Abstract
Melatonin affects diverse physiological functions through its receptor and plays an important role in the central nervous system. In the present study, we compared immunoreactivity patterns of arylalkylamine N-acetyltransferase (AANAT), an enzyme essential for melatonin synthesis, and melatonin receptor type 1B (MT2) in the spinal cord of young adult (2~3 years) and aged (10~12 years) beagle dogs using immunohistochemistry and Western blotting. AANAT-specific immunoreactivity was observed in the nuclei of spinal neurons, and was significantly increased in aged dog spinal neurons compared to young adult spinal neurons. MT2-specific immunoreactivity was found in the cytoplasm of spinal neurons, and was predominantly increased in the margin of the neuron cytoplasm in aged spinal cord compared to that in the young adult dogs. These increased levels of AANAT and MT2 immunoreactivity in aged spinal cord might be a feature of normal aging and associated with a feedback mechanism that compensates for decreased production of melatonin during aging.
Melatonin is an indolamine derived from tryptophan. Biosynthesis of this factor is mediated by the sequential reaction of two key enzymes: arylalkylamine N-acetyltransferase (AANAT) and hydroxyindole-O-methyltransferase (HIOMT) [8]. AANAT catalyzes the transfer of an acetyl group from acetyl-CoA to the primary amine of serotonin that leads to the production of N-acetylserotonin (NAS) [11,31]. Many studies have demonstrated that melatonin has chronobiotic, antioxidant, anti-inflammatory, neuroprotective, and analgesic properties [3,4,6,7,26]. Melatonin exerts theses physiological functions via its G protein-coupled receptors: MT1 and MT2 [5].
AANAT and MT2 mRNA expression has been found in the central nervous system including the hippocampus, cerebellum, and spinal cord [23,30]. The effects of melatonin on the brain have been well established. However, the possibility of direct melatonin action in the spinal cord has not been extensively investigated. Recently, studies have suggested that melatonin influences antinociceptive effects via MT2 in the spinal cord [28,30].
Melatonin secretion progressively declines during the normal aging process. A significant reduction of circulating melatonin has also been observed with neurodegenerative disorders such as Alzheimer's disease [10,12]. However, few studies monitoring AANAT and MT2 expression in the spinal cord, which is morphologically, neurochemically, and physiologically altered during normal aging, have been performed [19,27,29]. Therefore, we conducted the present study to compare patterns of AANAT and MT2 immunoreactivity in the spinal cord of young adult and aged beagle dogs, which is a good model of aging [2,20].
Clinically and neurologically normal male beagle dogs were obtained from the Experimental Animal Center, Kangwon National University (Korea). The animals were used when they were 2~3 years old (young adult dogs, n = 12, 9~11 kg body weight) or 10~12 years old (aged dogs, n = 12, 10~13 kg body weight). None of the dogs showed any clinical symptoms or other signs of neural disorders. The animals were housed in a conventional environment with adequate temperature (23℃) and humidity (60%) as well as a 12-h light/dark cycle with light onset at 07:00 am. They also had free access to water and food (DBL, Korea). To ensure adaptation to the new environment, the animals were kept in the departmental holding room for 1 week prior to the experiments. Procedures for handling and caring for the dogs were in compliance with current international laws and policies (National Institutes of Health [NIH] Guide for the Care and Use of Laboratory Animals, NIH Publication No. 85 23, 1985, revised 1996) [16].
Spinal cord tissue sections were prepared for histochemical analysis using a previously described method [1]. In brief, the young adult and aged dogs (n = 7 for each group) were anesthetized with a mixture of Zoletil 50 (8 mg/kg, Virbac Korea, Korea) and Xylazine (2 mg/kg, Bayer Korea, Korea), and perfused transcardially with 0.1 M phosphate-buffered saline (PBS, pH 7.4) followed by 4% paraformaldehyde in 0.1 M phosphate buffer (PB, pH 7.4). The cervical (C6-C8) and lumbar (L5-L6) spinal cord, levels that are closely related to limb innervation, were removed and postfixed in the 4% paraformaldehyde for 12 h at 4℃. The spinal cord tissues were cryoprotected by infusion with 30% sucrose overnight at 4℃. Next, the frozen tissues (-15℃~-20℃) were serially cut with a cryostat (Leica Biosystems, Germany) into sections 30 µm in thickness and transferred to six-well plates (SPL Lifescience, Korea) containing PBS.
