Abstract
The effects of black rice anthocyanidins (BRACs) on retinal damage induced by photochemical stress are not well known. In the present study, Sprague-Dawley rats were fed AIN-93M for 1 week, after which 80 rats were randomly divided into two groups and treated with (n = 40) or without BRACs (n = 40) for 15 days, respectively. After treatment, both groups were exposed to fluorescent light (3,000 ± 200 lux; 25℃), and the protective effect of dietary BRACs were evaluated afterwards. Our results showed that dietary BRACs effectively prevented retinal photochemical damage and inhibited the retinal cells apoptosis induced by fluorescent light (p < 0.05). Moreover, dietary BRACs inhibited expression of AP-1 (c-fos/c-jun subunits), up-regulated NF-κB (p65) expression and phosphorylation of IκB-α, and decreased Caspase-1 expression (p < 0.05). These results suggest that BRACs improve retinal damage produced by photochemical stress in rats via AP-1/NF-κB/Caspase-1 apoptotic mechanisms.
The retina is vulnerable to damage by light that occurs via thermal, mechanical, and photochemical effects [25,35]. Photochemical damage is known to be particularly prevalent because it can cause retinal damage within the intensity range of natural light. Photochemical lesions result in reduced visual function and disintegration of the retinal outer/inner segment (ROS/RIS). In this process, after the photoreceptors degenerate, the outer nuclear layer (ONL) becomes thinner, and retinal function may eventually be totally lost, leading to blindness [19,25]. Accordingly, it is particularly important to study protection against retinal photochemical damage through extensive and intensive research.
It has been suggested that the primary cause of retinal damage induced by photochemical exposure is photoreceptor cells apoptosis, but the specific mechanisms of retinal cells apoptosis induced by photochemical stress are still unclear [1,13]. The general view regarding retinal photochemical damage (RPD) is that photons are initially absorbed by chromophores (melanin and lipofuscin), rhodopsin, and retinoids. After a number of free radicals are generated, lipid peroxidation and reactive oxygen intermediates are produced via oxidizing reactions [8,10,11,34]. Next, the molecullar signals involved with apoptosis undergo a reaction, with increasing activator protein-1 (AP-1) activation and over-expression of caspase-1 regulated by reduction of nuclear factor-kappa B (NF-κB) transcription. These molecules then combine with DNA at the corresponding position, resulting in the death signal being transduced into the nucleus [7,16,18,33,37]. Finally, DNA fragmentation dependent on the caspase (Caspase-1) apoptotic pathways [10,11,18,37] and non-caspase apoptotic pathways such as LEI/L-DNase II [4] induce photoreceptor cell apoptosis.
Anthocyanidins of the compound naturally occur in six different monomers that can be converted into anthocyanins by combining with glycosyl and play a role as anthocyanins in organs such as the retina, brain and liver [21,24]. Anthocyanins exhibit high bioactivities including antioxidant, anti-inflammatory and cancer preventative activities [15,17].
Laboratory studies have suggested that Vitamin E, taurine, N-acetylcysteine and thiourea protect against retinal photochemical damage, but there were no well-pleasing effects [26,27,29,40]. It was recently reported that anthocyanidins exert protective effects against retinal injury in murine photoreceptor cells (661W) and retinal pigment epithelial cells [14,20,31], and that anthocyanidins can inhibit the apoptosis of retinal neuronal cells [22]. However, the specific cellular signaling pathways of anthocyanidins induced protection are currently unclear.
Based on the information presented above, it is known that anthocyanidins exert a protective effect against retinal damage induced by light, that retinal cell apoptosis primarily leads to RPD, and that molecular signals of AP-1, NF-κB and Caspase-1 regulate the process of retinal cells injury induced by apoptosis. Consequently, it can be assumed that anthocyanidins protect the retina against RPD through the apoptotic pathway of AP-1, NF-κB and Caspase-1. To test this assumption, an in vivo model of RPD was established in Sprague-Dawley rats and c-jun, c-fos, p65, and IκB-α [6,32], which induce NF-κB activity when phosphorylated and are known inhibitors of NF-κB, were measured by western blot and qRT-PCR to investigate the mechanism through which anthocyanidins induce protection to understand the role of AP-1, NF-κB and Caspase-1.
