Abstract
A study of amoxicillin pharmacokinetics was conducted in healthy goats and goats with chronic lead intoxication. The intoxicated goats had increased serum concentrations of liver enzymes (alanine aminotransferase and γ-glutamyl transferase), blood urea nitrogen, and reactivated δ-aminolevulinic acid dehydratase compared to the controls. Following intravenous amoxicillin (10 mg/kg bw) in control and lead-intoxicated goats, elimination half-lives were 4.14 and 1.26 h, respectively. The volumes of distribution based on the terminal phase were 1.19 and 0.38 L/kg, respectively, and those at steady-state were 0.54 and 0.18 L/kg, respectively. After intramuscular (IM) amoxicillin (10 mg/kg bw) in lead-intoxicated goats and control animals, the absorption, distribution, and elimination of the drug were more rapid in lead-intoxicated goats than the controls. Peak serum concentrations of 21.89 and 12.19 µg/mL were achieved at 1 h and 2 h, respectively, in lead-intoxicated and control goats. Amoxicillin bioavailability in the lead-intoxicated goats decreased 20% compared to the controls. After amoxicillin, more of the drug was excreted in the urine from lead-intoxicated goats than the controls. Our results suggested that lead intoxication in goats increases the rate of amoxicillin absorption after IM administration and distribution and elimination. Thus, lead intoxication may impair the therapeutic effectiveness of amoxicillin.
Lead is a high-risk environmental pollutant and extensively used by industry. This metal is therefore widely distributed in the environment. Routes of lead exposure include air, water, soil, food, and consumer products. One of the largest sources is chipping lead-based paint that exists in many old buildings. Lead poisoning due to high intake of lead from contaminated mining areas or industrial pollution, is a serious problem. Lead pollution can reach levels exceeding maximum residual limits [14,19,20,23,24]. Lead poisoning has been reported in sheep. A study in Iran demonstrated that the lead concentration in sheep milk (8.1%) was higher than the Codes standard [22]. In a private farm located in Giza (Egypt), eight out of 50 Marino sheep were found to have lead toxicity after consuming plants polluted with lead [16]. These sheep showed high degrees of kidney and liver degeneration accompanied by elevated serum concentrations of aspartate aminotransferase (AST), alanine aminotransferase (ALT), urea, creatinine, cortisol, sodium, and potassium [18].
Amoxicillin is a semi-synthetic amino-penicillin β-lactam antibiotic that is effective against a wide range of Gram-positive and -negative bacteria. This drug is widely used in veterinary medicine because of its broad spectrum of antibacterial activity [9]. It is administered as a sodium salt that is highly soluble in water, and as a trihydrate salt that is less soluble in water. Amoxicillin can be administered orally and parenterally. This compound is bactericidal and its minimum inhibitory concentration (MIC) is about 0.25 µg/mL for most susceptible bacteria [5]. Amoxicillin is highly absorbed after oral administration and, eliminated predominantly via renal excretion by glomerular filtration and active tubular secretion [18]. The efficacy of amoxicillin is correlated with time above the MIC (time > MIC) of the susceptible microorganism [25]. Consequently, plasma concentrations above 0.25 µg/mL may be considered therapeutically effective. Pharmacokinetics (PK) and pharmacodynamics (PD) of a drug determine the therapeutic outcomes. The area under the plasma concentration-time curve (AUC), the maximum plasma concentration (Cmax), and the time to reach Cmax (tmax) are the major useful PK parameters whereas the MIC is the most useful PD parameter [1].
The effects of lead intoxication on amoxicillin PK have not been studied. We hypothesized that chronic lead intoxication may severely damage the liver and kidneys, thereby affecting the PK and subsequently altering the effectiveness of amoxicillin therapy. The specific aim of the present study was to evaluate the effects of chronic lead intoxication on amoxicillin PK in goats following a single intravenous (IV) and intramuscular (IM) administration of amoxicillin (10 mg/kg bw).
