Abstract
Betulinic acid (BA), a pentacyclic lupane-type triterpene, has a wide range of bioactivities. The main objective of this work was to evaluate the hepatoprotective activity of BA and the potential mechanism underlying the ability of this compound to prevent liver damage induced by alcohol in vivo. Mice were given oral doses of BA (0.25, 0.5, and 1.0 mg/kg) daily for 14 days, and induced liver injury by feeding 50% alcohol orally at the dosage of 10 ml/kg after 1 h last administration of BA. BA pretreatment significantly reduced the serum levels of alanine transaminase, aspartate transaminase, total cholesterol, and triacylglycerides in a dose-dependent manner in the mice administered alcohol. Hepatic levels of glutathione, superoxide dismutase, glutathione peroxidase, and catalase were remarkably increased, while malondialdehyde contents and microvesicular steatosis in the liver were decreased by BA in a dose-dependent manner after alcohol-induced liver injury. These findings suggest that the mechanism underlying the hepatoprotective effects of BA might be due to increased antioxidant capacity, mainly through improvement of the tissue redox system, maintenance of the antioxidant system, and decreased lipid peroxidation in the liver.
Alcohol abuse and its associated social consequences are a major health problem in many areas of the world. Alcohol abuse is a brain-centered addictive behavioral disorder that develops regardless of gender, race, age, or economic standing, and can lead to alcoholic liver disease (ALD) in many patients [5,13]. ALD presents a broad spectrum of disorders ranging from simple fatty liver to more severe forms of liver injury that include alcoholic hepatitis (AH), cirrhosis, and superimposed hepatocellular carcinoma [12]. In the United States, the Centers for Disease Control and Prevention estimate that 50% of people aged 18 or older drink alcohol [3]. Among these, 5% are classified as heavy drinkers and 15% are binge drink. The National Institute on Alcohol Abuse and Alcoholism (USA) has reported that liver cirrhosis is the 12th leading cause of death with a total of 29,925 deaths in the United States in 2007 (48% of which were alcohol-related) and is also a major contributor to liver disease-related mortality in other countries [12].
The abuse of alcohol significantly increases morbidity and mortality from infectious diseases along with the risk of cardiovascular, brain, pancreatic, renal, cerebral, and oncological diseases [13]. The overall socioeconomic cost of alcohol abuse including direct (drug and hospitalization expenses) and indirect (due to loss of work productivity, crime, and reduction in health-related quality of life) costs has been estimated to be more than $185 million annually in the United States [20,25]. Despite the profound economic and health impact of ALD, many patients continue to consume alcohol. The primary treatment recommendation for this disease is abstinence from alcohol. Other treatment options, such as pharmacological and nutritional therapies, are also available [17]. Interest in potential antioxidant therapy for treating AH has increased as a growing body of evidence indicates that oxidative stress is a key mechanism underlying alcohol-mediated hepatotoxicity. Alcohol intoxication leads to increased generation of reactive oxygen species (ROS) while suppressing the antioxidant defense system [28,31]. Antioxidants have the ability to scavenge/deactivate ROS and inhibit the oxidation of various cellular substances. Several antioxidants such as S-adenosylmethionine, N-acetylcysteine, beta-carotene, vitamin C, vitamin E, and selenium have been systematically evaluated [12,18]. It was reported that AH patients treated with vitamin E, selenium, and zinc supplements had an in-hospital mortality rate of 6.5% compared to a mortality rate of 40% for the placebo group [17]. Plants produce an extensive variety of antioxidant compounds such as phenolics, terpenoids, alkaloids, and bioactive protein or peptides. These reagents can be used as ingredients in nutritional supplements/nutraceuticals that combat oxidative stress with minimal side effects [35].
Betulinic acid (BA; 3β-hydroxy-lup-20(29)-en-28-oic acid) is a pentacyclic lupane-type triterpene (Fig. 1A) that exists widely in food, medicinal herbs, and plants, especially birch bark [9]. As an oxidation product of betulin (see Fig. 1B), BA has also been detected in extracts of white birch bark [9,32]. These reagents are of particular interest due to their wide spectrum of biological activities such as antioxidant, anti-inflammatory, anti-tumor, anti-angiogenesis, anti-viral, anti-HIV, anti-neoplastic, and anti-plasmodial properties [10,11,22,24,32]. BA was not found to be toxic at a concentration of 500 mg/kg in mice [22].