BRACs were kindly provided by Wenhua Ling MD, PhD (University of Sun-Yet, China). To extract the BRACs, the black rice pigmented fraction was removed from whole rice and then extracted with 60% ethanol containing 0.1% HCl. All extracts were concentrated using a rotary evaporator until all alcoholic residues were removed, after which they were partitioned against petroleum ether. The aqueous extract was then purified with an Amberlite XAD-7 column. The eluted anthocyadins fraction was concentrated, and the aqueous residue was lyophilized. Characterization and quantification of anthocyanins present in the extract were conducted by HPLC followed by further LC-MS analysis [5]. Anthocyanins were identified by both retention time and mass profile in comparison with authentic standards. Two major anthocyanins have been extracted and identified from black rice, cyanidin-3-glucoside and peonidin-3-glucoside. The total anthocyanins content was 27.4% in the extract, among which cyanidin-3-glucoside accounted for 25.7% and peonidin-3-glucoside for 1.7%. These are similar to the conclusions of Xia XD et al. [39].
Sprague-Dawley rats (n = 100) aged 10 wks weighing 350 ± 20 g were housed in standard stainless steel cages at 25℃. All procedures were conducted in accordance with the approved protocol for experimental animals set by the standing committee on animal care at the Chungdu Medical College. After consuming a purified diet based on the AIN-93M formulation [28] for 1 week, eighty rats were randomly divided into two groups and treated with BRACs (1 g of BRACs in 100 g diet, n = 40) or without BRACs (n = 40) for 15 days. Following treatment, these two groups were exposed to light for 0, 3, 6, 12, or 24 h in an illumination chamber that transmitted a fluorescent light at an illuminance level of 3,000 ± 200 lux. Sixteen fluorescent lamps were mounted vertically and evenly along the four sides of the chamber, and the chamber temperature during the light exposure was 25℃. Another twenty rats were fed according to the AIN-93M formulation and maintained in the dark for 48 h without light exposure as a control (n = 20). After treatment, all rats were weighed and then sacrificed by decapitation under anesthesia. One eye was harvested for biochemical measurements and the other was collected for morphology, western blot, immunohistochemistry, qRT-PCR, or TUNEL assay.
The eyes were enucleated and fixed. After dehydration in a series of graded ethanol, the specimens were embedded in Luveak 812 Ultrathin. Sections were then made with a Porter-Blum MT2 microtome (Sorvall, USA) and examined with a Hitachi H300 (Hitachi, Japan) electron microscope.
Retinal physiological function was assessed by dark-adapted electroretinography (ERG) as previously described [9]. ERGs were recorded with a system developed by the US EPA and the amplitudes of the a- and b-waves of ERG were analyzed.
The frozen sections of retinas were used for an apoptosis assay with a TUNEL assay kit (Apop Tag; Oncor, USA). The apoptotic index (AI), expressed as a percentage, was calculated by dividing the number of TUNEL-positive photoreceptor cells by the total number of photoreceptor cells in the section as seen under a light microscope.
Total proteins of retinas were denatured and resolved by 12% SDS-polyacrylamide gel electrophoresis. After transfer of the protein from the gel to a polyvinylidene difluoride (PVDF) membrane, the membrane was saturated in PBS-Tween plus 5% milk and incubated with anti-phospho-IκB-α, anti-AP-1 (c-fos/c-jun), anti-NF-κB (p65) and anti-Caspase-1 (1 : 2,000 dilution; Santa Cruz Biotechnology, USA) at 4℃ overnight, followed by a horseradish peroxidase-linked secondary antibody (1 : 1,000 dilution). Specific protein bands were revealed by enhanced chemiluminescence and scanned using a gel imaging analytical system (BioRad Laboratories, USA).
