Abstract
This study evaluated pulsed TDI variables including the isovolumic time interval and duration of the major wave in a population of large healthy dogs. Longitudinal myocardial motion at the septal mitral annulus was evaluated with pulsed TDI in 45 healthy adult dogs. Maximal myocardial velocities, isovolumic time intervals, and duration of the myocardial waves were measured. The correlation between time intervals and velocity variables was also investigated. The mean maximal systolic velocity was 6.92 ± 1.78 cm/sec, the mean early diastolic velocity (Em) was 6.58 ± 1.81 cm/sec, the mean late diastolic velocity (Am) was 5.10 ± 2.00 cm/sec, the mean isovolumic contraction time (IVCT) was 53.61 ± 95.13 msec, and the mean isovolumic relaxation time (IVRT) was 26.74 ± 57.24 msec. The early diastolic mitral inflow velocity (E)/Em ratio was 10.94 ± 3.27 while the Em/Am ratio was 1.40 ± 0.40. There was a negative correlation between Am duration and Am amplitude, and a positive correlation between the IVRT and Em/Am ratio (p < 0.05). The normal LV parameter using pulsed TDI method could be used as the reference range for identifying myocardial dysfunction in dogs.
Diagnoses of myocardial disorders have been predominantly based on two-dimensional (2D), M-mode, and Doppler mode, also called conventional echocardiography in human as well as veterinary cardiology. However, the ability to estimate functional alterations of the myocardium during the early stages of cardiac disease with conventional echocardiography is critically limited. Tissue Doppler imaging (TDI) has emerged as one of the most sensitive and specific methods for noninvasive assessment of systolic and diastolic function in the early stages of cardiac disease [3,12,20,23]. It has been reported in humans that the power of TDI to predict fatalities attributed to cardiac disease by measuring the velocity of the mitral annulus in early diastole is greater than the predictive power of clinical data and conventional echocardiographic measurements [23].
TDI detects low velocity and high amplitude signals from the myocardium as opposed to blood flow signals with high velocity and low amplitude [3]. TDI has three modes including color 2D, color M-mode, and pulsed wave mode. Color 2D and color M-mode are capable of analyzing multiple segments simultaneously and determining the velocity gradient of the myocardium. However, specialized software is needed for post-processing analysis, so TDI variables cannot be measured in real time. Pulsed TDI can estimate myocardial motion without post-processing procedures, but simultaneous analysis of multiple segments cannot be performed. Nevertheless, pulsed TDI has been used in humans to evaluate systolic and diastolic left ventricle (LV) function in healthy subjects and patients with various cardiac diseases such as dilated cardiomyopathy (DCM), hypertrophic cardiomyopathy (HCM), restrictive cardiomyopathy, constrictive pericarditis, ischemic cardiomyopathy, congestive heart failure (CHF), mitral valve (MV) regurgitation, atrial hypertension, and cardiac amyloidosis [16,23].
Many TDI studies, particularly ones on DCM and HCM, have been performed in dogs and cats since the technique was introduced to veterinary medicine [5-7,18,21]. However, a reliable normal range of TDI variables in dogs, particularly for the pulsed wave mode, has not yet been established and no detailed study of isovolumic time intervals or major wave duration has been performed. The purpose of the present investigation was to prospectively evaluate the motion of the MV septal annulus in healthy dogs, establish reference values of pulsed TDI variables for longitudinal motion, and investigate the correlation between time variables and the velocity of myocardial motion.
Total 45 healthy adult dogs less than 10 years old were included in this study. All dogs were privately owned as pets, and consent was received from each owner to enroll the animals in this study. Every dog was in good health and had no history of heart or respiratory diseases. All animals were determined to be healthy based on physical examinations that included the evaluation of capillary refill time, mucous membrane color, cardiac auscultation, palpation of the femoral arterial pulse, blood pressure, resting heart and respiratory rates, complete blood count, serum chemistry, electrocardiogram (ECG) data, thoracic radiography findings, and conventional echocardiography results. Systolic arterial blood pressure was measured indirectly using a standard Doppler method (Model 811-B; Parks Medical Electronics, USA).
Standard echocardiography with continuous ECG monitoring was performed by one investigator (J.H. Choi, Chonnam National University, Korea) using an ultrasonograph unit (ProSound SSD-4000SV; Hitachi Aloka, Japan) equipped with a 7.5-MHz phased-array transducer. Echocardiography was conducted while the dogs were gently restrained in a lateral recumbent position without sedation or anesthesia. Cardiac geometry as well as the shape and motion of the atrioventricular valves on right and left approach were evaluated in two dimensions. Aortic and left atrial diameters were measured using the right parasternal short-axis views at the level of the aortic valve where the commissures of the cusps were visible during diastole [10]. LV parameters were measured and the fractional shortening (FS) was calculated in M-mode at the level of the chordae tendinae guided from the right parasternal short-axis view. Maximal velocities of LV inflow during early diastole (E) and late diastole (A) were measured in the left apical four-chamber view in the spectral Doppler mode. A mean of three measurements was obtained for each variable during three nonconsecutive cardiac cycles.