Several studies have demonstrated that natural triterpenes such as lupeol, betulin, ursolic acid, and oleanolic acid effectively reduce hepatotoxicity induced by carbon tetrachloride, acetaminophen, ethanol, and cadmium in vivo and in vitro [29,38]. The protective effect of betulin, BA, and oleanolic acid against ethanol-induced cytotoxicity in HepG2 cells has also been reported [28]. The mechanisms underlying this effect include the suppression of enzymes activities that play roles in liver damage such as cytochrome P450 (CYP450), cytochrome B5 (CYB5), and cytochrome P4501A (CYP1A), or increased production of antioxidant substances including catalase (CAT), superoxide dismutase (SOD), glutathione (GSH), and metallothioneins that help protect liver mitochondria [28]. In vitro, BA inhibits ethanol-induced activation of hepatic stellate cells, as a antioxidant, suppressing of ROS, tumor necrosis factor-α (TNF-α) and transforming growth factor-β (TGF-β) production as well as nuclear factor-kappa B/inhibitory protein of NF-κB (NF-κB/IκB) transduction signaling [28,29]. However, ability of BA to confer in vivo protection against liver injury induced by alcohol has not been previously reported. The objective of this study was to investigate the possible hepatoprotective effects of BA against alcohol induced acute liver damage in mice and elucidate the underlying mechanism.
White birch bark samples were collected during the spring of 2009 in Wroclaw, Poland. All the collected bark samples were immediately dried at 60℃ and stored in a dry, dark place. To extract betulin, about 15 g of dried bark were subjected to reflux with 200 mL methanol for 3 h at 70℃. The methanol extract was dried under negative gauge pressure and dissolved in 150 mL dichloromethane. After adding 150 mL 2 M sodium hydroxide and mixing, the lower liquid layer was collected and then filtered under negative gauge pressure. The residue was dissolved in 200 mL ether. Next, 200 mL water was added and the solution was thoroughly mixed. The upper liquid layer was subsequently collected, dried, and subjected to silica gel column chromatography (Flash column; Aisimo Corporation, China) [32]. Betulin was fractionated with hexane and ethyl acetate (6 : 1, v : v). BA was synthesized in two steps: 1) the betulin was oxidized with Jones reagent (1 : 6, molar ratio) at 20℃ for 3 h to obtain betulonic acid, and 2) betulonic acid was reduced by sodium borohydride (1 : 1, molar ratio, Sigma-Aldrich, USA) in 10 mL tetrahydrofuran (Sigma-Aldrich) to produce a mixture of 3α- and 3β-hydroxyl products (5 : 95) as previously described [9,32]. Crystallization of the product from methanol resulted in the 3β-hydroxyl product (BA) with a yield of 75%.
The semi-synthetic compound was a white powder, and characterized using Fourier transform infrared spectroscopy (FI-IR), high performance liquid chromatography-mass spectrometery (HPLC-MS), and nuclear magnetic resonance (NMR) as previously described [32]. Purity of the BA was measured with HPLC using a Zorbax Eclipse XDB-C8 column (4.6 × 150 mm, 5 µm; Agilent Technologies, USA). The injection volume was 20 µL and the column was eluted with an ethanol : water solution (86 : 14, v : v, 4 g/L ammonium formate in water) as the mobile phase at a flow rate of 1 mL/min, and UV detection was performed at 210 nm at room temperature. The Purity of the BA was 96.5%.
A total of 50 male Kunming mice weighing 18~22 g (6 weeks old) were purchased from Hunan Silaikejingda Laboratory Animal Co., Ltd. (China). The mice were acclimated to the laboratory environment for 1 week before the experiment was initiated, and fed an M02 standard mouse diet from Hunan Silaikejingda Laboratory Animal (China). The mice had access to food and water ad libitum, and were maintained at a constant temperature (23 ± 1℃) and humidity (60 ± 10%) with a 12-h light/dark cycle. The present study complied with the Animal Care and Use Guidelines of China and was approved by the Animal Care Committee of Hunan Agricultural University (China) [15].
The dose of BA and duration of treatment were established based on a previous study [37]. The mice were randomly divided into five groups: group A served as the control (no alcohol, no BA), group B served as the alcoholic liver injury model (alcohol, no BA), group C received a low dose of BA (0.25 mg/kg) and alcohol, group D received a moderate dose of BA (0.5 mg/kg) and alcohol, and group E consumed a high dose of BA (1 mg/kg) and alcohol. BA was administered orally to most mice with 1% starch jelly (Sinopharm Chemical Reagent, China) every day for 14 days while animals in groups A and B were given an equivalent amount of 1% starch jelly only. The mice were fasted overnight (16~18 h) before receiving a single dose of 50% ethanol (Sinopharm Chemical Reagent) at the dosage of 10 mL/kg on the 14th day of the study 1 h after the last administration of BA. Group A received an equal volume of sterile saline.
