Abstract
The purpose of this study was to determine whether the Ca2+ signaling pathway is involved in the ability of osteoprotegerin (OPG) to inhibit osteoclast differentiation and maturation. RAW264.7 cells were incubated with macrophage colony-stimulating factor (M-CSF) + receptor activator of nuclear factor-κB ligand (RANKL) to stimulate osteoclastogenesis and then treated with different concentrations of OPG, an inhibitor of osteoclast differentiation. The intracellular Ca2+ concentration [Ca2+]i and phosphorylation of Ca2+/calmodulin-dependent protein kinase II (CaMKII) in the different treatment groups were measured by flow cytometry and Western blotting, respectively. The results confirmed that M-CSF + RANKL significantly increased [Ca2+]i and CaMKII phosphorylation in osteoclasts (p < 0.01), and that these effects were subsequently decreased by OPG treatment. Exposure to specific inhibitors of the Ca2+ signaling pathway revealed that these changes varied between the different OPG treatment groups. Findings from the present study indicated that the Ca2+ signaling pathway is involved in both the regulation of osteoclastogenesis as well as inhibition of osteoclast differentiation and activation by OPG.
Bone resorption and formation are the two main physiological processes of bone remodeling [5]. Osteoclasts are multinucleated cells that control these processes via bone resorption [1024]. Bone volume is decreased as a result of elevated osteoclast activity, and bone volume is increased by suppressing osteoclast activity [3]. Abnormal osteoclast activity can lead to metabolic bone disorders such as osteoporosis, periodontal disease, and rheumatoid arthritis [3].
Osteoprotegerin (OPG) is a member of the tumor necrosis factor (TNF) receptor superfamily that negatively mediates differentiation and activation of osteoclasts [20]. OPG, receptor activator of nuclear factor-κB (RANK), and RANK ligand (RANKL) form the OPG/RANKL/RANK axis. These factors play an essential role in the regulation of differentiation, activation, survival, and apoptosis of osteoclasts [28].
The Ca2+ signaling pathway is also important for osteoclast differentiation and function [25] via variations in intracellular Ca2+ concentration ([Ca2+]i) [9]. Ca2+ oscillation during osteoclastogenesis is induced by RANKL, resulting in calcineurin-mediated activation of nuclear factor of activated T cells c1 (NFATc1) and promotion of osteoclast differentiation [13]. Following the binding of RANKL to RANK, Ca2+ oscillation is triggered by activation of co-stimulatory receptors that in turn activate phosphatase C-γ (PLCγ) [14]. Activation of PLCγ promotes the generation of inositol 1,4,5-triphosphate (IP3), leading to the subsequent release of Ca2+ from the endoplasmic reticulum (ER) [9].
Ca2+ mediates cellular behavior via Ca2+/calmodulin-dependent protein kinase II (CaMKII) [21]. This ubiquitously expressed effector protein transduces elevated Ca2+ signals in cells to multiple target proteins including ion channels and transcriptional activators. Previous reports have demonstrated that CaMKII is a modifier in the signaling pathway that regulates Ca2+ oscillation, that the CaMKII/RANK signaling pathway is involved in the regulation of early osteoclast differentiation [25], and that the formation of osteoclasts is inhibited by CaMKII antagonists, indicating that CaMKII participates in osteoclastogenesis via activator protein 1 (AP-1) transcription factor family members [18].
Our previous study demonstrated that macrophage colonystimulating factor (M-CSF) and RANKL can induce the differentiation of RAW 264.7 cells into osteoclasts [7]. This process is inhibited by OPG. Despite considerable research into the involvement of Ca2+ signaling in multiple physiological processes [2], its effects on osteoclasts remain unclear and were the focus of this investigation.
RAW 264.7 murine monocyte/macrophage cells were obtained from the American Type Culture Collection (ATCC, USA). The cells were cultured in α-minimal essential medium (α-MEM; Invitrogen, USA) containing 10% fetal bovine serum (FBS), 2 mM/L L-glutamine, 100 U/mL penicillin, and 100 µg/mL streptomycin at 37℃ in a humidified atmosphere of 5% CO2. After culturing, the cells were seeded in 6-well plates (1.5 × 104 cells/mL) and incubated for 24 h. The medium was replaced with serum-free α-MEM plus 25 ng/mL M-CSF and 30 ng/mL RANKL (PeproTech, USA), and the cells were cultivated for an additional 48 h. For the OPG treatment groups, 0, 10, 20, 50, or 100 ng/mL OPG (PeproTech) were added to the cultured cells treated with M-CSF + RANKL, and the cells were cultured for another 30 min.