For analysis of Caspase-1, AP-1 (c-fos/c-jun), and NF-κB (p65) gene expression, a quantitative real-time PCR (qRT-PCR) method was used as described in our previous study [38]. Oligonucleotide primers and TaqMan probes were designed using the Primer Express software 2.0 (Applied Biosystems, USA) and synthesized by Takara Biotechnology. The primer and probe sequences used were as follows: for the Caspase-1 gene, forward 5'- TGG ATT GCT GGA TGA ACT TTT AGA-3', reverse 5'- GCA CAG GTC TCG TGC CTT TT-3', and probe 5'-FAM- CAA GAC TTC TGA CAG TAC CTT CCT TGT ATT CAT GTC TCA TGG TCT CCA GGA GGG AAT ATG TG-TAMRA-3'; for the c-fos gene, forward 5'- GGA GGT CTG CCT GAG GCT TC-3', reverse 5'-CAC GTT GCT GAT GCT CTT GAC-3', and 5'-FAM- GAT GAC TTC TTG TTT CCG GCA TCA TCT AGG CCC AGT GGC TCG GAG ACT GC-TAMRA-3'; for the c-jun gene, forward 5'- GAC GGA CCG TTC TAT GAC TGC-3', reverse 5'- GGA GGA ACG AGG CGT TGA-3', and probe 5'-FAM- GGT ATT TCT CTT TTG ACA CAG CTG TGT CAA AAG AGA AAT ACC TTT TT-TAMRA-3'; for the p65 gene, forward 5'- AGG CTT CTG GGC CAT ATG TG-3', reverse 5'- TGT GCT TCT CTC CCC-3', and probe 5'-FAM- GCC CCC ATG AGG TCT GCC TGA CAC CAG CAT TTG AGG GGC CAC TTT TCT GT-TAMRA-3'; for the GAPDH, forward 5'- AAG TGA AGC AGG AGG GTG GAA -3', reverse 5'- CAG CCT CAC CCC ATT TGA TG -3', and probe 5'-FAM-TGC CGC CTG GAG AAA CCT GCC-TAMRA-3'. Total RNA was extracted from the retinas using TRIzol reagent according to the protocol provided by the manufacturer (Invitrogen, USA). Quantitative real-time TaqMan PCR analysis was performed according to the manufacturer's instructions (TaqMan Gold RT-PCR Kit protocol; Applied Biosystems) with an ABI Prism 7000 TaqMan real-time fluorescent thermal cycler (PerkinElmer Life Sciences, USA). The thermal cycling conditions consisted of 40 cycles of 2 min at 93℃, 1 min at 93℃, and 1 min at 55℃. Levels of the different mRNAs were subsequently normalized to GAPDH mRNA levels.
Results are given as the means and standard deviation. The weight, apoptotic index, mRNA expression and relative protein expression of rats treated with or without BRACs and exposed to light were compared by a Student's t test. Comparisons of the ERG components and light exposure were evaluated by Ryan's multiple range test when significant differences were detected by one-way ANOVA, and the differences in the same dietary rats exposed to light for different lengths of time were analyzed by Ryan's multiple range test (Stat-Light; Yukmus, Japan). All differences were considered statistically significant at p < 0.05.
There were atypical a- and b-waves of ERG with decreasing amplitude in rats exposed to light. In contrast, the a- and b-waves of ERGs in rats treated with BRACs were typical. As shown in Table 1, the amplitudes of the a- and b-waves in rats treated with BRACswere increased relative to those of rats exposed to light and not treated with BRACs (p < 0.05). There were no differences in the components of ERGs between normal rats and rats treated with BRACs.
We investigated whether BRACs decreased light-induced apoptosis in the retina. The value of labeled nuclei was detected by TUNEL assay. A number of apoptotic cells were found in the retinas of rats exposed to light, but few apoptotic cells were found in the retinas of rats treated with BRACs. As shown in Fig. 1, the apoptotic index (AI) increased gradually with exposure time. The AI was lower in the retinas of rats treated with BRACs than in rats not treated with BRACs after exposure for 3 h (p < 0.05).
As shown in Fig. 2A, there was an increase of Caspase-1 mRNA expression in the retinas of rats exposed to light at 3 h and 6 h, and a lower Caspase-1 mRNA expression in the retinas of rats treated with BRACs than in those not treated with BRACs (p < 0.05). Fig. 2B shows the reduction of Caspase-1 protein expression in the retinas of rats treated with BRACs relative to those not treated with BRACs. Dietary BRACs down-regulated the increased expression of Caspase-1 induced by light.
As shown in Fig. 3A, the expression of p65 mRNA increased transiently after exposure for 3 h and subsequently decreased in the retinas of rats exposed to light. However, p65 mRNA expression was initially lower (3 h) and higher in the retinas of rats treated with BRACs than in rats not treated with BRACs (p < 0.05). As shown in Fig. 3B, the expression of p65 protein in the retinas of rats not treated with BRACs was raised after exposure, peaked at 6 h, and declined afterwards. The expression was obviously higher (12 h and 24 h; p < 0.05) in the retinas of rats treated with BRACs than in those not treated with BRACs. Fig. 4 shows that the phosphorylation of IκB-α protein was higher in the retinas of rats treated with BRACs than those of rats not treated with BRACs at 12 h and 24 h (p < 0.05).