Nine h after alcohol administration, blood samples were collected in tubes (Eppendorf, Germany) without anticoagulant by venous puncture with the mice under light anesthesia induced by diethyl ether (Sinopharm Chemical Reagent). The animals were then sacrificed by cervical dislocation and liver samples were collected. Serum was collected by centrifugation (Z383K Universal High Speed Centrifuge; Hermle labortechnik, Germany) at 2,000 × g for 10 min at 4℃ and frozen at -70℃ until analysis. Livers were quickly removed, weighed, and the liver indices expressed in milligrams of liver per 10 g of body weight were calculated. The liver tissues were washed in a chilled 0.9% NaCl solution and minced into small pieces on ice. A 10% (w/v) homogenate (Tenbroeck tissue grinders; Wheaton, USA) was prepared in 10 mM phosphate buffer (pH 7.4) and centrifuged (Z383K Universal High Speed Centrifuge; Hermle labortechnik) at 2,500 × g for 15 min at 4℃. The resulting supernatant was collected and stored at -70℃ until analysis.
Serum alanine transaminase (ALT), aspartate transaminase (AST), total cholesterols (TC), and triacylglycerides (TG) levels were measured using Mindray commercial kits and a Mindray BS-200 automatic biochemistry analyzer (Shenzhen Mindray Bio-Medical Electronics, China). ALT and AST levels were expressed as units per liter of serum (U/L) while TC and TG concentrations were expressed as nmol per liter of serum (nmol/L).
The activities of superoxide dismutase (SOD), glutathione peroxidase (GSH-Px), and catalase (CAT) were measured along with malondialdehyde (MDA) and glutathione (GSH) levels using commercial kits (Nanjing Jiancheng Bioengineering Institute, China) according to the manufacturer's protocols. The levels of SOD, GSH-Px, and CAT activities were expressed as unit per mg of protein (U/mg protein). The MDA and GSH levels were expressed as nmol per mg protein (nmol/mg protein) and mg per g of protein (mg/g protein), respectively. Liver protein concentrations were determined with a Bradford assay using commercial kits (Nanjing Jiancheng Bioengineering Institute) according to the manufacturer's protocols.
Liver tissues from the mice were removed and fixed in 10% neutral buffered formalin. The tissues were then rinsed with water, dehydrated with ethanol, subsequently embedded in paraffin, and sectioned into slices (4-µm-thick) using a Leica RM2235 rotary microtome (Leica Microsystems, Germany). The sections were mounted onto glass slides (Beyotime Institute of Biotechnology, China), dewaxed in xylene and ethanol, and stained with hematoxylin and eosin (Beyotime Institute of Biotechnology, China) for histological evaluation using a Motic BA410 microscope (Motic Incorporation, China). Image Pro-Plus Motic Med 6.0 software (Motic Incorporation) was used for image processing.
The results are presented as the mean ± standard deviation (SD). The data were analyzed with a one-way analysis of variance (ANOVA) followed by the Student-Newman-Keuls test for multiple comparisons. All statistical analyses were performed using the SPSS 16.0 statistical package (SPSS, USA). A p value < 0.05 was considered statistically significant and a p value < 0.01 was considered highly significant.
The effects of BA on alcohol-affected liver indices along with serum levels of ALT, AST, TC, and TG in mice were assessed (Table 1). The liver indices of group B were increased (p < 0.05) after alcohol treatment compared to those in the control group A. Pretreatment with BA significantly restored the liver indices of groups C, D, and E in a dose-dependent manner compared to those of group B.
The activities of ALT and AST in alcohol-treated group B were increased by 45.3% and 130.5%, respectively, compared to those found in the control (group A; p < 0.01). Pretreatment with BA reduced both serum ALT and AST levels in a dose-dependent manner. Serum ALT and AST activities were decreased by 7.6% and 9.4% (p < 0.05), 17.8% and 17.0% (p < 0.01), and 25.4% and 30.9% (p < 0.01), respectively, in groups C, D, and E compared to those observed in group B. At the same time, the serum concentrations of TC and TG in group B were increased by 15.7% and 38.7%, respectively, relative to group A (p < 0.05). BA pretreatment reduced the concentrations of TC and TG in a dose-dependent manner. Serum TC and TG levels were decreased by 6.0% and 11.1%, 11.3% and 18.2% (p < 0.05), and 12.8% and 24.5% (p < 0.05), respectively, in groups C, D, and E compared to group B. These findings indicate that BA pretreatment conferred significant protection against alcohol-induced fatty liver by reducing elevated serum levels of ALT, AST, TC and TG in a dose-dependent manner.
SOD, CAT, and GSH-Px are important enzymes in the antioxidant defense system. Hepatic activities of these three factors decreased significantly in alcohol-treated mice (group B) compared to the control group A (Fig. 2). Pretreatment with BA (groups C, D, and E) increased the activities of SOD, CAT, and GSH-Px in a dose-dependent manner compared to treatment with alcohol alone (group B).