Following culture, the medium was replaced with serum-free α-MEM and the cells were pretreated with OPG (100 ng/mL). The cells were then divided into two groups and treated as follows. For the first group, the cells were incubated for 30 min with 50 µM 2-APB (Sigma-Aldrich, USA), a specific IP3R inhibitor. M-CSF + RANKL were added to the medium as described above, and the cells were cultured for another 30 min. For the second group, the cells were incubated for 30 min with 10 µM KN93 (Sigma-Aldrich), a specific inhibitor of CaMKII. M-CSF + RANKL were then added to the medium and the cells were cultured for an additional 30 min.
The treated cells were transferred to microcentrifuge tubes and 400 µL Fluo-4-AM (Sigma-Aldrich) were added at a final concentration of 5 µM/mL. The cells were incubated in the dark for 30 min at 37℃ and then rinsed twice with PBS. The mean fluorescent intensity from 1 × 104 randomly selected cells for each sample was measured by flow cytometry at an emission wavelength of 516 nm with alternate excitation wavelengths of 494 nm. Each experiment was performed in triplicate for each treatment group.
Cells from each treatment group were collected and lysed in 180 µL radioimmunoprecipitation assay (RIPA) buffer with 1% (v/v) PMSF for 30 min with intermittent shaking. After sonication, the lysed cells were centrifuged (12,000 × g for 10 min at 4℃) and the supernatants were collected. Total protein concentration in the supernatants was determined for each sample and equal amounts were separated on 12% SDS-polyacrylamide gels at 120 V for 90 min. The separated proteins were transferred by electroblotting to nitrocellulose membranes (Millipore, USA). The membranes were blocked in Tri-sbuffered saline with Tween-20 (TBST) containing 5% nonfat milk for 90 min at room temperature. Next, the membranes were washed five times for 5 min each with TBST and then incubated overnight at 4℃ with primary anti-phosphorylated (p)-CaMKII antibody or anti-CaMKII antibody (Cell Signaling Technology, USA) diluted in 5% BSA-TBST. The membranes were subsequently washed five times for 5 min each with TBST and incubated at room temperature for 90 min with rabbit anti-sheep IgG HRP secondary antibody (Santa Cruz Biotechnology, USA) diluted in 5% BSA-TBST. After washing five times for 5 min each with TBST, the immunoreactive proteins were visualized by electrochemiluminescence using ECL-plus detection reagents. Intensity of the protein bands was measured using Gel-Pro analyzer software (ver. 4.0; Media Cybernetics, USA).
[Ca2+]i was significantly higher in osteoclasts derived from RAW264.7 cells treated with M-CSF + RANKL compared to non-induced RAW264.7 cells (p < 0.01). Treatment with 50 or 100 ng/mL OPG significantly reduced [Ca2+]i in osteoclasts compared to untreated control cells (p < 0.05 and p < 0.01, respectively). However, no significant difference in [Ca2+]i was found when comparing the 10 and 20 ng/mL OPG treatment groups to the control group (Fig. 1).
Elevated [Ca2+]i observed in osteoclasts produced from M-CSF + RANKL-induced RAW264.7 cells was significantly reduced by exposure to 2-APB, an inhibitor of the Ca2+ signaling pathway, compared to osteoclasts derived from non-induced RAW24.7 cells. In addition, exposure to 2-APB further decreased [Ca2+]i in the OPG-treatment groups compared to the control cells (p < 0.01; Fig. 2).
The level of p-CaMKII was significantly higher in osteoclasts differentiated from M-CSF + RANKL-treated RAW264.7 cells compared to osteoclasts produced by non-induced RAW264.7 cells (p < 0.01). However, the levels of p-CaMKII were significantly lower in osteoclasts treated with 50 and 100 ng/mL OPG compared to the untreated control group (p < 0.01; panel B in Fig. 3). These findings indicated that OPG downregulated CaMKII phosphorylation in osteoclasts in a dose-dependent manner. Phosphorylation of CaMKII that was enhanced in osteoclasts differentiated from M-CSF + RANKL-treated RAW264.7 cells relative to those from non-induced RAW24.7 cells was significantly suppressed in the presence of KN93, an inhibitor of the Ca2+ signaling pathway. Furthermore, CaMKII phosphorylation in the OPG treatment groups was further reduced in the presence of KN93 compared to the level observed in the untreated control group (p < 0.01; panel B in Fig. 4).