Immunohistochemistry specific for AANAT and MT2 was performed under the same conditions for the dogs of different ages in order to determine whether the degree of staining was accurate. The sections were stained using a previously described method [1]. In brief, the sections were sequentially treated with 0.3% H2O2 and 10% normal goat serum (Vector Laboratories, USA) at room temperature. They were then incubated with rabbit anti-AANAT (diluted 1 : 500, Catalog No. ABIN751063; antibodies-online, USA) and goat anti-MEL-1B-R (T-18) (diluted 1 : 200, Catalog No. sc-13177; Santa Cruz Biotechnology, USA) overnight at 4℃. Next, the sections were subsequently exposed to biotinylated goat anti-rabbit IgG or rabbit anti-goat for 2 h and streptavidin peroxidase complex (1 : 200, Vector Laboratories) for 1 h at room temperature. Antibody binding was visualized by staining with 0.05% 3,3'-diaminobenzidine tetrahydrochloride (Sigma, USA) in 0.1 M Tris-HCl buffer (pH 7.2), and the sections were dehydrated by ethanol before being mounted on gelatin-coated slides (Marienfeld Superior, Germany) in Canada balsam (Kanto Chemical, Japan). In order to assess the immunostaining specificity, a negative control test was carried out with 10% pre-immune serum instead of primary antibody. No immunoreactivity was observed in any structure in the negative control.
To confirm the cellular location of AANAT immunoreactivity, sections of aged dog spinal cord were subjected to double immunofluorescence staining. Staining was performed using rabbit anti-AANAT (diluted 1 : 200, Catalog No. ABIN751063; antibodies-online, Germany) and mouse anti-NeuN (diluted 1 : 500, Catalog No. MAB377; EMD Millipore, USA) antibodies. The sections were incubated in the mixture overnight at room temperature. After washing three times for 10 min with PBS, the sections were then incubated in a mixture of Cy3-conjugated goat anti-rabbit IgG (1 : 200; Jackson ImmunoResearch Laboratories, USA) and fluorescein isothiocyanate (FITC)-conjugated goat anti-mouse IgG (1 : 200; Jackson ImmunoResearch Laboratories) for 2 h at room temperature. Immunoreactivity was observed with confocal microscopy (LSM 510 META NLO; Carl Zeiss, Germany).
To confirm changes in AANAT and MT2 expression in the lumbar spinal cord of young adult and aged dogs, Western blot analysis was performed using our previously described method [14]. In brief, the spinal cord tissues were homogenized (Sigma) in 50 mM PBS (pH 7.4, Sigma-Aldrich, USA) containing EGTA (pH 8.0, Santa Cruz Biotechnology), 0.2% NP-40 (Sigma), 10 mM EDTA (pH 8.0, Sigma), 15 mM sodium pyrophosphate (Santa Cruz Biotechnology), 100 mM β-glycerophosphate (Santa Cruz Biotechnology), 50 mM NaF (Santa Cruz Biotechnology), 150 mM NaCl (Santa Cruz Biotechnology), 2 mM sodium orthovanadate (Santa Cruz Biotechnology), 1 mM phenylmethylsulfonyl fluoride (PMSF, Santa Cruz Biotechnology), and 1 mM dithiothreitol (DTT, Santa Cruz Biotechnology). After centrifugation at 16,000 × g in a microcentrifuge for 5 min, the protein concentration in the supernatants was determined using a Micro BCA protein assay kit (Pierce Biotechnology, USA). Aliquots of the supernatant containing 20 µg of total protein were boiled in loading buffer containing 150 mM Tris (pH 6.8, Santa Cruz Biotechnology), 3 mM DTT (Santa Cruz Biotechnology), 6% SDS (Santa Cruz Biotechnology), 0.3% bromophenol blue (Santa Cruz Biotechnology), and 30% glycerol (Junsei Chemical, Japan). The samples (20 µg of total protein) were then loaded onto a polyacrylamide gel. After electrophoresis, the separated proteins were transferred to nitrocellulose membranes (Pall Corporation, USA). The membranes were incubated with 5% dry non-fat milk (Santa Cruz Biotechnology) in PBS containing 0.1% Tween 20 (Santa Cruz Biotechnology) followed by incubation with rabbit anti-AANAT (diluted 1 : 1,000) and goat anti-MEL-1B-R(T-18) (diluted 1 :1,000), peroxidase-conjugated donkey anti-rabbit IgG (Sigma), or anti-goat IgG (Sigma). Antibody binding was detected with an enhanced luminol-based chemiluminescent (ECL) kit (Thermo Scientific, USA).