As shown in Fig. 5A, there was a high level of c-fos mRNA expression in the retinas of rats exposed to light at 3 h, but expression of c-fos mRNA was lower in the retinas of rats treated with BRACs than in rats not treated with BRACs at the same time point (p < 0.05). Fig. 5B shows the analogous result of c-fos protein expression detected by western blot analysis. As shown in Fig. 6A, the expression of c-jun mRNA was increased in the retinas of rats after exposure for 3 h. There was a decrease in the expression of c-jun mRNA in the retinas of rats treated with BRACs when compared with rats not treated with BRACs after exposure for 3 h (p < 0.05). As shown in Fig. 6B, the c-jun protein expression was lower in the retinas of rats treated with BRACs than in rats not treated with BRACs (p < 0.05). Dietary BRACs could partially decrease c-fos and c-jun expression in the retinas of rats exposed to light.
In the current investigation, we demonstrated that BRACs provide a protective effect against RPD in Sprague-Dawley rats and that BRACs improve the RPD by inhibition of photoreceptor cells apoptosis, which is associated with the AP-1/NF-κB/Caspase-1 apoptotic pathway.
Anthocyanidins are water-soluble plant pigments that play a role in organs via conversion into anthocyanins [21,24]. Anthocyanins and anthocyanidins have been subjected to extensive mechanistic studies and suggested to engage an array of protective effects toward retinal cells such as inhibition of apoptosis and suppression of activities of oncogenic transcription factors and protein tyrosine kinases [15,17,20,31].
Rats may be influenced by stress during the experimental period; however, our observations revealed no such abnormalities during the experiment. All rats gained weight normally and there were no abnormal secretions.
Our previous study demonstrated that BRACs suppressed morphological and functional damage to retinal cells produced by photochemical stimulus in vivo. Morphological analysis showed that light exposure for 24 h caused varying degrees of structural damage to retinas in the without BRACs group. In contrast, the retinas of rats treated with BRACs had relatively high integrity, and there was no obvious damage observed (data not shown). Moreover, ERG data revealed a decrease in a- and b-wave amplitude induced by BRACs, demonstrating that BRACs improved the retinal function (Table 1).
Retinal cell apoptosis is known to occur in response to photic injury, and apoptosis not only participates in the morphogenesis and tissue reconstruction of the retina, but also in retinopathy [8,16]. Photoreceptor cell loss may occur due to necrosis [3] or apoptosis caused by 2,500~3,500 lux high intensity light [12,18,33,37]. In this study, TUNEL analysis suggested that the latter was occurring. However, we also demonstrated that anthocyanidins decrease the apoptosis of photoreceptors induced by photochemical stress (Fig. 1), which is in accordance with the opinion that anthocyanidins afford an anti-apoptotic effect [22].
The classical mechanism of RPD is that photoreceptor cells apoptosis is regulated by reduction of NF-κB activation and increased AP-1 and Caspase-1 [7,18,33,37]. In this study, we confirmed that the apoptosis of photoreceptor cells was also blocked by interdiction of Caspase-1 with siRNA (data is not shown), which supported the finding that Caspase-1 is the main regulatory protease in the process of photoreceptor cells apoptosis by photochemical stress. The Caspases are a family of cysteine proteases that are indispensable to mammalian apoptosis. Caspase-1 has been identified as a central player in neuronal cell apoptosis [30,33]. Caspase-1 is regulated by NF-κB, and its over-expression can induce apoptosis in vivo and in vitro [18,37]. Furthermore, Caspase-1 activation is related to retinal photoreceptor apoptosis caused by light [10,11]. In this study, we observed that anthocyanidins inhibited the increased expression of Caspase-1 mRNA and protein induced by light in the retina (Fig. 2). These findings strongly suggested that anthocyanidins inhibited the expression of Caspase-1, which is involved in photoreceptor apoptosis produced by photochemical stress, and that BRACs blocked the RPD via reducing the Caspase-1.
