Abstract
New enrofloxacin microspheres were formulated, and their physical properties, lung-targeting ability, and tissue distribution in rats were examined. The microspheres had a regular and round shape. The mean diameter was 10.06 µm, and the diameter of 89.93% of all microspheres ranged from 7.0 µm to 30.0 µm. Tissue distribution of the microspheres was evaluated along with a conventional enrofloxacin preparation after a single intravenous injection (7.5 mg of enrofloxacin/kg bw). The results showed that the elimination half-life (t1/2β) of enrofloxacin from lung was prolonged from 7.94 h for the conventional enrofloxacin to 13.28 h for the microspheres. Area under the lung concentration versus time curve from 0 h to ∞ (AUC0-∞) was increased from 11.66 h·µg/g to 508.00 h·µg/g. The peak concentration (Cmax) in lung was increased from 5.95 µg/g to 93.36 µg/g. Three lung-targeting parameters were further assessed and showed that the microspheres had remarkable lung-targeting capabilities.
Enrofloxacin (ENR) is a type of synthetic fluoroquinolone. This compound has been specifically used in veterinary clinics [13] and acts by inhibiting bacterial DNA gyrase [417]. In vitro study has shown that ENR acts against a broad spectrum of microbes, including Gram-negative and Gram-positive bacteria and Mycoplasma spp. [2]. Ciprofloxacin is an active de-ethylated metabolite of ENR [20]. In addition to metabolic elimination, ENR is mainly excreted via tubular excretion and glomerular filtration [19].
Respiratory disease is the most common disorder treated in veterinary clinics. ENR used to be administered in food to animals to treat respiratory infections due to its high antimicrobial activity against many respiratory pathogens [61424] and favorable pharmacokinetic properties [251127]. Fluoroquinolones (e.g., ciprofloxacin) are also commonly used to treat adult humans with infections such as typhoid fever and diarrhea caused by Escherichia coli. Human infection with fluoroquinolone-resistant pathogenic species has become increasingly common and is associated with consumption of edible animal tissues. Tolerability concerns have led to restrictions on the use of fluoroquinolones in food animals in some countries. However, ENR is still registered for use in poultry, pigs, cattle, cats, and dogs in China.
It is known that antibiotics administered to target site can eliminate bacteria most effectively and antibiotic concentrations at the target site are more relevant than plasma drug concentrations to predict therapeutic efficacy. In addition, conventional ENR preparations used in veterinary clinics are associated with some adverse effects such as gastrointestinal reactions, allergic hypersensitivity, and production of resistant bacteria [1828]. Thus, it is of interest to develop new lung-targeting preparations to obtain the maximum therapeutic effect while minimizing side effects. Considering the fact that a large number of target animals (e.g., dogs and cats) would be sacrificed during tissue distribution experiments, rodents were chosen as the primary model species to evaluate the lung-targeting abilities of different preparations. The objectives of this study were to develop and characterize an ENR microsphere (ENR-MIC), and determine whether this microsphere can target the lung in rats.
An ENR reference standard (99.8%, lot no. HOO80904) was obtained from the China Institute of Veterinary Drug Control (China). ENR raw material (99.0%) was purchased from Zhejiang Hisun Pharmaceutical (China). A conventional ENR injection (ENR-INJ; 10 mL, 50 mg, lot no. 20090301) was obtained from Eastern Along Pharmaceutical (China). Poly-(lactic-co-glycolic acid) (PLGA; lactic acid : glycolic acid = 50 : 50, MW = 15,000, lot no. 090813) was purchased from Shandong Freda Medical Device (China). Methanol and acetonitrile of high-performance liquid chromatography (HPLC) grade were purchased from Sigma Chemical (USA). Phosphate buffered saline (PBS) was used as the buffer to measure in vitro drug release. Other reagents were of analytical grade and obtained in China.
One hundred and fifty-six healthy Sprague-Dawley rats (78 males and 78 females) 6 weeks old and weighing from 243 g to 256 g were purchased from Guangdong Center of Medical Laboratory Animal (China). The animals were kept in a temperature- (21℃~22℃) and humidity- (70%) controlled room. They were acclimatized for at least 7 days before drug administration. Food and water were available ad libitum. This investigation (animal study protocol no. 201004003) was approved by the Institutional Animal Care and Use Committee of South China Agricultural University.
PLGA was chosen to prepare the ENR-MICs using the spray drying method. Briefly, a laboratory scale spray dryer (series SY-6000; Shiyuan Bio, China) was used to prepare ENR-MICs. The optimal experimental conditions were identified with a single factor and orthogonal experimental design: the inlet and outlet air temperature were set at 55℃ ± 2℃ and 40℃ ± 2℃, respectively; the spray flow control was 550 µL/h, the pump had a feed spray rate of 4.0 mL/min to 4.5 mL/min, the aspirator level was 100%, and the atomization pressure was 6 bar. An appropriate volume of dichloromethane (DCM) was used to dissolve the PLGA, ENR raw material, and other additives. The solution was then spray-dried until no more microspheres could be produced. The microspheres were collected and stored in a vacuum desiccator at room temperature.