For quantitative analysis of AANAT and MT2 immunoreactivity, 10 sections per animal were randomly selected from the corresponding areas of the cervical (C6-C8) and lumbar (L5-L6) spinal cord according to our previously described method [1]. The images were converted into an array of 512 × 512 pixels corresponding to a tissue area of 500 × 500 µm (20× primary magnification). Data are expressed as a percent relative to that of the adult dogs. Images of AANAT- and MT2-immunoreactive structures were obtained with an AxioM1 light microscope (Carl Zeiss) equipped with a digital camera (AxioCam; Carl Zeiss) connected to a PC monitor (Samsung, Korea). Each pixel resolution was 256 gray levels. The staining intensity of all AANAT- and MT2-immunoreactive structures was evaluated based on the relative optical density (ROD) obtained after transformation of the mean gray level using the formula: ROD = log (256/mean gray level). The ROD of the complete field was measured. Next, the brightness and contrast of each image file were calibrated using Adobe Photoshop (ver. 8.0; Microsoft, USA), and then analyzed using NIH Image 1.59 software (developed at the NIH, USA). Background staining values were obtained and subtracted from the immunoreactive intensities. The Western blot was densitometrically scanned (Samsung) to quantify the ROD of each band using Scion Image software (Informer Technologies, USA). A ratio of the ROD was calculated as a percentage with the adult group value designated as 100%.
AANAT-immunoreactive cells in the adult dogs were distributed in the gray matter of the cervical and lumbar spinal cord (Figs. 1A and D), and AANAT-specific signals were generally found in the nuclei of spinal neurons (Figs. 1a, b, e, and f). In the aged dogs, the AANAT expression pattern was similar to that found in the young adult dogs (Figs. 1B and E). However, AANAT immunoreactivity in the nuclei of the spinal neurons was significantly increased in the aged dog spinal cord compared to that in the young adult spinal cord (Figs. 1c, d, C, g, h, and F). Western blotting showed that the AANAT protein level in the spinal cord of the aged group was markedly increased compared to that found in the young adult group (Fig. 2).
We performed double immunofluorescence staining specific for AANAT and NeuN (a neuron marker) to determine whether AANAT immunoreactivity co-localized with NeuN-positive spinal neuron nuclei. AANAT-positive structures were co-localized with NeuN-positive spinal neuron nuclei in the aged spinal cord (Fig. 3).
In the young adult dogs, MT2-immunoreactive cells were easily observed throughout the gray matter of the cervical and lumbar spinal cord. Additionally, moderate levels of MT2 immunoreactivity were found in the cytoplasm of spinal neurons (Figs. 4a, b, e, and f). In the aged dogs, the expression pattern of MT2 was similar to that appearing in the young adult dogs (Figs. 4B and E). However, the level of MT2-specific immunoreactivity was much higher in the aged dog spinal cord than in the young adult spinal cord (Figs. 4c, d, C, g, h, and F). In addition, strong MT2 immunoreactivity was detected in the cytoplasm of spinal neurons in the aged group (Figs. 4d and h). Results of the Western blot study demonstrated that MT2 protein levels in the spinal cord of the aged group were markedly increased compared to that in the adult group (Fig. 2).
In the present study, we investigated age-related changes in the expression of AANAT and MT2 in the cervical and lumbar spinal cord. Levels of these factors are closely related with the innervations of limbs. Comparisons were made between young adult and aged dogs.
We found that AANAT-specific immunoreactivity generally appeared in nuclei of spinal neurons in the gray matter of the young adult spinal cord. This result concurs with data from a previous study showing that AANAT-immunoreactive structures can be observed in the nuclei of pinealocytes in humans and sheep [15]. In addition, it was reported that AANAT mRNA is mostly found in the gray matter of rat spinal cord [22,23]. It is thus likely that AANAT expression has a physiological function in spinal neurons.
The distribution pattern of MT2-immunoreactive cells in the spinal cord appears different among some species. It has been reported that melatonin receptors in rabbit and chicken spinal cord are localized in the dorsal horn and central gray matter [24,25]. Conversely, MT2 transcripts in the rat spinal cord are present in the dorsal and ventral horn [30]. Similar to rat spinal cord, MT2 immunoreactivity in the present study was observed in the gray matter of the cervical and lumbar spinal cord. The discrepancy may be species-related and reflect different functions of melatonin depending on the physiologically relevant location of the melatonin receptors.