We previously reported that two key cytokines, M-CSF and RANKL, are involved in the induction of osteoclast differentiation [7]. The induced osteoclasts were characterized by tartrate resistant acid phosphatase (TRAP)-positivity, regular and intact F-actin rings, and vigorous resorption activity. We further demonstrated that OPG inhibits the differentiation of osteoclast precursors, reduces the resorption capacity of mature osteoclasts, and destroys the cytoskeletal structure in osteoclasts [7]. The aim of the present study was to determine whether the Ca2+ signaling pathway promotes OPG inhibition of osteoclast differentiation and activation.
Ca2+ enters the cytoplasm from the extracellular environment and intracellular calcium stores following activation of the Ca2+ signaling pathway [26]. [Ca2+]i has been shown to be increased in many types of cells during apoptosis, particularly during the early phase [1611]. [Ca2+]i is one of the key factors that influences osteoclastic activity [1519] and is elevated following RANKL/RANK binding [9]. This is consistent with the present observation that osteoclastic activity induced by M-CSF + RANKL increases [Ca2+]i. Activation of the RANKL/RANK signaling pathway was shown to induce Ca2+ oscillation in osteoclast precursors [23]. Ca2+ oscillation is closely associated with differentiation, activation, and apoptosis in osteoclasts [18]. This process can be initiated by a variety of biomolecular and mechanical stimuli including high [Ca2+]i, ATP, integrin, and RANKL. In addition, various receptors and proteins are involved in the release of Ca2+ from calcium stores in the ER [9]; this process plays an important role in maintaining Ca2+ oscillation [9]. Furthermore, store-operated calcium entry (SOCE) was found to mediate the downstream cascade which were related to differentiation and activation of osteoclasts [5]. It has been suggested that the store-operated calcium (SOC) channel could be sensitized by RANKL [9]. This was demonstrated by showing that non-specific blockers of the SOC channel could attenuate bone resorption activity via suppression of SOCE [15]. In addition, RANKL inhibits Ca2+ oscillation [13].
The CaMK protein kinase family includes CaMKI, CaMKIV, and CaMKII [1722]. These kinases are involved in numerous cellular physiological processes such as cell cycle regulation, ion channels, and release of neurotransmitters [1]. They are also critical regulatory factors for downstream events in the Ca2+ signaling pathway. Chang et al. [4] suggested that the c-Jun N-terminal kinases-mitogen-activated protein kinases (JNK/MAPK) signaling pathway helps regulate osteoclast formation. They found that blocking the JNK signaling pathway during osteoclast differentiation reduces the number of TRAP-positive cells. This was attributed to decreased expression of NFATc1 due to reduced CaMK levels. Furthermore, it was shown that NFATc1 expression could be suppressed by the Ca2+ pathway inhibitor KN93, indicating that CaMK catalyzes NFATc1 expression during the differentiation of osteoclast precursors. By demonstrating that KN93 reduces the number of TRAP-positive cells, it was concluded that JNK/MAPK mediates CaMK activity, thereby impacting NFATc1 expression during osteoclast differentiation.
The extracellular regulated protein kinases (ERK)/MAPK signaling pathway has also been shown to be involved in the regulation of osteoclast differentiation and survival [6]. Furthermore, the transcriptional activity of cyclic adenosine monophosphate (cAMP), a downstream target of CaMK, and ERK phosphorylation were both increased during RANKLinduced differentiation in osteoclasts [1]. In contrast, the Ca2+ pathway inhibitors KN93 and KN62 were found to delay the formation of osteoclasts and decrease cathepsin K expression in a time- and dose-dependent manner. The phosphorylation of ERK during RANKL-induced differentiation of osteoclasts is also inhibited by KN93 and KN62 [1].
AP-1 is comprised of dimers formed by Jun and Fos family proteins, which are both important in the formation of osteoclasts [12]. AP-1 has been implicated in CaMKII regulation of osteoclast differentiation [18], implying that CaMKII plays significant a role in Ca2+ signaling during osteoclast differentiation. This was consistent with data from our previous study demonstrating that CaMKII is activated by increased [Ca2+]i via sensitization of calmodulin in the RANKL/RANK signaling pathway [18].