The microspheres were first suspended in a 0.9% sodium chloride solution. The microsphere appearance and particle size were measured using a micrometer with light microscopy (Olympus, Japan). The microspheres were mounted on aluminum studs and sputter-coated with gold. The gold-coated samples were then evaluated with scanning electron microscopy (Hitachi 5-4100; Hitachi, Japan).
An HPLC method was developed to quantify the ENR concentration in the microspheres. Briefly, 20 mg of ENR-MIC samples were dissolved in 1 mL DCM. The samples were subjected to extraction twice with 2 mL of 0.1 mol/L sodium hydroxide and then centrifuged at 4,000 × g for 10 min. The total supernatant was collected and mixed. Next, 0.4 mL of this supernatant was transferred to a 10-mL volumetric flask, diluted with water to volume, and mixed. Subsequently, 1 mL of dilute supernatant was transferred to a sample vial.
A Waters 2695-2487 Series HPLC system (Waters, USA) was used to inject the processed samples (10 µL) into a Hypersil BDS-C18 column (4.6 mm × 250 mm, 5 µm; Dalian Elite Analytical Instruments, China) kept at 30℃. The mobile phase contained phosphoric acid-triethylamine buffer (pH 2.4) and acetonitrile (81 : 19, v : v), and was delivered at a flow rate of 1 mL/min. A fluorescence detector was used to identify ENR with excitation and emission wavelengths of 278 nm and 456 nm, respectively.
For calibration, a series of ENR working standard solutions was subjected to extraction and analysis as described above. Loading rate for the ENR-MICs is expressed as a percentage and calculated as the ratio of drug content in the microspheres to microsphere weight. Three batches of microspheres were collected and tested to calculate the drug-loading rate.
A total of 1 g of microspheres was dissolved in 4 mL PBS (pH 7.4). The solution was then submerged in a dialysis tub and placed in a beaker containing 200 mL PBS at 37 ± 0.5℃ with continuous stirring at 100 rpm. Three mL of dialyzed solution was collected at 0.5, 1, 2, 4, 8, and 12 h after the start of dialysis, and 3 mL PBS (pH 7.4) was added after each sampling. ENR raw material was subjected to the same procedures as the ENR-MICs. For both the ENR-MICs and ENR raw material, three replicates were processed to evaluate the in vitro drug release. ENR concentration in each sample was determined using the HPLC method described above. The ENR concentration in each sample was used to calculate the mean cumulative release rate, and then to fit the kinetic equation for in vitro release. Release profiles of ENR from the microspheres were fitted using zero- and first-order kinetics along with Higuchi, Weibull, Baker-Lonsdale, Hixon-Crowell, and Riger-Peppas models.
One hundred and fifty-six healthy rats were equally divided into two groups. Each group was further divided into 12 sub-groups (three males and three females per sub-group). Rats in Group 1 were treated with ENR-MICs suspended in a sterile saline solution at a concentration of 7.5 mg ENR/kg bw delivered by caudal intravenous injection. Animals in Group 2 intravenously received the conventional ENR-INJ at the same dose. From both groups, a sub-group of rats was randomly chosen for sacrifice at 0 (before administration), 0.083, 0.25, 0.5, 1, 2, 4, 8, 12, 24, 48, 72, and 96 h after drug administration. Blood, muscle, kidney, liver, and lung were collected. Plasma was then obtained by centrifugation while the tissues were blotted dry and immediately homogenized. Plasma and tissue samples were immediately frozen and stored at -20℃ for further analysis.
ENR and ciprofloxacin in plasma were extracted according to our previously described method [31]. Briefly, 0.5 mL of plasma was transferred to a 2-mL centrifuge tube. A total of 1 mL methanol was then added to extract ENR and ciprofloxacin, vortexed for 3 min, and centrifuged at 12,000 × g for 15 min. The supernatant was passed through a 0.22-µm syringe filter into a sample vial.
Extraction of the drugs from tissues was performed using a previously published method [27] with minor modifications. Briefly, 0.5 g of homogenized tissue was placed in a centrifuge tube and mixed with 0.2 M phosphate buffer (0.5 mL). The extraction protocol was performed twice with 3 mL of DCM. For each round of extraction, the solution was vortexed for 1 min, sonicated for 10 min, and centrifuged for 10 min at 8,000 × g. The total supernatant was evaporated under a stream of air at 30℃. The remaining residue was dissolved in 0.5 mL mobile phase, vortexed for 1 min, and centrifuged for 15 min at 12,000 × g. The supernatant was transferred to a sample vial.
Conditions for chromatographic detection of the drugs in rat plasma and tissues were the same as those listed above. The method presented here was linear for both ENR (from 0.05 µg/mL to 5 µg/mL) and ciprofloxacin (from 0.02 µg/g to 10 µg/g) in plasma and tissues, respectively. For both reagents, the limits of quantification and detection of this assay were 0.05 µg/mL and 0.03 µg/mL in plasma, respectively, and 0.02 µg/g and 0.01 µg/g in tissues, respectively. These values were calculated as the ratio of signal to noise > 10 and > 3, respectively. For precision and accuracy, three replicates for both drugs at three different concentrations (0.05, 0.5, and 5 µg/mL or µg/g) were included to determine the coefficient of variation (CV) and measure recovery, respectively. The results indicated that both inter-day and intra-day CVs were below 5.6%, and recovery of ENR and ciprofloxacin was above 76.1% in plasma and tissues.