Ten clinically healthy 1-year-old female Boer goats weighing 15~27 kg were used in this investigation. These goats were procured from a local source in central Iowa. The animal protocol was approved by the Institutional Animal Care and Use Committee (IACUC) of Iowa State University, USA. The goats were allowed to acclimatize for 14 days prior to the study. The animal facility was maintained at 25 ± 2℃ and 45~65% humidity. All goats had free access to water and antibiotic-free feed from a local source.
Amoxicillin sodium was prepared by dissolving 69 mg of amoxicillin trihydrate (Research Products International, USA) equivalent to 60 mg amoxicillin base in 1 mL of 1 N NaOH. The solution was then neutralized with 1 mL of 1 N HCl, resulting in a final concentration of 30 mg amoxicillin base/mL with a pH of 7.1. The drug was sterilized by filtration with a 0.22-µm filter (Fisher Scientific, USA). Lead acetate (Fisher Scientific) was prepared by dissolving 18.308 g of lead acetate (Fisher Scientific) in 1 L of distilled water. This concentration of lead acetate was equivalent to 10 g lead base/L.
The 10 goats were randomly divided into two groups: the control group and the lead-intoxicated group. The lead-intoxicated group received 10 mg lead base/kg bw/day, orally for 10 weeks. Body weight was measured daily and the total daily lead dose given to the animals was adjusted accordingly. For the PK study, all goats were given a single dose of amoxicillin (10 mg/kg bw) by IV and IM administration. The study had a two-sequence, two-period randomized design with a washout period of 14 days between drug administration via the two routes. Amoxicillin was injected into the right jugular vein or neck for the IV and IM administration, respectively. Blood samples (5 mL) were collected using a 5-mL syringe (Beckton Dickinson & Company, USA) from the left jugular vein of each goat immediately before medication delivery and at 5, 10, 15, and 30 min as well as 1, 2, 4, 6, 8 and 12 h after amoxicillin administration. The blood samples were allowed to clot at room temperature and then centrifuged at 1,000 × g, 24℃ for 5 min. The resulting serum was stored at -20℃ until analysis.
On the day of the experiment, the urinary bladder was emptied via a balloon catheter (Foley latex vesical drainage balloon catheter; Cook Medical, USA) before amoxicillin administration. Urine samples were collected using a 20-mL syringe (Beckton Dickinson & Company) before medication delivery as well as 1, 2, 4, 6, 8, 12, and 24 h post-amoxicillin administration. The urinary samples were stored at -20℃ until analysis. At the end of each experiment, the urinary bladder was irrigated with 20~30 mL of 0.2 mg/mL potassium permanganate solution (Fisher Scientific) as an antiseptic agent. The volume of each urine sample was measured after each collection.
The blood δ-ALAD concentration was measured to determine the degree of lead intoxication according to a previously published method [12]. The enzymatic activity was expressed as µM porphobilinogen per h in whole blood at 37℃.
Serum concentrations of two liver enzymes (ALT and GGT) and BUN were measured using ALT, GGT, and BUN diagnostic reagents (Pointe Scientific, USA) to determine the extent of liver and kidney damage according to the method described by the manufacturer.
The measurement of serum protein concentrations is important because animals with liver and/or kidney damage can have low serum protein concentrations. Urine protein concentrations can serve an index for kidney dysfunction. One week before the PK study, a bicinchoninic acid assay was performed to measure urine and serum protein concentrations according to a previously published method [22].
Serum and urine amoxicillin concentrations were determined using high performance liquid chromatographic (HPLC). Previously published HPLC and amoxicillin extraction procedures [11,17] were modified for this study. Briefly, frozen serum or urine samples were thawed at room temperature, and 0.5 mL of each sample was mixed with 1 mL HPLC-grade methanol. Each sample was shaken in a vortex mixer for 15 sec, and then centrifuged at 1,000 × g at 24℃ for 10 min. The supernatant was filtered through a 0.22-µm syringe filter (Fisher Scientific) to remove the insoluble proteins. The clear filtrate was transferred into an auto sampler vial (Fisher Scientific) and 50 µL was injected into the HPLC system. The HPLC system consisted of a Beckman System Gold 126 solvent module, a Beckman model 508 autosampler, a Beckman model 168 detector (Beckman Coulter, USA), and a RP-C18 column (10 µm, 300 × 3.9 mm; Phenomenex, USA) with an isocratic mobile phase methanol : buffer (6 : 94). The buffer consisted of 0.1 M KH2PO4 adjusted to pH 3.2 with H3PO4. The mobile phase was filtered through a 0.22-µm membrane (Fisher Scientific) and degassed. The mobile phase was eluted at a flow rate of 1 mL/min and detected at a UV wavelength of 227 nm.