MDA is a major biomarker of lipid peroxidation in tissues. GSH is an antioxidant that helps protect cells against ROS. As shown in Fig. 3, MDA levels was remarkably increased by 87.5% (p < 0.01) while GSH levels were significantly decreased by 46.3% (p < 0.01) in the alcohol-treated animals (group B) 9 h after alcohol administration compared to the control group A. BA pretreatment significantly decreased hepatic MDA contents while increased GSH levels in a dose- dependent manner. MDA contents were decreased by 12.8%, 17.4%, and 39.7% while the GSH levels were increased by 39.4%, 54.8%, and 66.0% in groups C, D, and E, respectively, compared to group B (Fig. 3).
To confirm the protective effect of BA on alcohol-induced liver tissue damage, histological examinations were also performed. The control group had normal hepatic architecture (Fig. 4A) whereas the mice treated with alcohol alone exhibited diffuse cytoplasmic vacuolization (Fig. 4B) indicative of hepatocellular steatosis with no signs of necro-inflammation. In contrast, BA pretreatments reduced the degree of alcohol-induced micro-vacuolization in the liver in a dose-dependent manner (Figs. 4C, D, and E). These results showed that BA protected the mouse livers against alcohol-induced hepatic steatosis, a known indicator of alcohol toxicity.
BA is a naturally occurring plant-derived pentacyclic triterpenoid present in many fruits and vegetables such as Tecomella undulata, Coussarea paniculata, Caesalpinia paraguariensis, Vitex negundo, and Ilex macropoda [14,33]. Although BA is widely available from numerous botanical sources, BA contents in these sources are extremely low. Alternatively, BA can be chemically synthesized from betulin, a closely related compound. White bark birch trees (Betula species), a major source of betulin, are widely distributed in the northern hemisphere. Betulin accounts for up to 30% of the dry weight of birch bark extract [9]. Therefore, a semi-synthetic method for preparing BA from betulin was used to obtain sufficient quantities of this bioactive triterpene for the current study.
Alcohol abuse affects the morphology and function of almost all organs in the body [27,31]. Liver is one of the prime target organs of alcohol-related diseases. Alcohol abuse results in hepatocytes damage, liver injury, and inflammation that lead to increased cell permeability and leakage of AST and ALT. Hence, elevated activities of AST and ALT in the plasma are hallmarks of hepatic damage [14,23]. In the present study, alcohol treatment significantly increased AST and ALT activities. These activities significantly decreased when the mice were pretreated with BA. The results were in agreement with previous findings showing that mice pretreated with BA have significantly reduced serum levels of ALT and AST elevated by d-galactosamine/lipoplysaccharide [38], indicating that the hepatocytes sustained minimal injury and their membranes were stabilized.
Alcohol alters the fatty acid composition in the liver. It has been demonstrated that increased cellular nicotinamide adenine dinucleotide hydrogen concentrations and acetaldehyde dehydrogenase activity may impair fatty acid β-oxidation and the tricarboxylic acid cycle during alcohol metabolism, leading to severe free fatty acid overload, increased TG synthesis, and subsequent steatosis [6,27]. Results from the present study showed that BA pretreatment could protect against alcohol-induced fatty liver by reducing serum levels of TC and TG as well as liver indices, which was most likely due to increased β-oxidation in the liver and energy expenditure. BA also ameliorated steatosis (Fig. 4), indicating that BA decreased lipid accumulation in the visceral adipocytes of alcohol-treated mice. This in turn could prevent toxic complications resulting from increased levels of hepatic lipids. Previously, it was also reported that BA functions as both a hypolipidemic and hypoglycemic agent when administered daily to mice fed a high-fat diet [8].
Alcohol affects the liver by inducing nutritional disturbances along with its predominant metabolism in the liver associated with oxidation-reduction (redox) reactions and oxidative stress [26]. In hepatocytes, oxidation of alcohol into water and carbon dioxide is mediated by three known enzyme systems: alcohol dehydrogenase (ADH) in the cytoplasm, the microsomal ethanol oxidizing system in the smooth endoplasmic reticulum of mitochondria, and CAT in peroxisomal membranes [13]. All of these biochemical pathways produce acetaldehyde, a highly reactive product of alcohol oxidative metabolism that damages mitochondria and promotes glutathione depletion, free radical-mediated toxicity, and lipid peroxidation [12]. Alcohol also increases liver oxidative stress via the generation of ROS and adducts. Excessive ROS or a defect of antioxidant system can directly lead to hepatocyte damage and enhance the sensitivity of hepatocytes to lipid peroxidation [27]. Endogenous enzymatic and non-enzymatic antioxidants protect the intracellular milieu against various toxic factors. Elevated lipid peroxidation results in significant depletion of cellular antioxidants [14]. Cellular GSH levels can be decreased through ROS-induced GSH oxidation or GSH export from the cells. Reduced GSH concentrations further enhance ROS production during oxidative challenge [7]. Moreover, BA significantly inhibits ROS production by hepatic stellate cells treated with ethanol [29]. All of these observations indicate that BA exerts prominent antioxidant effects against alcohol-induced oxidative damage.