For Groups 1 and 2, the average ENR concentration at each sampling time point was calculated. Values for the mean concentration versus time were subjected to non-compartmental analysis using Phoenix WinNonlin (ver. 6.1; Pharsight, USA) to calculate the pharmacokinetic parameters of the ENR-MICs and conventional ENR-INJ. The areas under the concentration versus time curve from 0 h to ∞ (AUC0-∞) were calculated using the linear trapezoidal method. Peak concentration (Cmax) of ENR in tissues and time to reach Cmax (Tmax) were directly obtained from the concentrations versus time data. Ciprofloxacin was only detected in some samples at trace levels. Therefore, the pharmacokinetic parameters of this reagent were not evaluated. Furthermore, three main targeting parameters, relative intake rate (Re), targeting efficacy (Te), and ratio of peak concentration (Ce) [10], were calculated based on AUC0-∞ and Cmax to evaluate lung-targeting abilities. These values are expressed as ratios of AUCENR-MIC to AUCENR-INJ, AUCtargeting organ to AUCuntargeting organ, and Cmax-ENR-MIC to Cmax-ENR-INJ, respectively. Statistical differences in the pharmacokinetic and targeting parameters between Group 1 and 2 were evaluated with Duncan's multiple-range test and an ANOVA using SPSS statistical software (ver. 11.0; SPSS, USA). A p value < 0.05 was considered significant.
The drug-loading rate (27.57% ± 2.87%) was calculated for three batches of microspheres. Scanning electron and optical microscopy demonstrated that the ENR-MICs were regular and round in shape while ENR raw material had a granular structure (Fig. 1). Particle sizes and their distribution are shown in Fig. 2. The mean diameter of all microspheres was 10.06 µm, and 89.93% of all microspheres had a diameter ranging from 7.0 µm to 30.0 µm.
An in vitro release test which lasted 12 h was carried out in PBS for the ENR-MICs and ENR raw material; the dissolution rate was also measured under the same conditions. The results are shown in Fig. 3. In the first two hours, about 20% of the ENR in the ENR-MICs was released, while more than 90% of the ENR in ENR raw material was dissolved. Coupled with all the data acquired during the entire 12 h, the release rate for the ENR-MICs was found to be much slower than that for the ENR raw material. The in vitro release profile of ENR from the ENR-MICs was best expressed by a zero-order kinetic equation: in which t is the release time, Q is the percent of the drug released, and R is the correlation coefficient. Based on this equation, the release half-life was 5.0 h. For ENR-MICs, 85.4% of the ENR was released in 12 h while 99.8% of the ENR raw material was released in 4 h under the same conditions (Fig. 3).
After one single intravenous injection of ENR-MICs, the ENR and ciprofloxacin concentrations versus time data were acquired (Fig. 4 and Table 1, respectively). Pharmacokinetic parameters of the ENR are shown in Table 2. The corresponding concentrations of ENR and ciprofloxacin along with the pharmacokinetic parameters for the ENR-INJ are presented in Fig. 5 and Tables 3 and 4, respectively. The ENR concentrations in lung at all sampling time points after administration of the conventional preparation were significantly lower (p < 0.01) than those measured after delivery of the microspheres. In the other tissues, this trend was reversed and no significant differences were observed. The elimination half-life (t1/2β) of enrofloxacin from lung was prolonged from 7.94 h for the ENR-INJ to 13.28 h for the microspheres. Additionally, the AUC0-∞ for lung was increased from 11.66 h·µg/g to 508.00 hh·µg/g, and the Cmax for lung was also increased from 5.95 µg/g to 93.36 µg/g. After intravenous injection of the microspheres and conventional preparation, low levels of ciprofloxacin were identified in some plasma and tissues samples (Tables 1 and 3). However, the ciprofloxacin concentrations were inconsistent. Therefore, the pharmacokinetic parameters for ciprofloxacin were not calculated.
Three main targeting parameters, Re, Te, and Ce (Table 5), were calculated based on Cmax and AUC0-∞ values presented in Tables 2 and 4. The results showed that the Re for ENR-MICs in lung (43.57) was far greater than those (0.38~0.92) for other tissues. The Tes for the ENR-MICs in other tissues (23.83~240.76) was much greater than that in lung (1.00). The Te values for the ENR-INJ in lung, liver, kidney, muscle, and plasma were 1.00, 0.40, 0.43, 1.24, and 2.11, respectively. The targeting ratios (Te-ENR-MIC/Te-ENR-INJ) in lung, muscle, liver, kidney, and plasma were 1.00, 47.36, 59.34, 93.74, and 113.98, respectively. Finally, the Ce for the ENR-MICs in lung was 15.69, which was much greater than values (0.49~1.09) for the microspheres in other tissues.
ENR has been widely used to treat respiratory tract infections in veterinary clinics. It is known that antibiotics administered directly to a target site can most effectively eliminate bacteria. In order to obtain the optimal therapeutic effect without incurring adverse side effects, a lung-targeting ENR preparation is urgently needed. Taking into account pharmacokinetic, pharmaceutical, pharmacodynamic, and commercial factors, lung-targeting technology has become an attractive method for developing such a preparation. Much attention is being given to targeting microspheres [921222329], especially ones that are biodegradable [71826]. PLGA has been widely used to load many proteins, drugs, and other factors [151625] because this compound allows control over the rates of degradation and drug release.