Transcription factor NF-κB acts as a master regulator of stress responses by exerting a strong modulatory effect on apoptosis [2,23]. Our results indicated that continuous light exposure led to an early increase of NF-κB (p65) mRNA expression at 3 h and increased expression of NF-κB (p65) protein at 6 h, which subsequently decreased. This difference may have been because the early increase is due to an emergency response or constitutive expression and the subsequent decrease is in response to photochemical oxidative stress, which is supported by the results of a previous study [36]. We also found that anthocyanidins up-regulated NF-κB expression (Fig. 3) and phosphorylation of IκB-α, which induces NF-κB activity (Fig. 4). The experimental evidence demonstrated that NF-κB also played a significant role in protection against retinal photochemical injury via the supply of anthocyanidins.
We found that BRACs further decreased the expression of AP-1 (c-fos/c-jun) protein (Figs. 5 and 6), and that c-fos/c-jun mRNA expression was up-regulated in the retinas of rats exposed to light, but that this increase of c-fos/c-jun mRNA was restrained by anthocyanidins (Figs. 5A and 6B). Expression of AP-1 is associated with the apoptosis of photoreceptors induced by photochemical stress [12,37]. However, the detailed mechanism of this action has yet to be elucidated. The results of this study indicated that BRACs blocks the photoreceptor apoptotic pathway through reducing the AP-1 complex formation. Herein, AP-1 was found to play an important role in protection against retinal photochemical damage induced by anthocyanidins.
The results of this study suggest that BRACs could offer protection against retinal photochemical damage, implying that anthocyanidins have the potential for use in the treatment of retinal photochemical injury. However, there are many differences in the biochemical characteristics of human and rat eyes; accordingly, it is not clear if humans will benefit from anthocyanidins. Additionally, anthocyanidins are prone to avid chemical decomposition, the stability of anthocyanidins in plasma and tissues has not been clearly defined, and they only survive for minutes in the biophase. Furthermore, the interconversion between isomers of anthocyanins and anthocyanidins depends on pH, temperature and access to light. These pharmaceutical issues will strongly influence whether anthocyanidins are developed as clinical drugs.
In conclusion, our study revealed that retinal photochemical damage is regulated by AP-1, NF-κB and the Caspase-1 apoptotic pathway and that BRACs prevented RPD via inhibition of photoreceptor cells apoptosis. However, further studies are necessary to examine the mechanism through which anthocyanidins mediate a protective effect against retinal photochemical damage.
Figures and Tables
Fig. 1
Apoptotic index of the retina of rats treated with (-▪-) or without (-♦-) 1% BRACs for 15 days and exposed to light for 0~24 h. Values are the means for seven rats at each time point, with standard deviations represented by vertical bars. *Mean value was significantly different compared with the same light exposure time in rats not treated with BRACs (p < 0.05, t test). a,b,cMean values with different letters on the same curve at different exposure times were significantly different (p < 0.05, Ryan's multiple range test).
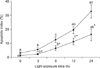
Fig. 2
The relative Caspase-1 (A) mRNA expression normalized for corresponding GAPDH levels in retinas of rats treated with (-▪-) or without (-♦-) 1% BRACs for 15 days and exposed to light for 0~24 h. The semi-quantitative densitometry of Caspase-1 (B) protein expressions normalized for 0 h light levels without BRACs (set as 1) in retinas of rats treated with (-▪-) or without (-□-) 1% BRACs for 15 days and exposed to light for 0 ~ 24 h. Values are the means for three determinations with their standard deviations shown by vertical bars for each time point. a,b,cMean values with unlike letters on the same curve or among the same diet group at different exposure times were significantly different by Ryan's multiple range test (p < 0.05). *Mean value was significantly different from that for rats at the same light exposure time but not treated with BRACs (p < 0.05, t test).