A standard calibration curve was prepared by adding 0.l mL of amoxicillin base (1 mg/mL) to 0.9 mL amoxicillin-free goat serum from these Boer goats or the phosphate buffer mentioned above. This solution was further diluted with the phosphate buffer mentioned above to produce standards containing 0.5, 1, 2.5, 5, 10, 25, 50, and 100 µg amoxicillin/mL. These standards underwent extraction and were analyzed in the same manner as unknown samples; these procedures are mentioned in the last paragraph. HPLC for amoxicillin analysis in goat serum and urine was validated by assessing linearity, precision, accuracy, and recovery. Two sets of quality control samples (5, 20, and 80 µg amoxicillin/mL) were prepared and analyzed at the beginning and end of each assay to determine the intra- and inter-assay coefficients of variation (CV).
PK analysis of the data was performed using a non-compartmental model as previously described [11] with commercially available software (WinNonlin software version 5.2; Pharsight Corporation, USA). The AUC was calculated using the trapezoidal rule with extrapolation to infinity. Cmax and tmax were determined by inspecting the individual drug serum concentration-time profiles. The slope of the terminal phase of the time-concentration curve was calculated by linear regression and converted to an elimination half-life (t1/2β) by multiplying the reciprocal by 0.693. The absolute bioavailability (F) was calculated using Equation 1:
Values for other PK parameters [total body clearance (ClB), volume of distribution based on the terminal phase (Vd(z)), and volume of distribution at steady-state (Vd(ss))] were calculated according to previously published equations [3]. A deconvolution analysis was performed using Phoenix WinNonlin, USA. For this analysis, the time-concentration profile was considered a composite or convolution of a disposition function [c(t)] and an input function [f(t)]. The disposition function was assumed to be a bi-exponential equation (Equation 2) for which the parameter values were calculated according to observed time-concentration data following IV administration in each animal.
α1 and α2 are the slopes; A1 and A2 are the y-intercepts of the distribution and elimination phases of the time-concentration curves, respectively.
Given this disposition function, parameter values for the input function [f(t)]: lag time (Tlag) and dispersion or smoothing factor (δ), drug input rates were calculated for each animal by iterative adjustment and comparison of calculated drug concentrations with time-concentration data measured following IM administration.
Data were expressed as mean ± the standard error (SE). Differences were analyzed using an analysis of variance. Mean comparisons were performed using Tukey's test. The p values < 0.05 were considered statistically significant. These calculations were performed using Prism 5.0 (GraphPad, USA).
All control goats were clinically healthy throughout the study period. The lead-intoxicated goats suffered from moderate excitation, ataxia, ~10% weight loss (compared to the control goats), and pale mucous membranes (anemia). One goat from the lead-intoxicated group died after 8 weeks of lead administration. Therefore, we had data from only four goats in the lead-intoxicated group.
The ratio between the reactivated and free δ-ALAD (R/F ratio) in lead-intoxicated goats was 0.14 before lead administration. This ratio increased over time to 3.95 and 4.74 at the sixth and tenth week, respectively, after lead administration (Fig. 1). In contrast, the R/F ratio of the control group reamined low (0.13~0.25) and steady throughout the 10-week period.
The serum concentrations of ALT, GGT, and BUN were significantly higher in the lead-intoxicated goats than the control animals during the 10-week lead treatment period (Fig. 2). These results were indicative of liver and kidney damage. Total urine protein concentrations at the ninth week of lead administration were 3 times higher in the lead-intoxicated goats than the controls (2.51 ± 0.01 gm/dL versus 0.81 ± 0.03 gm/dL, p < 0.05). In addition, the total serum protein concentrations at the ninth week of lead administration were significantly lower in the lead-intoxicated goats (6.67 ± 0.03 gm/dL) than in the control animals (7.47 ± 0.13 gm/dL), suggesting that the liver of the lead-intoxicated goats was unable to produce sufficient plasma proteins and urinary excretion of plasma protein was increased.