An ENR microsphere was previously prepared by emulsifying gelatin and liquid paraffin [27]. The ENR-MICs presented in the current study were prepared using a spray drying method. The drug-loading rate for the previously described microsphere (38.6% ± 0.3%) was slightly higher than that for the ones produced in the present investigation (27.57% ± 2.87%). However, the method presented in this study has greater reliability and is easier to perform for the preparation of ENR-MICs. A previous study showed that microspheres with a diameter of 5 µm or less would be mainly captured by reticuloendothelial cells in the liver whereas those with a diameter ≥ 7 µm would be filtered by capillary beds in the lung [12]. The ENR-MICs prepared in the present investigation had a mean diameter of 10.06 µm and 89.93% of all the microspheres had a diameter ranging from 7.0 µm to 30.0 µm, indicating that these microspheres could easily target the lung.
In the present study, the in vitro release rate of ENR from the microspheres was markedly slowed compared to the ENR raw material. The models of zero- and first-order kinetics, Higuchi, Weibull, Baker-Lonsdale, Hixon-Crowell, and Riger-Peppas were used to fit the release profiles of ENR from the microspheres. Ultimately, the zero-order kinetic equation had the best fit. The in vitro release data showed that the PLGA loaded into the microspheres was able to control ENR release.
Tissue distribution of the ENR-MICs was assessed in 156 rats to determine if there the microspheres could reduce the ENR in vivo release rate and easily target the lung. The pharmacokinetic parameters of the ENR-MICs and ENR-INJ were calculated based on the concentration versus time data. For the ENR-MICs, the longest t1/2β of 13.28 h was found in lung followed by liver (5.67 h), kidney (4.81 h), muscle (2.03 h), and plasma (0.99 h). For the conventional injection, the longest t1/2β (27.42 h) was found in kidney followed by liver (9.95 h), lung (7.94 h), muscle (2.28 h), and plasma (1.60 h). The pharmacokinetic variables for ENR after one single intravenous administration have been compared in five different animals including rats and ranged from 1.48 h in mice to 2.82 h in cows [3]. When various single doses of ENR which ranged from 5 mg/kg to 400 mg/kg were subcutaneously administered to rats, a markedly disproportionate increase of t1/2β was observed with the increase of the doses, and nonlinear kinetics of elimination were observed [30].
Three targeting parameters were further evaluated in the present study based on the AUC0-∞ and Cmax. Compared to the conventional preparation, AUC0-∞ and Cmax in lung for the ENR-MICs were both markedly increased. For the ENR-MICs, both Re and Ce in lung were the greatest among the values for all sampled tissues. The ENR-MICs prepared in the current investigation appeared to have potent lung-targeting abilities and reached a high concentration (93.36 µg/g) in lung. At this concentration, toxicity or inflammation may occur. However, no abnormal clinical findings were observed in rats that were subcutaneously administered a higher dose of enrofloxacin (400 mg/kg bw). Therefore, the possibility that the ENR-MICs may cause lung toxicity appears to be low. An additional study is being carried out in rats to determine whether the microspheres indeed cause lung toxicity and the results will be available in the future.
In summary, rats were used as model species in the current investigation to evaluate the lung-targeting abilities of ENR-MICs. Ultimately, these microspheres will be used for treating other types of animals such as dogs and cats. Therefore, further studies are needed to evaluate the pharmacokinetics, safety, and lung-targeting abilities of the ENR-MICs in the target animals.
Figures and Tables
Fig. 1
(A) Optical photomicrograph of the enrofloxacin microspheres (ENR-MICs). (B) Scanning electron microscopy micrograph of the ENR raw material. (C) Scanning electron microscopy micrograph of an ENR-MIC. Scale bar = 50 µm. Magnification: 100× (A), 150× (B), 3000× (C).
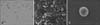
Fig. 2
Particle size distribution of the ENR-MICs. Size distribution was determined using 800 particles chosen randomly.
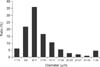
Fig. 4
Semilogarithmic plots showing the mean ± SD plasma and tissue concentrations of ENR vs. time in rats (n = 6) after intravenous administration of the ENR-MICs (7.5 mg/kg bw).
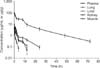
Fig. 5
Semilogarithmic plots showing the mean ± SD plasma and tissue concentrations of ENR vs. time in rats (n = 6) after intravenous administration of the ENR-INJ (7.5 mg/kg bw).
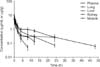
Table 1
The concentrations (µg/mL or µg/g) of ciprofloxacin in plasma and tissues after one single intravenous injection of ENR-MICs (7.5 mg/kg bw) in rats (n = 6, mean ± SD)
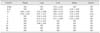
Table 2
The pharmacokinetic parameters of ENR in plasma and tissues after one single intravenous injection of the microspheres (7.5 mg/kg bw) in rats (mean of six rats)