It is well known that melatonin levels can change in a day and throughout the year [10,12,18]. This fluctuation in melatonin levels can be affected by a key enzyme, AANAT. In the present study, AANAT-specific immunoreactivity was significantly increased in the aged dog spinal cord compared to that in the young adult dogs. Our finding corresponds to data from a study by Sanchez-Hidalgo et al. [19] who reported that AANAT enzymatic activity was increased in the liver of aged rats although melatonin levels decrease during the aging process. In addition, age-related increases of AANAT mRNA levels and AANAT enzymatic activity have been found in the chicken pineal gland during postembryonic development [17].
In the current study, MT2-specific immunoreactivity was significantly increased in the aged dog spinal cord compared to that found in the young adult dogs. The level of immunoreactivity was increased in the neuronal cytoplasm. This result concurs with findings from a previous study showing that MT2 immunoreactivity and protein expression are markedly increased in the aged gerbil hippocampus [13]. Furthermore, it was discovered that plasma melatonin concentration are lowest although the affinity of melatonin for 2-[125I]-iodomelatonin and total 2-[125I]-iodomelatonin along with melatonin receptor mRNA expression are highest in the pars tuberalis of rat and in the pars tuberalis and suprachiasmatic nuclei of hamster [9,21].
In summary, we found that AANAT and MT2 immunoreactivity and protein levels increased in the nuclei of spinal neurons from aged dog spinal cord. These data suggest that increased AANAT and MT2 immunoreactivity in aged spinal cord neurons might be associated with a feedback mechanism that compensates for decreased melatonin production during the normal aging process. Based on the results of this study, a further study on the physiological function of melatonin in spinal neurons needs to find more specific mechanisms of aging-related changes in AANAT and MT2.
Figures and Tables
Fig. 1
Arylalkylamine N-acetyltransferase (AANAT)-specific immunoreactivity in the cervical (A and B) and lumbar (D and E) spinal cord of young adult (A and D) and aged (B and E) dogs. AANAT immunoreactivity in the nuclei of spinal neurons (arrows in panels c, d, g, and h) was apparently increased in the aged group. (C and F) The relative optical density (ROD) expressed as a percentage of AANAT immunoreactivity in the cervical (C) and lumbar (F) spinal cord of young adult and aged dogs (n = 7 per group; *p < 0.05 compared to the young adult dogs). Bars in the graphs indicate the mean values ± SEM. DH: dorsal horn, VH: ventral horn. Scale bars = 500 µm (A, B, D, and E) or 50 µm (a-h).
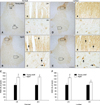
Fig. 2
Western blot analysis of AANAT and melatonin receptor type 1B (MT2) expression levels in lumbar spinal cord samples taken from young adult and aged dogs. The ROD of the immunoblot bands is expressed as percent values (n = 5 per group; *p < 0.05 vs. the young adult group). Data are presented as the mean ± SEM.
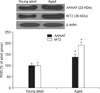
Fig. 3
Double immunofluorescence staining for AANAT (A) and NeuN (B) in the spinal cord of aged dogs. The merged images are also presented (C). AANAT immunoreactivity co-localized with NeuN-immunoreactive spinal neuron nuclei (arrows). Scale bar = 50 µm.
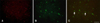
Fig. 4
MT2 expression in the cervical (A and B) and lumbar (D and E) spinal cord of young adult (A and D) and aged (B and E) dogs. MT2-specific immunoreactivity in the cytoplasm of spinal neurons (arrows in panels c, d, g, and h) was apparently increased in the aged group. Data are presented as the mean values ± SEM. (C and F) ROD expressed as the percentage of MT2 immunoreactivity in the cervical (C) and lumbar (F) spinal cord of young adult and aged dogs (n = 7 per group; *p < 0.05, significantly different from the young adult dogs). The bars indicate the mean values ± SEM. Scale bars = 500 µm (A B, D, and E) or 50 µm (a-h).
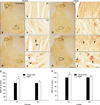
Acknowledgments
The authors would like to thank Seung Uk Lee for his technical help with this study. This research was supported by the Technology Innovation Program funded by the Ministry of Knowledge Economy (MKE; Korea), and by the National Research Foundation of Korea (NRF) funded by the Ministry of Education, Science and Technology (2010-0010580).
References
1. Ahn JH, Choi JH, Song JM, Lee CH, Yoo KY, Hwang IK, Kim JS, Shin HC, Won MH. Increase in Trx2/Prx3 redox system immunoreactivity in the spinal cord and hippocampus of aged dogs. Exp Gerontol. 2011; 46:946–952.