The calibration curves of amoxicillin were linear over the range of 0.5~100 µg/mL, and the correlation coefficients (r2) were > 0.999. The calculated limit of quantification was 0.5 µg/mL. The level of amoxicillin recovery calculated by comparing the peak height ratios for the serum samples and aqueous samples ranged from 80 to 85%. The inter-assay CV were 5, 4, and 5% while the intra-assay CV were 3, 3, and 8%, respectively, for amoxicillin concentrations of 5, 20 and 80 µg/mL.
The mean serum concentration-time profiles of amoxicillin (10 mg/kg bw) after a single IV administration in control and lead-intoxicated goats are shown in Fig. 3. The PK parameters are shown in Table 1. In the control goats, the serum concentration of amoxicillin was 78.9 ± 1.2 µg/mL 5 min post-administration. This concentration declined gradually to 0.9 ± 0.1 µg/mL at 8 h post-administration. Compared to the controls, the mean serum concentration of amoxicillin was significantly higher in lead-intoxicated goats during the first hour after lead administration and significantly lower at 4 h post-administration. Amoxicillin was slowly eliminated from the control goats with t1/2β of 4.14 ± 0.82 h compared to 1.26 ± 0.09 h for the lead-intoxicated goats (p < 0.05). The volume of distribution based on the terminal phase (Vd(z)) decreased by 70% in the lead-intoxicated goats. There was no significant difference in ClB between the two groups. After IV administration, amoxicillin was detectable for 8 h and 4 h in the urine of the control goats and lead-intoxicated goats, respectively (Table 2). The total amount of amoxicillin in the urine was 12% greater in the lead-intoxicated goats than the control animals. During the first hour post-administration, lead-intoxicated goats excreted 50% more amoxicillin than the controls.
The mean serum concentration-time profiles of amoxicillin after a single IM administration of 10 mg/kg bw in control and lead-intoxicated goats are shown in Fig. 4. The PK parameters are presented in Table 1. In the control goats, amoxicillin in the serum was detected 5 min post-administration. The maximum serum concentration (Cmax) was 12.2 ± 0.1 µg/mL at 2 h (tmax) post-administration and was still detectable until 8 h post-administration. In the lead-intoxicated goats, the Cmax was about 2 times higher than that of control goats (21.9 ± 1.7 µg/mL) at 1 h post-administration (tmax). However, serum amoxicillin concentrations were no longer measurable 6 h post-administration in the lead-intoxicated goats. Absolute bioavailability (F) of amoxicillin after IM administration decreased by 20% in lead-intoxicated goats compared to the control animals. After IM administration, amoxicillin was detected for 8 h and 4 h in the urine of control goats and lead-intoxicated goats, respectively (Table 2). Total amount of amoxicillin in the urine was 36% greater in the lead-intoxicated goats than the controls. In the IM amoxicillin experiment, the lead-intoxicated goats excreted 50% more amoxicillin into the urine than the controls 4 h post-administration.
Input rates and cumulative input calculated by the deconvolution analysis for the two experimental groups are presented in Fig. 5. The results showed that the lead-intoxicated animals had an earlier and higher peak, suggesting that amoxicillin was absorbed more rapidly in the lead-intoxicated animals than the controls. In addition, the δ value was significantly higher for the lead-intoxicated goats (0.50 ± 0.17) compared to the controls (0.05 ± 0.04; p = 0.02). No difference in lag time (Tlag) before absorption was observed between the two groups [0.008 ± 0.005 and 0.011 ± 0.003 h, respectively, for the control and lead intoxicated goats (p = 0.66)].
