measured with a CCK-8 assay. The values are expressed as the mean ± SE of three independent experiments. *p < 0.05 vs. the control; **p < 0.05 vs. H2O2 alone.
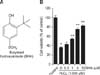
Fig. 3
Effect of BHA on H2O2-induced ROS generation. (A~F) Dichlorofluorescein (DCF)-sensitive cellular ROS generation was assessed. (A) Control. (B) Treatment with 1,000 µM H2O2 for 2 h. (C) Pretreatment with 5 µM BHA for 30 min before exposure to 1,000 µM H2O2 for 2 h. (D) Incubtion with with BHA for 2 h. (E) Pretreatment with 1,000 µM N-acetyl-cysteine (NAC) for 30 min before exposure to 1,000 µM H2O2 for 2 h. (F) Incubation with NAC for 2h. (G) DCF-DA fluorescence was measured and quantified with a fluorometer. Values are expressed as the mean ± SE of three independent experiments. *p < 0.05 vs. the control, **p < 0.05 vs. H2O2 alone. Scale bars = 50 µm (A~F).
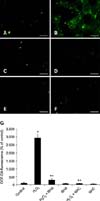
Fig. 4
Effect of BHA on H2O2-induced apoptosis. (A) Mouse hepatocytes were pretreated with 5 µM BHA or 1,000 µM NAC for 30 min and then incubated with or without 1,000 µM H2O2 for 24 h. Cell viability was measured with a CCK-8 assay. Values are expressed as the mean ± SE of three independent experiments with triplicate dishes. *p < 0.05 vs. control; **p < 0.05 vs. H2O2 alone. (B) Cells were pretreated with 5 µM BHA followed by treatment with 1,000 µM H2O2 for 24 h. Scale bar = 50 µm. (C) Cells were pretreated with 5 µM BHA and then incubated with 1,000 µM H2O2 for 24 h. Cell lysates were subjected to Western blotting to measure the levels of Bax, Bcl-2, and PARP-1 expression. (D) Densities of bands corresponding to Bax and Bcl-2 protein were measured, and the Bax/Bcl-2 ratio was calculated. Values are expressed as the mean ± SE of three independent experiments. *p < 0.05 vs. the control , **p < 0.05 vs. H2O2 alone.
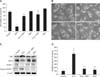
Acknowledgments
This work was supported by a grant from Kyungpook National University Research Fund, 2013 (Korea); the Next-Generation BioGreen 21 Program (no. PJ009090), Rural Development Administration, Korea; and a National Research Foundation (NRF) grant funded by the Korean government (MSIP; NRF-2012R1A4A1028835).
References
1. Aviram M. Review of human studies on oxidative damage and antioxidant protection related to cardiovascular diseases. Free Radic Res. 2000; 33:Suppl. S85–S97.
2. Cai Y, Luo Q, Sun M, Corke H. Antioxidant activity and phenolic compounds of 112 traditional Chinese medicinal plants associated with anticancer. Life Sci. 2004; 74:2157–2184.


3. Chen KC, Zhou Y, Zhang W, Lou MF. Control of PDGF-induced reactive oxygen species (ROS) generation and signal transduction in human lens epithelial cells. Mol Vis. 2007; 13:374–387.
4. Cole KK, Perez-Polo JR. Poly(ADP-ribose) polymerase inhibition prevents both apoptotic-like delayed neuronal death and necrosis after H2O2 injury. J Neurochem. 2002; 82:19–29.


5. Conde de la Rosa L, Schoemaker MH, Vrenken TE, Buist-Homan M, Havinga R, Jansen PLM, Moshage H. Superoxide anions and hydrogen peroxide induce hepatocyte death by different mechanisms: involvement of JNK and ERK MAP kinases. J Hepatol. 2006; 44:918–929.


6. Daniel H. Molecular and integrative physiology of intestinal peptide transport. Annu Rev Physiol. 2004; 66:361–384.


7. de Magalhães JP, Church GM. Cells discover fire: employing reactive oxygen species in development and consequences for aging. Exp Gerontol. 2006; 41:1–10.
8. Dumont A, Hehner SP, Hofmann TG, Ueffing M, Dröge W, Schmitz ML. Hydrogen peroxide-induced apoptosis is CD95-independent, requires the release of mitochondria-derived reactive oxygen species and the activation of NF-κ B. Oncogene. 1999; 18:747–757.


9. Englert RP, Shacter E. Distinct modes of cell death induced by different reactive oxygen species: amino acyl chloramines mediate hypochlorous acid-induced apoptosis. J Biol Chem. 2002; 277:20518–20526.


10. Evans ZP, Mandavilli BS, Ellett JD, Rodwell D, Fariss MW, Fiorini RN, Schnellmann RG, Schmidt MG, Chavin K. Vitamin E succinate enhances steatotic liver energy status and prevents oxidative damage following ischemia/reperfusion. Transplant Proc. 2009; 41:4094–4098.


11. Fang L, Gou S, Fang X, Cheng L, Fleck C. Current progresses of novel natural products and their derivatives/analogs as anti-Alzheimer candidates: an update. Mini Rev Med Chem. 2013; 13:870–887.


12. Halliwell B. Free radicals, antioxidants, and human disease: curiosity, cause, or consequence? Lancet. 1994; 344:721–724.


13. Ishikawa K, Takenaga K, Akimoto M, Koshikawa N, Yamaguchi A, Imanishi H, Nakada K, Honma Y, Hayashi J. ROS-generating mitochondrial DNA mutations can regulate tumor cell metastasis. Science. 2008; 320:661–664.


14. Iverson F. In vivo studies on butylated hydroxyanisole. Food Chem Toxicol. 1999; 37:993–997.
15. Jaeschke H. Reactive oxygen and mechanisms of inflammatory liver injury. J Gastroenterol Hepatol. 2000; 15:718–724.