Table 3
The concentrations (µg/mL or µg/g) of ciprofloxacin in plasma and tissues after one single intravenous injection of a conventional ENR-injection ([ENR-INJ], 7.5 mg/kg bw) in rats (n = 6, mean ± SD)
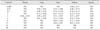
Acknowledgments
This study was supported by the Doctoral Scientific Research Foundation of Henan University of Science and Technology, China (grant no. 09001677).
References
1. Bebear CM, Renaudin J, Charron A, Renaudin H, de Barbeyrac B, Schaeverbeke T, Bebear C. Mutations in the gyrA, parC, and parE genes associated with fluoroquinolone resistance in clinical isolates of Mycoplasma hominis. Antimicrob Agents Chemother. 1999; 43:954–956.


2. Bimazubute M, Cambier C, Baert K, Vanbelle S, Chiap P, Albert A, Delporte JP, Gustin P. Penetration of enrofloxacin into the nasal secretions and relationship between nasal secretions and plasma enrofloxacin concentrations after intramuscular administration in healthy pigs. J Vet Pharmacol Ther. 2010; 33:183–188.


3. Bregante MA, Saez P, Aramayona JJ, Fraile L, Garcia MA, Solans C. Comparative pharmacokinetics of enrofloxacin in mice, rats, rabbits, sheep, and cows. Am J Vet Res. 1999; 60:1111–1116.
5. Cambier C, Bimazubute M, Baert K, Vanbelle S, Chiap P, Albert A, Delporte JP, Gustin P. Penetration of enrofloxacin into the nasal secretions in healthy pigs. Relationship between nasal secretions and plasma enrofloxacin concentrations. J Vet Pharmacol Ther. 2009; 32:Suppl 1. 147–148.
6. Dorfman M, Barsanti J, Budsberg SC. Enrofloxacin concentrations in dogs with normal prostate and dogs with chronic bacterial prostatitis. Am J Vet Res. 1995; 56:386–390.
7. El-Sherbiny IM, Smyth HDC. Biodegradable nano-micro carrier systems for sustained pulmonary drug delivery: (I) self-assembled nanoparticles encapsulated in respirable/swellable semi-IPN microspheres. Int J Pharm. 2010; 395:132–141.