Pulsed TDI examination was performed with the same ultrasonograph unit used for conventional echocardiography while the dogs were physically restrained in a lateral position with continuous ECG monitoring. To evaluate the longitudinal myocardial motion, a 2-mm sample volume gate was placed on the annulus of the MV in the left apical four-chamber view. No angle correction was performed, and the Nyquist limit was adjusted to less than 15 cm/sec to obtain the maximal velocity signal while avoiding aliasing. Gain and filter settings were optimized to reduce noise. Systolic (Sm), early diastolic (Em), and late diastolic (Am) motion of the septal mitral annulus were recorded three nonconsecutive times and averaged in the chosen tracings that showed the highest velocities with minimal artifacts. Two time interval phases with pressure changes and a steady LV volume were measured. Isovolumic contraction time (IVCT) was measured from the end of Am to the beginning of Sm, and isovolumic relaxation time (IVRT) was determined based on the end of Sm to the beginning of Em. The duration of each myocardial wave was measured as duration of Sm (DurSm), duration of Em (DurEm), and duration of Am (DurAm). The correlation between myocardial velocity and time variables was investigated. Additionally, the Em/Am ratio and the ratio of late diastolic velocity of LV inflow to Em were calculated.
Data are expressed as the mean ± SD. Correlations between the myocardial velocity and time variables were analyzed using Pearson correlation. Statistical analysis was performed using SPSS (ver. 15.0; IBM, USA), and a 95% confidence interval was applied. A p-value less of than 0.05 was considered significant.
Forty-five dogs (16 sexually intact and eight castrated males; 18 sexually intact and three spayed females) of 11 breeds (six Shih Tzus, five cocker spaniels, five Maltese, five poodles, five schnauzers, four Yorkshire terriers, three beagles, three Dachshunds, one Pekingese, one Pomeranian, and fived mixed) were studied. The mean age was 4.95 ± 2.95 years (range, 1 to 9.6 years), and mean weight was 5.41 ± 2.91 kg (range, 1.3 to 14 kg). All dogs were determined to be healthy with a mean heart rate of 96.41 ± 37.78 beats/min and mean blood pressure of 121.76 ± 22.05 mmHg. Normal cardiac function was confirmed by conventional echocardiography. The ratio between the diameter of the left atrium and aorta was 1.11 ± 0.12. The mean FS was 35.38 ± 8.45%. Mean E and A velocities of the LV inflow were 67.21 ± 10.3 cm/sec and 47.3 ± 7.94 cm/sec, respectively, and the E/A ratio was 1.41 ± 0.31.
TDI analysis clearly revealed that the MV annulus moved with different velocities. All velocity patterns included one positive wave (Sm), two negative waves (Em and Am), and two isovolumic time intervals (Table 1). The Em was consistently higher than the Am with a mean Em/Am ratio of 1.40 ± 0.40. The mean E/Em ratio was 10.94 ± 3.27. The correlations of IVCT with Sm and FS as well as the correlations of DurSm with Sm and FS were investigated (Fig. 1). There were no significant relationships among these variables. IVRT showed a positive correlation with the Em/Am ratio (p < 0.05), but there was no relationship between IVRT and Em or between IVRT and Am (Fig. 2). DurEm was not correlated with Em, but DurAm showed a negative correlation with Am (p < 0.05).
TDI examination can be performed in the longitudinal or radial axis of the myocardium. Depending on the axis, the myocardium fibers can appear different. Subepicardial and subendocardial layers of the myocardium consist of longitudinally arrayed oblique fibers that can be analyzed in the longitudinal axis. The mid-wall of the myocardium is composed of circumferential fibers that can be analyzed in the radial axis. In the present study, longitudinal motion was evaluated because the longitudinal axis may be less affected by rotational and translational cardiac activity than the radial axis. Furthermore, the longitudinal fibers may be affected first under pathologic conditions such as ischemia and hypertrophy [1,7,13]. The mean velocity of TDI variables measured in the longitudinal axis was reported to be higher than that in the radial axis [5]. Therefore, the normal range of TDI variables depends upon the axis.
Pulsed TDI measuring LV function can quantify the velocity of the myocardial wall and valve annulus motions [16,17,20]. The most common structure examined by pulsed TDI is the MV annulus [19]. Because the apex remains relatively stationary throughout the cardiac cycle, mitral annular motion is an appropriate surrogate measurement of overall longitudinal LV contraction and relaxation [22]. The MV septal annulus was used as the sampling location in the present study because it is reported to be the most effective position for assessing global cardiac function compared to the MV annulus of the LV free wall [7].
The mean velocity of TDI variables differs according to sampling position, so it is necessary to determine a normal range of values for each position. Pulsed TDI can only evaluate a single segment. Therefore, this technique only provides an estimate of regional cardiac function. Pulsed TDI cannot be used to evaluate the gradient of velocities according to segment. However, it can be performed in real time without specialized software. Hence, in practice pulsed TDI may be the first-choice modality for imaging. There have been many studies of TDI in dogs and cats [4-7], but only a few have been performed with pulsed TDI including a report on pulsed TDI variables in 71 healthy dogs [24].
Pulsed TDI measures three major waves: Sm, Em, and Am. Em and Am correspond to the early diastolic relaxation phase and atrial contraction, respectively [13]. Poor diastolic function is characterized by decreased Em, elevated Am, and an Em/Am ratio < 1 [19]. In conventional echocardiography, alterations of diastolic function characterized by an abnormal MV inflow pattern with a decreased E/A ratio indicate poor LV relaxation. However, these changes may be absent or equivocal during the early stages of disease. For example, increased left atrial pressure affects the balance between E and A, and causes "pseudonormalization" in cases of moderate to advanced diastolic dysfunction [19]. Mitral annular motion, unlike mitral inflow velocity, is relatively unaffected by changes in preload [19,20]. Thus, the Em/Am ratio typically remains < 1 even with advanced myocardial dysfunction. In the current study, the Em/Am ratio was 1.4 ± 0.36 in normal dogs, and this result concurs with findings from previous study [21].