16. Jiang J, Yu S, Jiang Z, Liang C, Yu W, Li J, Du X, Wang H, Gao X, Wang X. N-acetyl-serotonin protects HepG2 cells from oxidative stress injury induced by hydrogen peroxide. Oxid Med Cell Longev. 2014; 2014:310504.


17. Kähkönen MP, Hopia AI, Vuorela HJ, Rauha JP, Pihlaja K, Kujala TS, Heinonen M. Antioxidant activity of plant extracts containing phenolic compounds. J Agric Food Chem. 1999; 47:3954–3962.


18. Kim KC, Lee C. Curcumin induces downregulation of E2F4 expression and apoptotic cell death in HCT116 human colon cancer cells; involvement of reactive oxygen species. Korean J Physiol Pharmacol. 2010; 14:391–397.


19. Lee MY, Jung SC, Lee JH, Han HJ. Estradiol-17β protects against hypoxia-induced hepatocyte injury through ER-mediated upregulation of Bcl-2 as well as ER-independent antioxidant effects. Cell Res. 2008; 18:491–499.


20. Li WM, Liu HT, Li XY, Wu JY, Xu G, Teng YZ, Ding ST, Yu C. The effect of tetramethylpyrazine on hydrogen peroxide-induced oxidative damage in human umbilical vein endothelial cells. Basic Clin Pharmacol Toxicol. 2010; 106:45–52.


21. Loguercio C, Federico A. Oxidative stress in viral and alcoholic hepatitis. Free Radic Biol Med. 2003; 34:1–10.


22. Lu Y, Zhang YY, Hu YC, Lu YH. Protective effects of 2',4'-dihydroxy-6'-methoxy-3',5'-dimethylchalcone against hydrogen peroxide-induced oxidative stress in hepatic L02 cell. Arch Pharm Res. 2014; 37:1211–1218.


23. Martin D, Salinas M, Fujita N, Tsuruo T, Cuadrado A. Ceramide and reactive oxygen species generated by H2O2 induce caspase-3-independent degradation of Akt/protein kinase B. J Biol Chem. 2002; 277:42943–42952.
24. Menon SG, Goswami PC. A redox cycle within the cell cycle: ring in the old with the new. Oncogene. 2007; 26:1101–1109.


25. Moriya K, Nakagawa K, Santa T, Shintani Y, Fujie H, Miyoshi H, Tsutsumi T, Miyazawa T, Ishibashi K, Horie T, Imai K, Todoroki T, Kimura S, Koike K. Oxidative stress in the absence of inflammation in a mouse model for hepatitis C virus-associated hepatocarcinogenesis. Cancer Res. 2001; 61:4365–4370.
26. Murakami Y, Shoji M, Ogiwara T, Tanaka S, Yokoe I, Fujisawa S. Preventive effect of ortho dimer of butylated hydroxyanisole on activator protein-1 activation and cyclooxygenase-2 expression in macrophages stimulated by fimbriae of Porphyromonas gingivalis, an oral anaerobe. Anticancer Res. 2006; 26:2915–2920.
27. Pham-Huy LA, He H, Pham-Huy C. Free radicals, antioxidants in disease and health. Int J Biomed Sci. 2008; 4:89–96.
28. Rajendran P, Nandakumar N, Rengarajan T, Palaniswami R, Gnanadhas EN, Lakshminarasaiah U, Gopas J, Nishigaki I. Antioxidants and human diseases. Clin Chim Acta. 2014; 436:332–347.


29. Ruiz C, Casarejos MJ, Gomez A, Solano R, de Yebenes JG, Mena MA. Protection by glia-conditioned medium in a cell model of Huntington disease. PLoS Curr. 2012; 4:e4fbca54a2028b.


30. Saito M, Sakagami H, Fujisawa S. Cytotoxicity and apoptosis induction by butylated hydroxyanisole (BHA) and butylated hydroxytoluene (BHT). Anticancer Res. 2003; 23:4693–4701.
31. Sandoval-Acuña C, Ferreira J, Speisky H. Polyphenols and mitochondria: an update on their increasingly emerging ROS-scavenging independent actions. Arch Biochem Biophys. 2014; 559:75–90.


32. Sarada SK, Himadri P, Ruma D, Sharma SK, Pauline T, Mrinalini . Selenium protects the hypoxia induced apoptosis in neuroblastoma cells through upregulation of Bcl-2. Brain Res. 2008; 1209:29–39.


33. Tribble DL, Aw TY, Jones DP. The pathophysiological significance of lipid peroxidation in oxidative cell injury. Hepatology. 1987; 7:377–386.


34. Valko M, Leibfritz D, Moncol J, Cronin MT, Mazur M, Telser J. Free radicals and antioxidants in normal physiological functions and human disease. Int J Biochem Cell Biol. 2007; 39:44–84.


35. Vandghanooni S, Forouharmehr A, Eskandani M, Barzegari A, Kafil V, Kashanian S, Ezzati Nazhad Dolatabadi J. Cytotoxicity and DNA fragmentation properties of butylated hydroxyanisole. DNA Cell Biol. 2013; 32:98–103.


36. Wang CC, Fang KM, Yang CS, Tzeng SF. Reactive oxygen species-induced cell death of rat primary astrocytes through mitochondria-mediated mechanism. J Cell Biochem. 2009; 107:933–943.


37. Wang X. The expanding role of mitochondria in apoptosis. Genes Dev. 2001; 15:2922–2933.
38. Weng D, Lu Y, Wei Y, Liu Y, Shen P. The role of ROS in microcystin-LR-induced hepatocyte apoptosis and liver injury in mice. Toxicology. 2007; 232:15–23.