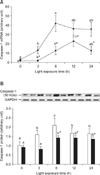
Fig. 3
The relative NF-κB, p65, (A) mRNA expressions normalized for corresponding GAPDH levels in retinas of rats treated with (-▪-) or without (-♦-) 1% BRACs for 15 days and exposed to light for 0 ~ 24 h. The semi-quantitative densitometry of Caspase-1 (B) protein expressions normalized for 0 h light levels without BRACs (set as 1) in retinas of rats treated with (-▪-) or without (-□-) 1% BRACs for 15 days and exposed to light for 0~24 h. Values are the means for three determinations with their standard deviations shown by vertical bars for each time point. a,b,cMean values with unlike letters on the same curve or among the same diet group at different exposure times were significantly different by Ryan's multiple range test (p < 0.05). *Mean value was significantly different from that for rats at the same light exposure time but not treated with BRACs (p < 0.05, t test).
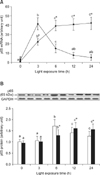
Fig. 4
Phosphorylation of IκB-α protein exposed to light for 0 ~ 24 h. (A) after exposure to light, the phosphorylation of IκB-α was analyzed at various time points with western blot and (B) semi-quantitative densitometry of phosphor-IκB-α relative to 0 h light levels (set as 1) with (-▪-) or without (-□-) 1% BRACs for 15 days. a,b,cMean values with unlike letters among the same diet group at different exposure times were significantly different (p < 0.05, Ryan's multiple range test). *Mean value was significantly different compared with rats not treated with BRACs at the same light exposure time (p < 0.05, t test).
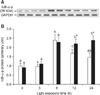
Fig. 5
The relative AP-1, c-fos, (A) mRNA expressions normalized for corresponding GAPDH levels in retinas of rats treated with (-▪-) or without (-♦-) 1% BRACs for 15 days and exposed to light for 0 ~ 24 h. The semi-quantitative densitometry of Caspase-1 (B) protein expressions normalized for 0 h light levels without BRACs (set as 1) in retinas of rats treated with (-▪-) or without (-□-) 1% BRACs for 15 days and exposed to light for 0 ~ 24 h. Values are the means for three determinations with their standard deviations shown by vertical bars for each time point. a,b,cMean values with unlike letters on the same curve or among the same diet group at different exposure times were significantly different by Ryan's multiple range test (p < 0.05). *Mean value was significantly different from that for rats at the same light exposure time but not treated with BRACs (p < 0.05, t test).
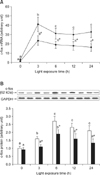
Fig. 6
The relative AP-1, c-jun, (A) mRNA expressions normalized for corresponding GAPDH levels in retinas of rats treated with (-▪-) or without (-♦-) 1% BRACs for 15 days and exposed to light for 0 ~ 24 h. The semi-quantitative densitometry of Caspase-1 (B) protein expressions normalized for 0 h light levels without BRACs (set as 1) in retinas of rats treated with (-▪-) or without (-□-) 1% BRACs for 15 days and exposed to light for 0~24 h. Values are the means for three determinations with their standard deviations shown by vertical bars for each time point. a,b,cMean values with unlike letters on the same curve or among the same diet group at different exposure times were significantly different by Ryan's multiple range test (p < 0.05).*Mean value was significantly different from that for rats at the same light exposure time treated but not treated with BRACs (p < 0.05, t test).
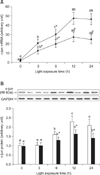
Acknowledgments
This work was supported by grants from the National Natural Science Foundation of China (81273074), the Found of Chengdu Medical College (CYZ12-015), the innovative science research team of Sichuan Province (KYTD201012), the development and regeneration key laboratory of Sichuan Province (SYS11-004) and the scientific research item of Health Department of Sichuan Province (110465). We gratefully acknowledge Wenhua Ling MD, PhD (University of Sun-Yet, China) for providing BRACs and Fred Bogott, MD, PhD (University of Minnesota, USA) for editing the manuscript.
References
1. Aonuma H, Yamazaki R, Watanabe I. Retinal cell death by light damage. Jpn J Ophthalmol. 1999; 43:171–179.