The extensive use of amoxicillin for treating various infections in animals and the problems caused by heavy metal intoxication in food-producing animals have necessitated the study of lead intoxication effects on amoxicillin PK in goats. In the present study, daily oral administration of lead acetate (10 mg lead base/kg bw) for 10 weeks in goats greatly increased the ratio of reactivated and free erythrocytic δ-ALAD. These results were indicative of chronic lead intoxication [13]. In the present investigation, marked increases in serum concentrations of ALT, GGT, and BUN were observed after 4 weeks of lead administration. These results indicated that both the liver and kidneys were damaged by chronic lead-intoxication. Serum and urine protein concentrations in lead-intoxicated goats also reflected liver and kidney damage in these animals; at week 9, the urinary protein levels were high while the serum protein concentrations were low. The high urinary protein level is attributable to chronic renal damage while the low serum protein level is probably caused by a combination of reduced hepatic protein synthesis and high urinary protein loss.
The liver and kidneys play a key role in drug metabolism and elimination. Therefore, damage of these organs may have a great impact on the PK and bioavailability of drugs. For example, liver and kidney damage in humans decreases the elimination rate and increases the AUC of selegiline, a monoamine oxidase inhibitor, by lowering cytochrome P-450 enzyme levels [2]. Because lead intoxication can damage both the liver and kidneys, we expected that drug PK would be affected in lead-intoxicated subjects. After an extensive literature search, we located only one relevant publication. In three adult human patients with chronic lead poisoning, renal clearance and metabolism of meso-2,3-dimercaptosuccinic acid (MDSA), a lead-chelating agent, were lower than those of healthy subjects [6]. The authors of this study attributed the low clearance from patients with lead poisoning to changes in the distribution/binding of MDSA to erythrocytes. To the best of our knowledge, the present study is the first to describe the PK of a drug in animals with chronic lead intoxication.
In the present investigation, lead-intoxicated goats had a higher Cmax, but lower Vd, t1/2β, mean residence time, and bioavailability than control goats after parenteral administration of amoxicillin. However, no difference in amoxicillin clearance time was found between the two groups of goats in the IV amoxicillin experiment. This occurred despite the fact that the lead-intoxicated goats showed signs of kidney damage as indicated by higher BUN and urinary protein concentrations along with lower serum protein levels compared to the controls.
Following IM administration of amoxicillin, serum concentrations of this drug reached a maximum faster and higher in the lead-intoxicated goats than the controls, suggesting an increase in the amoxicilln absorption from the IM injection site in the lead-intoxicated animals. Lead intoxication can have a significant impact on the cardiovascular system by inducing vasodilatation and endothelial dysfunction due to the overproduction of nitric oxide [10,26]. This could promote drug absorption via vasodilatation, particularly in the capillaries. The deconvolution analysis allowed us to determine whether the differences between the control and lead-intoxicated goats observed in the IM amoxicillin experiment were attributable to differences in drug absorption and/or disposition. Since the results of the deconvolution analysis showed that the lead-intoxicated goats had a faster input rate and faster dispersion (higher δ value) than the controls, we concluded that the difference in serum amoxicillin concentrations after IM administration is due to both drug absorption and disposition. The effects of lead intoxication on drug absorption still warrant further studies.
Vd values for the lead-intoxicated goats were lower than those for the controls, indicating low distribution of amoxicillin in tissues of the lead-intoxicated animals. After IM administration, amoxicillin bioavailability was lower in the lead-intoxicated goats than the controls. These results suggest that decreased drug bioavailability after IM administration is attributable to decreased Vd.
As previously stated, we hypothesized that liver and kidney damage associated with lead-intoxication may be responsible for changes in the PK of amoxicillin. Therefore, we searched for literature regarding the effects of liver and kidney damage on amoxicillin PK. Bhar et al. [4] performed a study in which amoxicillin was orally administered to chickens with acute hepatopathy induced by acetaminophen given for 7 days and acute nephropathy induced by uranyl nitrate taken for 4 days. Persistently higher plasma concentrations of amoxicillin were seen after oral administration in chickens with liver and kidney damage compared to the healthy birds. Additionally, chickens with liver and kidney damage had higher t1/2β values and lower ClB values than healthy birds. These results are different from our findings in goats. This is probably due to 1) the acute nephropathic conditions of the chickens and 2) species-specific differences. In mammals, most amoxicillin is excreted unchanged in the urine [21], but it is unknown whether this occurs in chickens. Because most amoxicillin in mammals is excreted as the parent compound, the effect of lead-induced liver damage on changes of amoxicillin PK in lead-intoxicated animals would be diminished.