By indexing mitral inflow velocity to TDI-derived mitral annular velocity, the effect of LV diastolic function can be uncoupled from the mitral inflow data, leaving an index of preload that can be used to estimate left atrial pressure [11]. In this way, TDI provides a non-invasive index of filling pressures that can be used to make clinical decisions regarding the presence or absence of CHF [11]. The E/Em ratio has been found to closely correlate with pulmonary capillary wedge pressure and LV diastolic pressure in humans with sinus tachycardia, DCM, and HCM [14,15,19]. The E/Em ratio in our study was 10.94 ± 3.27. A previous investigation [21] reported a mean E/Em ratio of 8.4 ± 1.1 and a cutoff value of 13, which provides diagnostic criteria for decompensated CHF with a sensitivity of 80% and specificity of 83%. However, the applicability of these results is limited because the investigators used only five normal dogs to establish the TDI variables and E/Em ratio. Because the E/Em ratio has proven to be the most powerful prognostic predictor for cardiac diseases [4], the cutoff E/Em ratio for identifying CHF has to be established through large population comparisons between healthy dogs and canines with various cardiac diseases. In the present study, the range of pulsed TDI variables in normal dogs was determined.
Using conventional echocardiography, IVCT and IVRT can be recorded by placing a pulsed wave Doppler gate or a continuous wave Doppler cursor in the LV outflow tract near the MVs and recording both aortic ejection flow and transmitral flow on the left apical or modified left apical views. IVCT is measured from the beginning of the Q wave on the ECG reading to the start of the ejection flow. IVRT is measured as the time from the end of ventricular ejection to the beginning of transmitral inflow. In our study, IVCT was determined by measuring the interval between the end of Am and the beginning of Sm. IVRT was measured from the end of Sm to the beginning of Em. When the duration of each wave was evaluated, there was a negative correlation between Am and DurAm, but no relationship existed between Sm and DurSm or between Em and DurEm. IVRT had no relationship with Em or Am, but there was a positive correlation between IVRT and the Em/Am ratio. IVCT did not show any relationship with Sm or FS. Based on these results, it may be concluded that these time variables have no clinical significance. However, the variables were not estimated with hemodynamic monitoring, so further study is necessary to determine the full value of these parameters. Wider IVCT and IVRT ranges were obtained compared to those for humans, and our results could be indicating that isovolumic time intervals might be affected by heart rate [2]. Therefore, isovolumic time intervals heart rate corrected can be used instead [9].
The present study had a few limitations. This investigation was carried out with animals privately owned as pets. Consequently, we were unable to generate hemodynamic data using more invasive methods to provide gold standards with which to compare our TDI results. This may have resulted in the inadvertent inclusion of animals with subclinical cardiac disorder in normal dogs. TDI variables have been reported to be less affected by certain factors like loading condition, age, and heart rate [8]. However, the effect of bodyweight and breed on TDI values is unclear [4]. This study was performed with mainly small dogs with bodyweights that were not significantly different. However, larger breeds may have different TDI values. Due to the angle dependence of the TDI technique, only the velocity component parallel to the ultrasonic beam was recorded. This may have led to an underestimation of the true myocardial velocities. However, sampling of the myocardial segments was consistently performed in the same echocardiographic view every time. Finally, intra- and inter-observer variability was not evaluated in the present study.
In summary, we successfully investigated the pulsed TDI variables for healthy dogs, including the isovolumic time interval and duration of the major wave. This study is clinically relevant because a large population of normal dogs was evaluated using pulsed TDI to assess the MV septal annuls. We plan to conduct a future study investigating the differences in normal ranges of pulsed TDI variables according to breed and body weight, and examine changes of these variables associated with different cardiac diseases.
Figures and Tables
Fig. 1
(A) The correlations between DurSm and myocardium systolic velocity of Sm, (B) between DurSm and fractional shortening (FS), (C) between IVCT and Sm, and (D) between IVCT and FS. No significant correlations were observed.
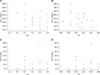
Fig. 2
(A) The correlations between DurEm with Em, and DurAm with Am (B). There was a negative correlation between DurAm and Am. Among the correlation (C) between IVRT and Am, (D) between IVRT and Em, and (E) between IVRT and Em/Am ratio, IVRT was positively correlated with the Em/Am ratio.
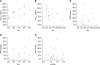
Acknowledgments
The animal experiment in this study was supported by the Animal Medical Institute of Chonnam National University, Korea.
References
1. Bolognesi R, Tsialtas D, Barilli AL, Manca C, Zeppellini R, Javernaro A, Cucchini F. Detection of early abnormalities of left ventricular function by hemodynamic, echo-tissue Doppler imaging, and mitral Doppler flow techniques in patients with coronary artery disease and normal ejection fraction. J Am Soc Echocardiogr. 2001. 14:764–772.