2. Barkett M, Gilmore TD. Control of apoptosis by Rel/NF-κB transcription factors. Oncogene. 1999; 18:6910–6924.


3. Büchi ER, Szczesny PJ. Necrosis and apoptosis in neuroretina and pigment epithelium after diffuse photodynamic action in rats: a light and electron microscopic study. Jpn J Ophthalmol. 1996; 40:1–11.
4. Chahory S, Padron L, Courtois Y, Torriglia A. The LEI/L-DNase II pathway is activated in light-induced retinal degeneration in rats. Neurosci Lett. 2004; 367:205–209.


5. Chandra A, Rana J, Li Y. Separation, identification, quantification, and method validation of anthocyanins in botanical supplement raw materials by HPLC and HPLC-MS. J Agric Food Chem. 2001; 49:3515–3521.


6. Cichocki M, Paluszczak J, Szaefer H, Piechowiak A, Rimando AM, Baer-Dubowska W. Pterostilbene is equally potent as resveratrol in inhibiting 12-O-tetradecanoylphorbol-13-acetate activated NFκB, AP-1, COX-2, and iNOS in mouse epidermis. Mol Nutr Food Res. 2008; 52:S62–S70.
7. Crawford MJ, Krishnamoorthy RR, Rudick VL, Collier RJ, Kapin M, Aggarwal BB, Al-Ubaidi MR, Agarwal N. Bcl-2 overexpression protects photooxidative stress-induced apoptosis of photoreceptor cells via NF-κB preservation. Biochem Biophys Res Commun. 2001; 281:1304–1312.


8. Donovan M, Carmody RJ, Cotter TG. Light-induced photoreceptor apoptosis in vivo requires neuronal nitric-oxide synthase and guanylate cyclase activity and is caspase-3-independent. J Biol Chem. 2001; 276:23000–23008.


9. Geller AM, Sutton LD, Marshall RS, Hunter DL, Madden V, Peiffer RL. Repeated spike exposure to the insecticide chlorpyrifos interferes with the recovery of visual sensitivity in rats. Doc Ophthalmol. 2005; 110:79–90.


10. Grimm C, Wenzel A, Hafezi F, Remé CE. Gene expression in the mouse retina: the effect of damaging light. Mol Vis. 2000; 6:252–260.
11. Grimm C, Wenzel A, Hafezi F, Yu S, Redmond TM, Remé CE. Protection of Rpe65-deficient mice identifies rhodopsin as a mediator of light-induced retinal degeneration. Nat Genet. 2000; 25:63–66.


12. Hafezi F, Grimm C, Wenzel A, Abegg M, Yaniv M, Remé CE. Retinal photoreceptors are apoptosis-competent in the absence of JunD/AP-1. Cell Death Differ. 1999; 6:934–936.
13. Hafezi F, Steinbach JP, Marti A, Munz K, Wang ZQ, Wagner EF, Aguzzi A, Remé CE. The absence of c-fos prevents light-induced apoptotic cell death of photoreceptors in retinal degeneration in vivo. Nat Med. 1997; 3:346–349.


14. Jang YP, Zhou J, Nakanishi K, Sparrow JR. Anthocyanins protect against A2E photooxidation and membrane permeabilization in retinal pigment epithelial cells. Photochem Photobiol. 2005; 81:529–536.


15. Kalt W, Hanneken A, Milbury P, Tremblay F. Recent research on polyphenolics in vision and eye health. J Agric Food Chem. 2010; 58:4001–4007.


16. Kaltschmidt B, Uherek M, Wellmann H, Volk B, Kaltschmidt C. Inhibition of NF-κB potentiates amyloid β-mediated neuronal apoptosis. Proc Natl Acad Sci U S A. 1999; 96:9409–9414.


17. Kowalczyk E, Krzesiński P, Kura M, Szmigiel B, Błaszczyk J. Anthocyanins in medicine. Pol J Pharmacol. 2003; 55:699–702.
18. Krishnamoorthy RR, Crawford MJ, Chaturvedi MM, Jain SK, Aggarwal BB, Al-Ubaidi MR, Agarwal N. Photo-oxidative stress down-modulates the activity of nuclear factor-κB via involvement of caspase-1, leading to apoptosis of photoreceptor cells. J Biol Chem. 1999; 274:3734–3743.


19. Li F, Cao W, Anderson RE. Alleviation of constant-light-induced photoreceptor degeneration by adaptation of adult albino rat to bright cyclic light. Invest Ophthalmol Vis Sci. 2003; 44:4968–4975.


20. Liu Y, Song X, Han Y, Zhou F, Zhang D, Ji B, Hu J, Lv Y, Cai S, Wei Y, Gao F, Jia X. Identification of anthocyanin components of wild Chinese blueberries and amelioration of light-induced retinal damage in pigmented rabbit using whole berries. J Agric Food Chem. 2011; 59:356–363.