Oxidative stress results in apoptosis and cell damage, and can directly disrupt the intracellular redox state or indirectly activate signal transduction pathways [37]. Apoptosis is triggered by extrinsic and intrinsic signals via two main pathways: the death receptor and mitochordria-mediated cascades [16]. The death receptor pathway involves ligand-receptor binding initiated by proteinprotein interactions at the cell membranes that activate initiator caspases [1]. Ligands that activate death receptors belong to the tumor necrosis factor (TNF) superfamily of cytokines that includes TNF-α, Fas ligand (FasL), and tumor necrosis factor-alpha-related apoptosis-inducing ligand (TRAIL) [2]. TNF-α, one of the most important proinflammatory mediators, induces hepatocyte apoptosis and necrosis through the activation of caspase-3, thereby leading to hepatocellular DNA fragmentation [36]. Several studies have shown that oxidative stress in cases of alcohol liver injury could result in the up-regulation of TNF-α expression [19,29,38]. TNF-α-induced intracellular ROS generation is markedly decreased by pretreatment with BA [34]. Moreover, BA decreases ethanol-induced TNF-α and TGF-β expression, probably through its ability to inhibit the NF-κB pathway [19,29,38].
B-cell lymphoma/leukemia-2 (Bcl-2) is an anti-apoptotic protein, and Bcl-2-associated X protein (Bax) and Bcl-2 antagonist/killer (Bak) are pro-apoptotic proteins that are important regulators of apoptosis in the mitochondrial pathway [4,30]. BA promotes the expression of Bcl-2 and suppresses acute liver damage by preventing apoptosis [38]. Additionally, ROS activation of c-Jun NH2-terminal kinase (JNK) can induce extrinsic or intrinsic apoptotic signaling. Previous reports have shown that BA significantly inhibits the production of ROS and down-regulates JNK activity in hepatic stellate cells treated with ethanol [21,29]. Therefore, the protective effects of BA against alcohol-induced liver injury possibly involve both exterior and interior pathways.
In conclusion, the present study demonstrated that BA exerts potent hepatoprotective effects that reduce alcohol-induced liver damage in mice. The primary mechanisms responsible for the hepatoprotective activities of BA might be due to the antioxidant capacity of this compound that improve the tissue redox system, maintain the antioxidant system, and decrease lipid peroxidation in the liver. These properties may involve both external and internal pathways. BA has a great potential for use as a beneficial nutraceuticals or dietary supplements for treating alcohol-related diseases. Further investigation is necessary to determine the exact mechanism governing the ability of BA to prevent alcohol-induced liver damage. It is also worth investigating the in vivo and in vitro roles of BA in apoptotic pathways that include signal transduction cascades and pro-apoptotic cytokines.
Figures and Tables
Fig. 2
Effects of BA on SOD (A), CAT (B), and GSH-Px (C) levels altered by alcohol in the liver of mice. Values are presented as the mean ± SD. ap < 0.05 and bp < 0.01 compared to the control group (group A). cp < 0.05 and dp < 0.01 compared to the group treated with alcohol alone (group B).
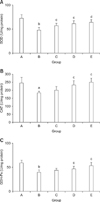
Fig. 3
Effects of BA on alcohol-induced changes of MDA (3) and GSH (3) contents in mouse liver. Values are presented as the mean ± SD. ap < 0.01 compared to the control group (group A). bp < 0.05 and cp < 0.01 compared to the mice treated with alcohol alone (group B).
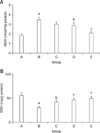
Fig. 4
Histopathological changes in mouse liver (H&E staining, 400× magnification). No steatosis was observed in the control group (A). Diffuse cytoplasmic vacuolization (arrow) indicative of steatosis was observed in mice treated with alcohol alone (B). Hepatocellular steatosis was alleviated in a dose-dependent manner by the administration of 0.25 mg/kg (C), 0.5 mg/kg (D), and 1 mg/kg (E) BA for 14 days. Scale bars = 10 µm.
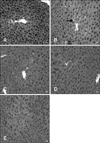
Acknowledgments
Our research was supported by the National Natural Science Foundation of China (Grant No. 31201964), Research Fund for the Doctoral Program of Higher Education of China (Grant No. 20124320120011), Department of Education of Hunan Province, China (Key project No. 12A066), and the Hunan Provincial Key Laboratory of Crop Germplasm Innovation and Utilization of China (Opening Science Fund No. 12KFXM08).
References
1. Ashkenazi A, Dixit VM. Apoptosis control by death and decoy receptors. Curr Opin Cell Biol. 1999; 11:255–260.