Lashev and Fink-Gremels [13] conducted a study in which amoxicillin trihydrate was orally administered to albino rats with experimentally injured kidneys (by IV potassium chromate) and liver (by IP tetrachloromethane). They showed that impairment of these organs for 4~6 days increases the AUC and t1/2β of amoxicillin, and speculated that lower cytochrome P-450 levels in rats with liver or kidney damage causes these changes. It is interesting that our findings concerning the PK of amoxicillin were different from those of the previous studies in chickens [4] and rats [14], and lead-intoxicated goats had lower Vd and t1/2β values than control animals after both IV and IM amoxicillin administration. However, the lead-intoxicated goats did not show significant changes in drug clearance after IV administration of amoxicillin. Therefore, decreased Vd of amoxicillin could be the primary change in lead-intoxicated animals.
Because penicillins have a structure similar to that of penicillamine (an excellent lead chelator), we hypothesize that amoxicillin might have chelated lead in the goats. This may have resulted in the formation of a lead-amoxicillin complex similar to lead-penicillamine complexes. Such a complex might persist in the circulation and would thus decrease Vd without affecting drug clearance. Unfortunately, we have not found any reports demonstrating the lead-chelating activity of penicillins. The possible formation of lead-amoxicillin complexes needs to be tested in future studies.
PK/PD relationships between AUC : MIC, Cmax : MIC, and time above MIC ratios during which plasma/serum concentrations exceed the MIC have been particularly useful for optimizing drug efficacy to minimize bacterial resistance [15]. Accordingly, antimicrobial agents can be classified as concentration-dependent, time-dependent, or co-dependent [1]. The efficacy of amoxicillin is thought to be correlated with the time > MIC [25]. Based on the serum concentration-time profile of amoxicillin after IM administration, the drug concentrations were above the MIC for most susceptible microorganisms (MIC = 0.25 µg/mL) for 4 and 8 h in lead-intoxicated and control goats, respectively. The effect of amoxicillin is time-dependent, which means that efficacy requires maintaining drug concentrations above the MIC for prolonged periods of time [7]. Achieving drug concentrations that are higher than MIC does not necessarily improve efficacy, especially if these concentrations fall rapidly to levels that are below the MIC. When comparing lead-intoxicated goats to the control animals, Cmax of amoxicillin was twice as high in the lead-intoxicated goats, but fell below the MIC more rapidly. This suggests that amoxicillin will be less effective in lead-intoxicated animals.
Amoxicillin PK in the control (healthy) goats in the present study was different from that reported in a previous study. Elsheikh et al. [9] found that the AUC, Vd, and t1/2β in goats were 31 µg.h/mL, 0.39 L/kg bw, and 1.02 h, respectively, after IV administration of 10 mg/kg bwamoxicillin. These values are different from our data (43 µg.h/mL, 0.54 L/kg bw, and 4.14 h, respectively). These variations are probably due to the fact that we used HPLC to analyze amoxicillin whereas Elsheikh et al. [8] used a microbial plate assay.
In conclusion, the findings from the present study indicated that chronic lead intoxication in goats resulted in a shorter and more rapid disposition of amoxicillin compared to normal animals. Thus, lead intoxication may impair the therapeutic efficacy of this drug. Goats with chronic lead intoxication would therefore need more frequent amoxicillin administration for effective antibiotic therapy.
Figures and Tables
Fig. 1
Ratio (R/F) of the reactivated and free δ-aminolevulinic acid dehydratase (δ-ALAD) in the blood of control and lead-intoxicated goats. The four lead-intoxicated goats received 10 mg/kg body weight/day of lead for 10 weeks. Serum δ-ALAD activity was measured every 2 weeks for 10 weeks. Another five goats served as controls. The R/F ratio significantly increased over time in the lead-intoxicated goats by the addition of glutathione that reactivated the enzyme through chelation. Values are presented as the mean ± SE. *p < 0.05 vs. the control at the corresponding time point.