2. Cotman CW, Head E. The canine (dog) model of human aging and disease: dietary, environmental and immunotherapy approaches. J Alzheimers Dis. 2008; 15:685–707.


3. Dagci T, Yilmaz O, Taskiran D, Peker G. Neuroprotective agents: is effective on toxicity in glial cells? Cell Mol Neurobiol. 2007; 27:171–177.


4. Dominguez-Rodriguez A, Abreu-Gonzalez P, Sanchez-Sanchez JJ, Kaski JC. Melatonin and circadian biology in human cardiovascular disease. J Pineal Res. 2010; 49:14–22.


5. Dubocovich ML, Markowska M. Functional MT1 and MT2 melatonin receptors in mammals. Endocrine. 2005; 27:101–110.
6. Espino J, Pariente JA, Rodríguez AB. Oxidative stress and immunosenescence: therapeutic effects of melatonin. Oxid Med Cell Longev. 2012; 2012:670294.


7. Esposito E, Cuzzocrea S. Antiinflammatory activity of melatonin in central nervous system. Curr Neuropharmacol. 2010; 8:228–242.


8. Ganguly S, Coon SL, Klein DC. Control of melatonin synthesis in the mammalian pineal gland: the critical role of serotonin acetylation. Cell Tissue Res. 2002; 309:127–137.


9. Guerrero HY, Gauer F, Schuster C, Pévet P, Masson-Pévet M. Melatonin regulates the mRNA expression of the mt1 melatonin receptor in the rat Pars tuberalis. Neuroendocrinology. 2000; 71:163–169.


10. Hardeland R. Melatonin in aging and disease-multiple consequences of reduced secretion, options and limits of treatment. Aging Dis. 2012; 3:194–225.
11. Jagota A, Reddy MY. The effect of curcumin on ethanol induced changes in suprachiasmatic nucleus (SCN) and pineal. Cell Mol Neurobiol. 2007; 27:997–1006.


13. Lee CH, Choi JH, Yoo KY, Park OK, Hwang IK, You SG, Lee BY, Kang IJ, Won MH. MT2 melatonin receptor immunoreactivity in neurons is very high in the aged hippocampal formation in gerbils. Cell Mol Neurobiol. 2010; 30:255–263.