2. Berg D, Lehne M, Müller N, Siegmund D, Münkel S, Sebald W, Pfizenmaier K, Wajant H. Enforced covalent trimerization increases the activity of the TNF ligand family members TRAIL and CD95L. Cell Death Differ. 2007; 14:2021–2034.


3. Brandon-Warner E, Schrum LW, Schmidt CM, McKillop IH. Rodent models of alcoholic liver disease: of mice and men. Alcohol. 2012; 46:715–725.


4. Brenner C, Cadiou H, Vieira HLA, Zamzami N, Marzo I, Xie Z, Leber B, Andrews D, Duclohier H, Reed JC, Kroemer G. Bcl-2 and Bax regulate the channel activity of the mitochondrial adenine nucleotide translocator. Oncogene. 2000; 19:329–336.


5. Cook RT. Alcohol abuse, alcoholism, and damage to the immune system-a review. Alcohol Clin Exp Res. 1998; 22:1927–1942.


6. Crabb DW. Recent developments in alcoholism: the liver. Recent Dev Alcohol. 1993; 11:207–230.
7. D'Alessio M, Cerella C, De Nicola M, Bergamaschi A, Magrini A, Gualandi G, Alfonsi AM, Ghibelli L. Apoptotic GSH extrusion is associated with free radical generation. Ann N Y Acad Sci. 2003; 1010:449–452.
8. de Melo CL, Queiroz MGR, Arruda Filho ACV, Rodrigues AM, de Sousa DF, Almeida JGL, Pessoa ODL, Silveira ER, Menezes DB, Melo TS, Santos FA, Rao VS. Betulinic acid, a natural pentacyclic triterpenoid, prevents abdominal fat accumulation in mice fed a high-fat diet. J Agric Food Chem. 2009; 57:8776–8781.