8. Gautier-Bouchardon AV, Reinhardt AK, Kobisch M, Kempf I. In vitro development of resistance to enrofloxacin, erythromycin, tylosin, tiamulin and oxytetracycline in Mycoplasma gallisepticum, Mycoplasma iowae and Mycoplasma synoviae. Vet Microbiol. 2002; 88:47–58.


9. Genchi M, Pengo G, Genchi C. Efficacy of moxidectin microsphere sustained release formulation for the prevention of subcutaneous filarial (Dirofilaria repens) infection in dogs. Vet Parasitol. 2010; 170:167–169.


10. Gupta PK, Hung CT. Quantitative evaluation of targeted drug delivery systems. Int J Pharm. 1989; 56:217–226.


11. Haritova A, Urumova V, Lutckanov M, Petrov V, Lashev L. Pharmacokinetic-pharmacodynamic indices of enrofloxacin in Escherichia coli O78/H12 infected chickens. Food Chem Toxicol. 2011; 49:1530–1536.


12. Ilium L, Davis SS, Wilson CG, Thomas NW, Frier M, Hardy JG. Blood clearance and organ deposition of intravenously administered colloidal particles. The effects of particle size, nature and shape. Int J Pharm. 1982; 12:135–146.


13. Kalpana S, Aggarwal M, Srinivasa Rao G, Malik JK. Effects of aflatoxin B1 on tissue residues of enrofloxacin and its metabolite ciprofloxacin in broiler chickens. Environ Toxicol Pharmacol. 2012; 33:121–126.