21. Matsumoto H, Nakamura Y, Iida H, Ito K, Ohguro H. Comparative assessment of distribution of blackcurrant anthocyanins in rabbit and rat ocular tissues. Exp Eye Res. 2006; 83:348–356.


22. Matsunaga N, Imai S, Inokuchi Y, Shimazawa M, Yokota S, Hara H. Bilberry and its main constituents have neuroprotective effects against retinal neuronal damage in vitro and in vivo. Mol Nutr Food Res. 2009; 53:869–877.


23. Mattson MP, Camandola S. NF-κB in neuronal plasticity and neurodegenerative disorders. J Clin Invest. 2001; 107:247–254.


24. McGhie TK, Walton MC. The bioavailability and absorption of anthocyanins: towards a better understanding. Mol Nutr Food Res. 2007; 51:702–713.


25. Noell WK, Walker VS, Kang BS, Berman S. Retinal damage by light in rats. Invest Ophthalmol. 1966; 5:450–473.
26. Organisciak DT, Bicknell IR, Darrow RM. The effects of L-and D-ascorbic acid administration on retinal tissue levels and light damage in rats. Curr Eye Res. 1992; 11:231–241.


27. Ranchon I, Gorrand JM, Cluzel J, Droy-Lefaix MT, Doly M. Functional protection of photoreceptors from light-induced damage by dimethylthiourea and Ginkgo biloba extract. Invest Ophthalmol Vis Sci. 1999; 40:1191–1199.
28. Reeves PG, Nielsen FH, Fahey GC Jr. AIN-93 purified diets for laboratory rodents: final report of the American Institute of Nutrition ad hoc writing committee on the reformulation of the AIN-76A rodent diet. J Nutr. 1993; 123:1939–1951.


29. Stoyanovsky DA, Goldman R, Darrow RM, Organisciak DT, Kagan VE. Endogenous ascorbate regenerates vitamin E in the retina directly and in combination with exogenous dihydrolipoic acid. Curr Eye Res. 1995; 14:181–189.


31. Tanaka J, Nakanishi T, Ogawa K, Tsuruma K, Shimazawa M, Shimoda H, Hara H. Purple rice extract and anthocyanidins of the constituents protect against light-induced retinal damage in vitro and in vivo. J Agric Food Chem. 2011; 59:528–536.


32. Torres J, Enríquez-de-Salamanca A, Fernández I, Rodríguez-Ares MT, Quadrado MJ, Murta J, del Castillo JMB, Stern ME, Calonge M. Activation of MAPK signaling pathway and NF-κB activation in pterygium and ipsilateral pterygium-free conjunctival specimens. Invest Ophthalmol Vis Sci. 2011; 52:5842–5852.


33. Wenzel A, Grimm C, Marti A, Kueng-Hitz N, Hafezi F, Niemeyer G, Remé CE. c-fos Controls the "private pathway" of light-induced apoptosis of retinal photoreceptors. J Neurosci. 2000; 20:81–88.


34. Wenzel A, Grimm C, Samardzija M, Remé CE. Molecular mechanisms of light-induced photoreceptor apoptosis and neuroprotection for retinal degeneration. Prog Retin Eye Res. 2005; 24:275–306.


35. Wu J, Seregard S, Algvere PV. Photochemical damage of the retina. Surv Ophthalmol. 2006; 51:461–481.


36. Wu T, Chen Y, Chiang SKS, Tso MOM. NF-κB activation in light-induced retinal degeneration in a mouse model. Invest Ophthalmol Vis Sci. 2002; 43:2834–2840.
37. Wu T, Chiang SKS, Chau FY, Tso MOM. Light-induced photoreceptor degeneration may involve the NFκB/caspase-1 pathway in vivo. Brain Res. 2003; 967:19–26.


38. Xia M, Hou M, Zhu H, Ma J, Tang Z, Wang Q, Li Y, Chi D, Yu X, Zhao T, Han P, Xia X, Ling W. Anthocyanins induce cholesterol efflux from mouse peritoneal macrophages: the role of the peroxisome proliferator-activated receptor γ-liver X receptor α-ABCA1 pathway. J Biol Chem. 2005; 280:36792–36801.