9. Flekhter OB, Nigmatullina LR, Baltina LA, Karachurina LT, Galin FZ, Zarudii FS, Tolstikov GA, Boreko EI, Pavlova NI, Nikolaeva SN, Savinova OV. Synthesis of betulinic acid from betulin extract and study of the antiviral and antiulcer activity of some related terpenoids. Pharm Chem J. 2002; 36:484–487.
10. Fujioka T, Kashiwada Y, Kilkuskie RE, Cosentino LM, Ballas LM, Jiang JB, Janzen WP, Chen IS, Lee KH. Anti-AIDS agents, 11. Betulinic acid and platanic acid as anti-HIV principles from Syzigium claviflorum, and the anti-HIV activity of structurally related triterpenoids. J Nat Prod. 1994; 57:243–247.


11. Fulda S. Betulinic acid: a natural product with anticancer activity. Mol Nutr Food Res. 2009; 53:140–146.


12. Gao B, Bataller R. Alcoholic liver disease: pathogenesis and new therapeutic targets. Gastroenterology. 2011; 141:1572–1585.


13. Gramenzi A, Caputo F, Biselli M, Kuria F, Loggi E, Andreone P, Bernardi M. Review article: alcoholic liver disease-pathophysiological aspects and risk factors. Aliment Pharmacol Ther. 2006; 24:1151–1161.


14. Jain M, Kapadia R, Jadeja RN, Thounaojam MC, Devkar RV, Mishra SH. Hepatoprotective potential of Tecomella undulate stem bark is partially due to the presence of betulinic acid. J Ethnopharmacol. 2012; 143:194–200.


15. Kong Q, Qin C. Analysis of current laboratory animal science policies and administration in China. ILAR J. 2009; 51:e1–r10.


16. Mandal D, Mazumder A, Das P, Kundu M, Basu J. Fas-, Caspase 8-, and Caspase 3-dependent signaling regulates the activity of the aminophospholipid translocase and phosphatidylserine externalization in human erythrocytes. J Biol Chem. 2005; 280:39460–39467.


17. Marsano LS, Mendez C, Hill D, Barve S, McClain CJ. Diagnosis and treatment of alcoholic liver disease and its complications. Alcohol Res Health. 2003; 27:247–256.
18. Mato JM, Cámara J, Fernández de Paz J, Caballería L, Coll S, Caballero A, García-Buey L, Beltrán J, Benita V, Caballería J, Solá R, Moreno-Otero R, Barrao F, Martín-Duce A, Correa JA, Parés A, Barrao E, García-Magaz I, Puerta JL, Moreno J, Boissard G, Ortiz P, Rodés J. S-adenosylmethionine in alcoholic liver cirrhosis: a randomized, placebo-controlled, double-blind, multicenter clinical trial. J Hepatol. 1999; 30:1081–1089.


19. Nanji AA, Jokelainen K, Rahemtulla A, Miao L, Fogt F, Matsumoto H, Tahan SR, Su GL. Activation of nuclear factor kappa B and cytokine imbalance in experimental alcoholic liver disease in the rat. Hepatology. 1999; 30:934–943.


20. Neff GW, Duncan CW, Schiff ER. The current economic burden of cirrhosis. Gastroenterol Hepatol (N Y). 2011; 7:661–671.
21. Nishitani Y, Matsumoto H. Ethanol rapidly causes activation of JNK associated with ER stress under inhibition of ADH. FEBS Lett. 2006; 580:9–14.