14. Keil DJ, Fenwick B. Role of Bordetella bronchiseptica in infectious tracheobronchitis in dogs. J Am Vet Med Assoc. 1998; 212:200–207.
15. Lagarce F, Faisant N, Desfontis JC, Marescaux L, Gautier F, Richard J, Menei P, Benoit JP. Baclofen-loaded microspheres in gel suspensions for intrathecal drug delivery: in vitro and in vivo evaluation. Eur J Pharm Biopharm. 2005; 61:171–180.


16. Lam XM, Duenas ET, Daugherty AL, Levin N, Cleland JL. Sustained release of recombinant human insulin-like growth factor-I for treatment of diabetes. J Control Release. 2000; 67:281–292.


17. Lizondo M, Pons M, Gallardo M, Estelrich J. Physicochemical properties of enrofloxacin. J Pharm Biomed Anal. 1997; 15:1845–1849.


18. Mu L, Feng SS. A novel controlled release formulation for the anticancer drug paclitaxel (Taxol®): PLGA nanoparticles containing vitamin E TPGS. J Control Release. 2003; 86:33–48.


19. Nouaille-Degorce B, Veau C, Dautrey S, Tod M, Laouari D, Carbon C, Farinotti R. Influence of renal failure on ciprofloxacin pharmacokinetics in rats. Antimicrob Agents Chemother. 1998; 42:289–292.


20. Rahal A, Kumar A, Ahmad AH, Malik JK, Ahuja V. Pharmacokinetics of enrofloxacin in sheep following intravenous and subcutaneous administration. J Vet Pharmacol Ther. 2006; 29:321–324.


21. Sahin S, Selek H, Ponchel G, Ercan MT, Sargon M, Hincal AA, Kas HS. Preparation, characterization and in vivo distribution of terbutaline sulfate loaded albumin microspheres. J Control Release. 2002; 82:345–358.


22. Schettini DA, Ribeiro RR, Demicheli C, Rocha OGF, Melo MN, Michalick MSM, Frézard F. Improved targeting of antimony to the bone marrow of dogs using liposomes of reduced size. Int J Pharm. 2006; 315:140–147.


23. Schmidt S, Müller RH. Plasma protein adsorption patterns on surfaces of Amphotericin B-containing fat emulsions. Int J Pharm. 2003; 254:3–5.


24. Schroder J. Enrofloxacin: a new antimicrobial agent. J S Afr Vet Assoc. 1989; 60:122–124.
25. Shive MS, Anderson JM. Biodegradation and biocompatibility of PLA and PLGA microspheres. Adv Drug Deliv Rev. 1997; 28:5–24.


26. Son JS, Appleford M, Ong JL, Wenke JC, Kim JM, Choi SH, Oh DS. Porous hydroxyapatite scaffold with three-dimensional localized drug delivery system using biodegradable microspheres. J Control Release. 2011; 153:133–140.


27. Tang S, Zhou Y, Li R, Chen Q, Xiao X. Pharmacokinetics and lung-targeting characterization of a newly formulated enrofloxacin preparation. J Vet Pharmacol Ther. 2007; 30:443–450.


28. Wu CC, Shryock TR, Lin TL, Faderan M, Veenhuizen MF. Antimicrobial susceptibility of Mycoplasma hyorhinis. Vet Microbiol. 2000; 76:25–30.
29. Ye J, Wang Q, Zhou X, Zhang N. Injectable actarit-loaded solid lipid nanoparticles as passive targeting therapeutic agents for rheumatoid arthritis. Int J Pharm. 2008; 352:273–279.


30. Yi H, Shin MJ, Cho SM, Lee DG, Cho K, Cho HJ, Shin SJ, Bartlett MG, Kim JS, Shin HC. Nonlinear toxicokinetics of enrofloxacin in rats. Arch Pharm Res. 2010; 33:1851–1857.


31. Zeng ZL, Huang XH, Ding HZ, Zhen ZL. A comparison of pharmacokinetics in pigs after intramuscular administration of two different pH enrofloxacin injections. Chinese J Vet Drug. 2001; 35:13–15.