2. Bruch C, Schmermund A, Bartel T, Schaar J, Erbel R. Tissue Doppler imaging: a new technique for assessment of pseudonormalization of the mitral inflow pattern. Echocardiography. 2000. 17(6 Pt 1):539–546.


3. Cannesson M, Jacques D, Pinsky MR, Gorcsan J 3rd. Effects of modulation of left ventricular contractile state and loading conditions on tissue Doppler myocardial performance index. Am J Physiol Heart Circ Physiol. 2006. 290:H1952–H1959.


4. Chetboul V, Blot S, Sampedrano CC, Thibaud JL, Granger N, Tissier R, Bruneval P, Gaschen F, Gouni V, Nicolle AP, Pouchelon JL. Tissue Doppler imaging for detection of radial and longitudinal myocardial dysfunction in a family of cats affected by dystrophin-deficient hypertrophic muscular dystrophy. J Vet Intern Med. 2006. 20:640–647.


5. Chetboul V, Carlos C, Blot S, Thibaud JL, Escriou C, Tissier R, Retortillo JL, Pouchelon JL. Tissue Doppler assessment of diastolic and systolic alterations of radial and longitudinal left ventricular motions in Golden Retrievers during the preclinical phase of cardiomyopathy associated with muscular dystrophy. Am J Vet Res. 2004. 65:1335–1341.


6. Chetboul V, Gouni V, Sampedrano CC, Tissier R, Serres F, Pouchelon JL. Assessment of regional systolic and diastolic myocardial function using tissue Doppler and strain imaging in dogs with dilated cardiomyopathy. J Vet Intern Med. 2007. 21:719–730.