In agreement with previous reports [1827], we found that [Ca2+]i and the phosphorylation levels of CaMKII were increased in M-CSF + RANKL-treated osteoclasts differentiated from RAW264.7 cells. We further demonstrated that these were decreased by treatment with OPG during osteoclastogenesis, indicating that OPG inhibited osteoclast differentiation. Our findings were verified by observing changes in [Ca2+]i and CaMKII phosphorylation following exposure to specific inhibitors of the Ca2+ signaling pathway.
In summary, findings from this study along with data from the literature revealed that [Ca2+]i varies during osteoclast differentiation, and that CaMKII plays a key role in this process. However, further investigations should be conducted to determine how variations in [Ca2+]i differ during differentiation and apoptosis in osteoclasts. Our results also suggested that the Ca2+ signaling pathway helps regulate osteoclast differentiation and activation along with the inhibition of osteoclastogenesis by OPG.
Figures and Tables
Fig. 1
Effect of osteoprotegerin (OPG) on [Ca2+]i in macrophage colony-stimulating factor (M-CSF) + receptor activator of nuclear factor-κB ligand (RANKL)-treated osteoclasts. [Ca2+]i in the osteoclasts following treatment with 0, 10, 20, 50, and 100 ng/mL OPG was analyzed by flow cytometry. Fluorescent intensities showed that OPG reduced [Ca2+]i in the osteoclasts. Results are expressed as the mean ± SEM for three independent experiments. *p < 0.05 and **p < 0.01 vs. the control group (#M-CSF + RANKL, 0 ng/mL OPG).
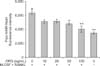
Fig. 2
Effect of 2-APB on [Ca2+]i in osteoclasts. [Ca2+]i in osteoclasts derived from RAW264.7 cells was analyzed by flow cytometry for the different treatment groups as indicated. Fluorescent intensities represent [Ca2+]i. It was found that 2-APB (50 ng/mL) reduces [Ca2+]i in M-CSF + RANKL-induced osteoclasts. [Ca2+]i was further lowered following treatment with 100 ng/mL OPG. Results are expressed as the mean ± SEM of three independent experiments. *p < 0.05 and **p < 0.01 vs. the control group (#M-CSF + RANKL, 0 ng/mL OPG, and 0 ng/mL 2-APB).
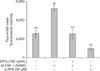
Fig. 3
Effect of OPG on Ca2+/calmodulin-dependent protein kinase II (CaMKII) phosphorylation in M-CSF + RANKL-induced osteoclasts. The phosphorylation levels of CaMKII in the osteoclasts following treatment with 0, 10, 20, 50, and 100 ng/mL OPG were determined by Western blotting. Band intensities represented the level of phosphorylated (p)-CaMKII relative to CaMKII. These finding showed that OPG reduces the phosphorylation of CaMKII in a dose-dependent manner. Results are expressed as the mean ± SEM for three independent experiments. *p < 0.05 and **p < 0.01 vs. the control group (M-CSF + RANKL, 0 ng/mL OPG).
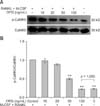
Fig. 4
Effect of KN93 on CaMKII phosphorylation in osteoclasts. The levels of p-CaMKII in osteoclasts differentiated from RAW264.7 cells were measured by Western blotting. Band intensities represent the levels of p-CaMKII relative to CaMKII in the different treatment groups as indicated. These findings showed that KN93 (10 ng/mL) reduces the levels of p-CaMKII in M-CSF + RANKL-induced osteoclasts. The levels of p-CaMKII were further decreased following treatment with OPG (100 ng/mL). Results are expressed as the mean ± SEM of three independent experiments. *p < 0.05 and **p < 0.01 vs. the control groups (con: M-CSF + RANKL, 0 ng/mL OPG, and 0 µM KN93).
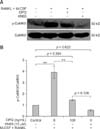
Acknowledgments
This study was supported by the National Natural Science Foundation of China (grant no. 31172373, 31372495, 31302154), the Priority Academic Program Development of Jiangsu Higher Education Institutions, and the National Research Foundation for the Doctoral Program of Higher Education of China (20113250110003).
References
1. Ang ES, Zhang P, Steer JH, Tan JW, Yip K, Zheng MH, Joyce DA, Xu J. Calcium/calmodulin-dependent kinase activity is required for efficient induction of osteoclast differentiation and bone resorption by receptor activator of nuclear factor kappa B ligand (RANKL). J Cell Physiol. 2007; 212:787–795.