22. Pisha E, Chai H, Lee IS, Chagwedera TE, Farnsworth NR, Cordell GA, Beecher CWW, Fong HHS, Kinghorn AD, Brown DM, Wani MC, Wall ME, Hieken TJ, Das Gupta TK, Pezzuto JM. Discovery of betulinic acid as a selective inhibitor of human melanoma that functions by induction of apoptosis. Nat Med. 1995; 1:1046–1051.


23. Raghu R, Liu CT, Tsai MH, Tang X, Kalari KR, Subramanian S, Sheen LY. Transcriptome analysis of garlic-induced hepatoprotection against alcohol fatty liver. J Agric Food Chem. 2012; 60:11104–11119.


24. Recio MC, Giner RM, Máñez S, Gueho J, Julien HR, Hostettmann K, Ríos JL. Investigations on the steroidal anti-inflammatory activity of triterpenoids from Diospyros leucomelas. Planta Med. 1995; 61:9–12.


25. Rehm J, Mathers C, Popova S, Thavorncharoensap M, Teerawattananon Y, Patra J. Global burden of disease and injury and economic cost attributable to alcohol use and alcohol-use disorders. Lancet. 2009; 373:2223–2233.


26. Steinkamp-Fenske K, Bollinger L, Xu H, Yao Y, Horke S, Förstermann U, Li H. Reciprocal regulation of endothelial nitric-oxide synthase and NADPH oxidase by betulinic acid in human endothelial cells. J Pharmacol Exp Ther. 2007; 322:836–842.


27. Sun F, Xie ML, Zhu LJ, Xue J, Gu ZL. Inhibitory effect of osthole on alcohol-induced fatty liver in mice. Dig Liver Dis. 2009; 41:127–133.


28. Szuster-Ciesielska A, Kandefer-SzerszeXMLLink_XYZ M. Protective effects of betulin and betulinic acid against ethanol-induced cytotoxicity in HepG2 cells. Pharmacol Rep. 2005; 57:588–595.
29. Szuster-Ciesielska A, Plewka K, Daniluk J, Kandefer-SzerszeXMLLink_XYZ M. Betulin and betulinic acid attenuate ethanol-induced liver stellate cell activation by inhibiting reactive oxygen species (ROS), cytokine (TNF-α, TGF-β) production and by influencing intracellular signaling. Toxicology. 2011; 280:152–163.


30. Vyssokikh MY, Zorova L, Zorov D, Heimlich G, Jürgensmeier JJ, Brdiczka D. Bax releases cytochrome c preferentially from a complex between porin and adenine nucleotide translocator. Hexokinase activity suppresses this effect. Mol Biol Rep. 2002; 29:93–96.
31. Wang M, Zhu P, Jiang C, Ma L, Zhang Z, Zeng X. Preliminary characterization, antioxidant activity in vitro and hepatoprotective effect on acute alcohol-induced liver injury in mice of polysaccharides from the peduncles of Hovenia dulcis. Food Chem Toxicol. 2012; 50:2964–2970.


32. Yi J, Obminska-Mrukowicz B, Yuan L, Yuan H. Immunomodulatory effects of betulinic acid from the bark of white birch on mice. J Vet Sci. 2010; 11:305–313.


33. Yogeeswari P, Sriram D. Betulinic acid and its derivatives: a review on their biological properties. Curr Med Chem. 2005; 12:657–666.


34. Yoon JJ, Lee YJ, Kim JS, Kang DG, Lee HS. Protective role of betulinic acid on TNF-α-induced cell adhesion molecules in vascular endothelial cells. Biochem Biophys Res Commun. 2010; 391:96–101.


35. Yu HY, Wang BL, Zhao J, Yao XM, Gu Y, Li Y. Protective effect of bicyclol on tetracycline-induced fatty liver in mice. Toxicology. 2009; 261:112–118.


36. Yuan HD, Jin GZ, Piao GC. Protective effects of the supernatant of ethanol eluate from Artemisia sacrorum ledeb. against acetaminophen-induced liver injury in mice. Biol Pharm Bull. 2009; 32:1683–1688.