7. Chetboul V, Sampedrano CC, Tissier R, Gouni V, Saponaro V, Nicolle AP, Pouchelon JL. Quantitative assessment of velocities of the annulus of the left atrioventricular valve and left ventricular free wall in healthy cats by use of two-dimensional color tissue Doppler imaging. Am J Vet Res. 2006. 67:250–258.


8. Disatian S, Bright JM, Boon J. The effects of age and heart rate on tricuspid annular motion velocities in healthy nonsedated cats. J Vet Intern Med. 2007. 21:731–736.


9. Giorgi D, Di Bello V, Pedrinelli R, Bertini A, Talini E, Dell'Omo G, Mengozzi G, Palagi C, Dell'Anna R, Mariani M. Ultrasonic tissue characterization and Doppler tissue imaging in the analysis of left ventricular function in essential arterial hypertension: a preliminary study. Echocardiography. 2002. 19:187–198.


10. Hansson K, Häggström J, Kvart C, Lord P. Left atrial to aortic root indices using two-dimensional and M-mode echocardiography in cavalier King Charles spaniels with and without left atrial enlargement. Vet Radiol Ultrasound. 2002. 43:568–575.


11. Ho CY, Solomon SD. A clinician's guide to tissue Doppler imaging. Circulation. 2006. 113:e396–e398.


12. Isaaz K, Thompson A, Ethevenot G, Cloez JL, Brembilla B, Pernot C. Doppler echocardiographic measurement of low velocity motion of the left ventricular posterior wall. Am J Cardiol. 1989. 64:66–75.


13. Koffas H, Dukes-McEwan J, Corcoran BM, Moran CM, French A, Sboros V, Simpson K, McDicken WN. Pulsed tissue Doppler imaging in normal cats and cats with hypertrophic cardiomyopathy. J Vet Intern Med. 2006. 20:65–77.


14. Nagueh SF, Lakkis NM, Middleton KJ, Spencer WH 3rd, Zoghbi WA, Quiñones MA. Doppler estimation of left ventricular filling pressures in patients with hypertrophic cardiomyopathy. Circulation. 1999. 99:254–261.


15. Nagueh SF, Mikati I, Kopelen HA, Middleton KJ, Quiñones MA, Zoghbi WA. Doppler estimation of left ventricular filling pressure in sinus tachycardia. A new application of tissue Doppler imaging. Circulation. 1998. 98:1644–1650.


16. Oki T, Tabata T, Mishiro Y, Yamada H, Abe M, Onose Y, Wakatsuki T, Iuchi A, Ito S. Pulsed tissue Doppler imaging of left ventricular systolic and diastolic wall motion velocities to evaluate differences between long and short axes in healthy subjects. J Am Soc Echocardiogr. 1999. 12:308–313.


17. Oki T, Tabata T, Yamada H, Wakatsuki T, Shinohara H, Nishikado A, Iuchi A, Fukuda N, Ito S. Clinical application of pulsed Doppler tissue imaging for assessing abnormal left ventricular relaxation. Am J Cardiol. 1997. 79:921–928.


18. O'Sullivan ML, O'Grady MR, Minors SL. Assessment of diastolic function by Doppler echocardiography in normal Doberman Pinschers and Doberman Pinschers with dilated cardiomyopathy. J Vet Intern Med. 2007. 21:81–91.
19. Oyama MA, Sisson DD, Bulmer BJ, Constable PD. Echocardiographic estimation of mean left atrial pressure in a canine model of acute mitral valve insufficiency. J Vet Intern Med. 2004. 18:667–672.


20. Sohn DW, Chai IH, Lee DJ, Kim HC, Kim HS, Oh BH, Lee MM, Park YB, Choi YS, Seo JD, Lee YW. Assessment of mitral annulus velocity by Doppler tissue imaging in the evaluation of left ventricular diastolic function. J Am Coll Cardiol. 1997. 30:474–480.


21. Teshima K, Asano K, Sasaki Y, Kato Y, Kutara K, Edamura K, Hasegawa A, Tanaka S. Assessment of left ventricular function using pulsed tissue Doppler imaging in healthy dogs and dogs with spontaneous mitral regurgitation. J Vet Med Sci. 2005. 67:1207–1215.


22. Vinereanu D, Khokhar A, Fraser AG. Reproducibility of pulsed wave tissue Doppler echocardiography. J Am Soc Echocardiogr. 1999. 12:492–499.