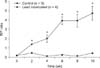
Fig. 2
Changes in serum concentrations of alanine aminotransferase (ALT; A), γ-glutamyl transferase (GGT; B), and blood urea nitrogen (BUN; C) in control and lead-intoxicated goats. The four lead-intoxicated goats received 10 mg/kg bw/day of lead for 10 weeks. The serum ALT, GGT, and BUN concentrations were measured every 2 weeks for 10 weeks. Another five goats served as controls. A significant increase in serum concentrations of ALT, GGT, and BUN in the lead-intoxicated goats indicated damage to the liver and kidney. Values are expressed as the mean ± SE. *p < 0.05 vs. the control at the corresponding time points.
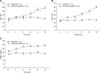
Fig. 3
The serum concentration-time profile of amoxicillin (10 mg/kg bw) after IV administration in control and lead-intoxicated goats. The four lead-intoxicated goats received 10 mg/kg bw/day of lead for 10 weeks. Another five goats served as controls. Amoxicillin was administered after 10 weeks of lead administration. Data are presented as the mean ± SE. *p < 0.05 vs. the control at the corresponding time point.
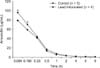
Fig. 4
The serum concentration-time profile of amoxicillin (10 mg/kg bw) after IM administration in control and lead-intoxicated goats. The four lead-intoxicated goats received 10 mg/kg bw/day of lead for 10 weeks. Another five goats served as controls. Amoxicillin was administered IM 12 weeks after the onset of lead administration (2 weeks after the IV amoxicillin experiment). Values are presented as the mean ± SE. *p < 0.05 vs. the control at the corresponding time points.
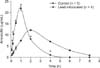
Fig. 5
Average input rates of amoxicillin and cumulative input for lead-intoxicated (solid line) and control (dashed line) goats. (A) Average input rates and (B) average cumulative input over time. These data were calculated based on extravascular data of the IM amoxicillin experiment with the deconvolution analysis. More rapid absorption and disposition of the amoxicillin was observed in the lead-intoxicated animals compared to the controls.
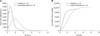
Table 1
Pharmacokinetics (PK) parameters of amoxicillin (10 mg/kg bw)* in control and lead-intoxicated goats after intravenous (IV) and intramuscular (IM) administration
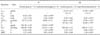
Data are presented as the mean ± SE. *p < 0.05. Cmax: maximal concentration, tmax: when the maximal serum concentration is reached, Vd(ss): volume of distribution at steady-state, Vdz: volume of distribution based on the terminal phase, AUC0-t: area under the plasma concentration-time curve, F: bioavailability, C0: initial concentration, ClB: total body clearance, t1/2β: elimination half-life, MRT: mean residence time.
References
1. Abu-Basha EA, Gehring R, Albwa'neh SJ. Pharmacokinetics and bioavailability of spectinomycin after i.v., i.m., s.c. and oral administration in broiler chickens. J Vet Pharmacol Ther. 2007; 30:139–144.


2. Anttila M, Sotaniemi EA, Pelkonen O, Rautio A. Marked effect of liver and kidney function on the pharmacokinetics of selegiline. Clin Pharmacol Ther. 2005; 77:54–62.


3. Baggot JD. Principles of Drug Disposition in Domestic Animals: The basis of Veterinary Clinical Pharmacology. Philadelphia: WB Saunders;1977. p. 168–179.
4. Bhar MK, Datta BK, Patra PH, Dash JR, Sar TK, Chakraborty AK, Mandal TK. Disposition kinetics of amoxicillin in healthy, hepatopathic and nephropathic conditions in chicken after single oral administration. Vet Res Forum. 2010; 1:150–156.
5. Brander GC, Paugh DM, Bywater RJ, Jenkins WL. Veterinary Applied Pharmacology and Therapeutics. 5th ed. London: Bailliere Tindall;1991. p. 436–437.
6. Dart RC, Hurlbut KM, Maiorino RM, Mayersohn M, Aposhian HV, Hassen LVB. Pharmacokinetics of meso-2,3-dimercaptosuccinic acid in patients with lead poisoning and in healthy adults. J Pediatr. 1994; 125:309–316.