2. Blair HC, Robinson LJ, Zaidi M. Osteoclast signalling pathways. Biochem Biophys Res Commun. 2005; 328:728–738.


3. Boyle WJ, Simonet WS, Lacey DL. Osteoclast differentiation and activation. Nature. 2003; 423:337–342.


4. Chang EJ, Ha J, Huang H, Kim HJ, Woo JH, Lee Y, Lee ZH, Kim JH, Kim HH. The JNK-dependent CaMK pathway restrains the reversion of committed cells during osteoclast differentiation. J Cell Sci. 2008; 121:2555–2564.


5. Di Capite J, Ng SW, Parekh AB. Decoding of cytoplasmic Ca2+ oscillations through the spatial signature drives gene expression. Curr Biol. 2009; 19:853–858.


6. Feng X. Regulatory roles and molecular signaling of TNF family members in osteoclasts. Gene. 2005; 350:1–13.


7. Fu YX, Gu JH, Zhang YR, Tong XS, Zhao HY, Yuan Y, Liu XZ, Bian JC, Liu ZP. Osteoprotegerin influences the bone resorption activity of osteoclasts. Int J Mol Med. 2013; 31:1411–1417.


8. Hofbauer LC, Kühne CA, Viereck V. The OPG/RANKL/RANK system in metabolic bone diseases. J Musculoskelet Neuronal Interact. 2004; 4:268–275.
9. Hwang SY, Putney JW Jr. Calcium signaling in osteoclasts. Biochim Biophys Acta. 2011; 1813:979–983.


10. Jansen ID, Vermeer JA, Bloemen V, Stap J, Everts V. Osteoclast fusion and fission. Calcif Tissue Int. 2012; 90:515–522.


11. Joseph SK, Hajnóczky G. IP3 receptors in cell survival and apoptosis: Ca2+ release and beyond. Apoptosis. 2007; 12:951–968.


13. Kim MS, Yang YM, Son A, Tian YS, Lee SI, Kang SW, Muallem S, Shin DM. RANKL-mediated reactive oxygen species pathway that induces long lasting Ca2+ oscillations essential for osteoclastogenesis. J Biol Chem. 2010; 285:6913–6921.


14. Koga T, Inui M, Inoue K, Kim S, Suematsu A, Kobayashi E, Iwata T, Ohnishi H, Matozaki T, Kodama T, Taniguchi T, Takayanagi H, Takai T. Costimulatory signals mediated by the ITAM motif cooperate with RANKL for bone homeostasis. Nature. 2004; 428:758–763.


15. Mentaverri R, Kamel S, Brazier M. Involvement of capacitive calcium entry and calcium store refilling in osteoclastic survival and bone resorption process. Cell calcium. 2003; 34:169–175.


16. Orrenius S, Zhivotovsky B, Nicotera P. Regulation of cell death: the calcium-apoptosis link. Nat Rev Mol Cell Bio. 2003; 4:552–565.


17. Penzes P, Cahill ME, Jones KA, Srivastava DP. Convergent CaMK and RacGEF signals control dendritic structure and function. Trends Cell Biol. 2008; 18:405–413.


18. Seales EC, Micoli KJ, McDonald JM. Calmodulin is a critical regulator of osteoclastic differentiation, function, and survival. J Cell Biochem. 2006; 97:45–55.


19. Silver IA, Murrills RJ, Etherington DJ. Microelectrode studies on the acid microenvironment beneath adherent macrophages and osteoclasts. Exp Cell Res. 1988; 175:266–276.


20. Simonet WS, Lacey DL, Dunstan CR, Kelley M, Chang MS, Lüthy R, Nguyen HQ, Wooden S, Bennett L, Boone T, Shimamoto G, DeRose M, Elliott R, Colombero A, Tan HL, Trail G, Sullivan J, Davy E, Bucay N, Renshaw-Gegg L, Hughes TM, Hill D, Pattison W, Campbell P, Sander S, Van G, Tarpley J, Derby P, Lee R, Boyle WJ. Osteoprotegerin: a novel secreted protein involved in the regulation of bone density. Cell. 1997; 89:309–319.


21. Soderling TR, Chang B, Brickey D. Cellular signaling through multifunctional Ca2+/calmodulin-dependent protein kinase II. J Biol Chem. 2001; 276:3719–3722.


22. Soderling TR, Stull JT. Structure and regulation of calcium/calmodulin-dependent protein kinases. Chem Rev. 2001; 101:2341–2352.


23. Takayanagi H, Kim S, Koga T, Nishina H, Isshiki M, Yoshida H, Saiura A, Isobe M, Yokochi T, Inoue J, Wagner EF, Mak TW, Kodama T, Taniguchi T. Induction and activation of the transcription factor NFATc1 (NFAT2) integrate RANKL signaling in terminal differentiation of osteoclasts. Dev Cell. 2002; 3:889–901.


24. Teitelbaum SL. Bone remodeling and the osteoclast. J Bone Miner Res. 1993; 8:Suppl 2. S523–S525.


25. Yao CH, Zhang P, Zhang L. Differential protein and mRNA expression of CaMKs during osteoclastogenesis and its functional implications. Biochem Cell Biol. 2012; 90:532–539.