14. Lee DH, Ahn JH, Park JH, Yan BC, Cho JH, Kim IH, Lee JC, Jang SH, Lee MH, Hwang IK, Moon SM, Lee B, Cho JH, Shin HC, Kim JS, Won MH. Comparison of expression of inflammatory cytokines in the spinal cord between young adult and aged beagle dogs. Cell Mol Neurobiol. 2013; 33:615–624.


15. Maronde E, Saade A, Ackermann K, Goubran-Botros H, Pagan C, Bux R, Bourgeron T, Dehghani F, Stehle JH. Dynamics in enzymatic protein complexes offer a novel principle for the regulation of melatonin synthesis in the human pineal gland. J Pineal Res. 2011; 51:145–155.


16. National Research Council of the National Academies (US). Guide for the Care and Use of Laboratory Animals. 8th ed. Washington, D.C: National Academies Press;2011.
17. Piesiewicz A, Kedzierska U, Podobas E, Adamska I, Zuzewicz K, Majewski PM. Season-dependent postembryonic maturation of the diurnal rhythm of melatonin biosynthesis in the chicken pineal gland. Chronobiol Int. 2012; 29:1227–1238.


18. Reiter RJ. Pineal melatonin: cell biology of its synthesis and of its physiological interactions. Endocr Rev. 1991; 12:151–180.


19. Rodrigues de Amorim MA, Garcia-Segura LM, Goya RG, Portiansky EL. Decrease in PTEN and increase in Akt expression and neuron size in aged rat spinal cord. Exp Gerontol. 2010; 45:457–463.


20. Sarasa M, Pesini P. Natural non-trasgenic animal models for research in Alzheimer's disease. Curr Alzheimer Res. 2009; 6:171–178.


21. Schuster C, Gauer F, Malan A, Recio J, Pévet P, Masson-Pévet M. The circadian clock, light/dark cycle and melatonin are differentially involved in the expression of daily and photoperiodic variations in mt1 melatonin receptors in the Siberian and Syrian hamsters. Neuroendocrinology. 2001; 74:55–68.


22. Stefulj J, Hörtner M, Ghosh M, Schauenstein K, Rinner I, Wölfler A, Semmler J, Liebmann PM. Gene expression of the key enzymes of melatonin synthesis in extrapineal tissues of the rat. J Pineal Res. 2001; 30:243–247.


23. Uz T, Qu T, Sugaya K, Manev H. Neuronal expression of arylalkylamine N-acetyltransferase (AANAT) mRNA in the rat brain. Neurosci Res. 2002; 42:309–316.


24. Wan Q, Liao M, Brown GM, Pang SF. Localization and characterization of melatonin receptors in the rabbit spinal cord. Neurosci Lett. 1996; 204:77–80.


25. Wan Q, Pang SF. Segmental, coronal and subcellular distribution of 2-[125I]iodomelatonin binding sites in the chicken spinal cord. Neurosci Lett. 1994; 180:253–256.


26. Wilhelmsen M, Amirian I, Reiter RJ, Rosenberg J, Gögenur I. Analgesic effects of melatonin: a review of current evidence from experimental and clinical studies. J Pineal Res. 2011; 51:270–277.


27. Wu J, Hu Q, Huang D, Chen X, Chen J. Effect of electrical stimulation of sciatic nerve on synaptic plasticity of spinal dorsal horn and spinal c-fos expression in neonatal, juvenile and adult rats. Brain Res. 2012; 1448:11–19.


28. Yu CX, Zhu CB, Xu SF, Cao XD, Wu GC. Selective MT2 melatonin receptor antagonist blocks melatonin-induced antinociception in rats. Neurosci Lett. 2000; 282:161–164.


29. Yuan Q, Su H, Guo J, Tsang KY, Cheah KS, Chiu K, Yang J, Wong WM, So KF, Huang JD, Wu W, Lin ZX. Decreased c-Jun expression correlates with impaired spinal motoneuron regeneration in aged mice following sciatic nerve crush. Exp Gerontol. 2012; 47:329–336.