7. Delis GA, Koutsoviti-Papadopoulou M, Siarkou VI, Kounenis G, Batzias GC. Pharmacodynamics of amoxicillin against Mannheimia haemolytica and Pasteurella multocida and pharmacokinetic/pharmacodynamic (PK/PD) correlation in sheep. Res Vet Sci. 2010; 89:418–425.


8. Elsheikh HA, Taha AA, Khalafalla AE, Osman IA, Wasfi IA. Pharmacokinetics of amoxicillin trihydrate in Desert sheep and Nubian goats. Vet Res Commun. 1999; 23:507–514.
9. Errecalde JO, Carmely D, Mariño EL, Mestorino N. Pharmacokinetics of amoxicillin in normal horses and horses with experimental arthritis. J Vet Pharmacol Ther. 2001; 24:1–6.


10. Fiorim J, Ribeiro Júnior RF, Silveira EA, Padilha AS, Vescovi MVA, de Jesus HC, Stefanon I, Salaices M, Vassallo DV. Low-level lead exposure increases systolic arterial pressure and endothelium-derived vasodilator factors in rat aortas. PLoS One. 2011; 6:e17117.


11. Foulstone M, Reading C. Assay of amoxicillin and clavulanic acid, the components of Augmentin, in biological fluids with high-performance liquid chromatography. Antimicrob Agents Chemother. 1982; 22:753–762.


12. Haas T, Mache W, Schaller KH, Mache K, Klavis G, Stumpf R. The determination of delta-aminolaevulinic acid dehydratase activity and its diagnostic value. Int Arch Arbeitsmed. 1972; 30:87–104.
13. Lashev L, Fink-Gremels J. Changes in amoxicillin pharmacokinetics in white rats with experimentally damaged kidneys and liver. Vet Med Nauki. 1987; 24:57–63.
14. Liu ZP. Lead poisoning combined with cadmium in sheep and horses in the vicinity of non-ferrous metal smelters. Sci Total Environ. 2003; 309:117–126.


15. McKellar QA, Sanchez Bruni SF, Jones DG. Pharmacokinetic/pharmacodynamic relationships of antimicrobial drugs used in veterinary medicine. J Vet Pharmacol Ther. 2004; 27:503–514.


16. Zaki MS, Mostafa S, Awad I. Some studies on lead toxicity in Marino sheep. J Am Sci. 2010; 6:128–131.
17. Pires de Abreu LR, Ortiz RAM, de Castro SC, Pedrazzoli J. HPLC determination of amoxicillin comparative bioavailability in healthy volunteers after a single dose administration. J Pharm Pharm Sci. 2003; 6:223–230.
18. Plumb DC. Veterinary Drug Handbook. 4th ed. Ames: Iowa State Press;2002. p. 42–45.
19. Rahimi E. Lead and cadmium concentrations in goat, cow, sheep, and buffalo milks from different regions of Iran. Food Chem. 2013; 136:389–391.


20. Rodríguez-Estival J, Barasona JA, Mateo R. Blood Pb and δ-ALAD inhibition in cattle and sheep from a Pb-polluted mining area. Environ Pollut. 2012; 160:118–124.


21. Sarmah AK, Meyer MT, Boxall ABA. A global perspective on the use, sales, exposure pathways, occurrence, fate and effects of veterinary antibiotics (VAs) in the environment. Chemosphere. 2006; 65:725–759.


22. Smith PK, Krohn RI, Hermanson GT, Mallia AK, Gartner FH, Provenzano MD, Fujimoto EK, Goeke NM, Olson BJ, Klenk DC. Measurement of protein using bicinchoninic acid. Anal Biochem. 1985; 150:76–85.


23. Smith KM, Abrahams PW, Dagleish MP, Steigmajer J. The intake of lead and associated metals by sheep grazing mining-contaminated floodplain pastures in mid-Wales, UK: I. Soil ingestion, soil-metal partitioning and potential availability to pasture herbage and livestock. Sci Total Environ. 2009; 407:3731–3739.

