Abstract
The glycoprotein 3 (GP3) of type II porcine reproductive and respiratory syndrome virus has the characteristic domains of a membrane protein. However, this protein has been reported to be retained in the endoplasmic reticulum (ER) rather than transported to the plasma membrane of the cell. In this study, we performed confocal laser scanning microscopy analysis of variants of GP3 and foundthat the signal sequence of the GP3 led to confinement of GP3 in the ER, while the functional ortransmembrane domain did not affect its localization. Based on these results, we concludedthat the signal sequence of GP3 contains the ER retention signal, which might play an important role in assembly of viral proteins.
Porcine reproductive and respiratory syndrome (PRRS) has been a worldwide threat to the pig industry since it was first reported in the US [19] and Europe [36]. The etiological agent, PRRS virus (PRRSV), was isolated in Europe in 1991 and in the US in 1992 [12,36]. Weak and delayed protective immune response against PRRSV and lack of proper vaccine are the main reasons for worldwide spread of this virus [24,30,35]. PRRSV belongs to the family Arteriviridae, along with equine arteritis virus (EAV), lactate dehydrogenaseelevating virus (LDV), and simian hemorrhagic fever virus (SHFV) [6]. PRRSV can be divided into European (type 1) and American (type 2) strains based on their sequence homology [25]. PRRSV is a single positive-stranded RNA virus composed of ten different open reading frames (ORFs). ORF 1a and 1b are the largest ORFs, encompassing approximately 80% of the whole genome. Protein products translated from these sequences are cleaved into several non structural proteins (nsps), some known to be required for the replication of viruses and others with unknown functions [33]. ORF 2a, 3, 4, and 5 are glycoproteins (GPs), while ORF2 b is an envelope (E) protein and ORF 6 and 7 are matrix (M) and nucleocapsid (N) proteins, respectively [14,22,23,26,27].
GPs of PRRSVare known to be critical to infection due to their ability to interact with receptors on the cell surface, including sialoadhesin and CD 163 [6,35,37], and the formation of GP complexes [10,38]. Additionally, GPs are considered to be important antigens inimmune response against PRRSV [13,14,17,23,37].
Although minor GPs (GP2a, 3, 4) are thought to play important roles in infection and formation of the glycoprotein complex of PRRSV, their structures and functions have not been extensively investigated relative to those of GP5, which is classified as a major glycoprotein. The absence of any minor GPs or envelope protein suppresses the protein complex formation or retention of proteins in the ER, which further hinders modification at the Golgi complex for unknown reasons [38]. Furthermore, in a recent study, minor glycoproteins GP2-GP4 were shown to be retained in the ER along with E proteins [39]. GP5 moves to the Golgi for further modification and the signal sequence is cleaved [2], which differs from other GPs.
GP3 is a minor GP that consists of 254 a.a. with a molecular weight of approximately 42 kDa and contains 7 N-glycosylation sites [14,16,22,27]. In previous studies, the secretion of GP3 of type 2 PRRSV in the medium of cell culture was reported, although it had characteristics of a typical transmembrane protein [14,18,22]. In contrast, this protein has also been reported to contain the same structural component as type 1 PRRSV [12]. GP3 was reported to be retained in the ER, but the reasons for this are not yet known [39].
It is well known that arteriviridae is assembled and budded in the ER or golgi complex, as is the necessity of viral protein complex formation; however, the method by which the proteins are assembled to the complex and budded has not yet been investigated fully. The localization of GP3 in the ER may be natural considering its assembly and budding in the ER. However,we hypothesized that this protein may have an ER retention signal among its domains and play an important role in inducing retention in the ER to facilitate budding of viruses in the ER. Therefore, we conducted this study to identify the domain containing such retention signals.
We first analyzed the amino acid sequence of GP3 to investigate if it had typical characteristics. To accomplish this, the amino acid sequence of GP3 of type II PRRSV was analyzed by the SignalP 4.0 Server provided by the Center for Biological Sequence Analysis at the Technical University of Denmark [32]. In addition, the transmembrane domain was analyzed using the DAS Transmembrane Prediction Server [10].
Plasmid containing EGFP sequence (EGFP N-1 Invitrogen, USA) was kindly provided by Dr. Chi-Ho Yu from Samseong Medical Center in Seoul, Korea. BHK-21 cells for transfection were generously provided by Dr. Yeon Gyeong Shin from the Animal and Plant Quarantine Agency (National Veterinary Research Quarantine Services, Anyang, Korea). The EGFP fusion construct containing the full sequence was designated GP3-GFP and other constructs without signal sequences and transmembrane domains were designated as GP3ΔTM2-GFP, GP3ΔTM1.2-GFP, GP3ΔS.S.-GFP, GP3ΔS.S.TM2-GFP, and GP3-ΔS.S.TM1.2-GFP, respectively. Moreover, the construct that contains signal sequence of GP3 fused with GFP was designated as S.S.-GFP. The sequences of primers are listed in Table 1. Additionally, the Kozak motif sequence (GCCGCCGCC) immediately upstream of the ATG starting codon and GC was added for every reverse primer to maintain the in-frame of GFP, which was downstream of the insert. To prevent early stop of the fusion protein, the stop codon of the insert was also removed. Sequences were amplified using 0.5 µL ExTaq (Takara Bio, Japan) and the FL-12 infectious cDNA clone (AY545985), which was generously provided by Dr. Fernando A. Osorio (University of Nebraska-Lincoln, USA) as the backbone sequence. Each PCR product was subcloned into RBC TA cloning vector (Real Bio Tech, Taiwan) and transformed into the DH 5 α cell (Real Bio Tech, Taiwan) to amplify the plasmid. The sequence of each clone was analyzed at Cosmo Gene Tech (Korea). The clones containing correct sequences were amplified in LB broth containing 25 mg/mL of ampicillin and the obtained plasmids were digested with Bam H I and Kpn I restriction enzyme (New England Biolab, USA) to generate inserts. The EGFP-N1 vector was also digested with the same restriction enzymes and the product obtained from the clone containing the PCR product was ligated with T4 ligase (New England Biolab, USA) overnight. Each ligate was transformed into DH 5 α cells and the plasmid containing the product was sequenced. Consequently, we obtained seven clones harboring each insert located upstream of the EGFP in-frame under control of the CMV promoter. The clones were cultured in 300 mL of L.B. broth containing 25 mg/mL of Kanamycin overnight (O.D. = 0.6)and the plasmid for the transfection was purified using the Maxi Prep kit (Qiagen, Germany) according to the manufacturer's protocol, after which it was stored at -20℃ for later use.
BHK-21 cells were cultured on sterile coverslips autoclaved with PBS in 60 mm culture dishes in MEM (WelGene, Korea) supplemented with 10% fetal bovine serum (FBS) (Gibco, USA) at 37℃ under 5% CO2. When cell confluency reached at 80%, the cells were incubated with 1 mL of MEM without antibiotics and fetal bovine serum (FBS) containing 25 µL of Lipofectamine Reagent (Invitrogen, USA) and 5 µg of each plasmid construct. After 5 hrs of incubation, the medium was changed into medium containing 10% FBS and incubated for an additional 12 h at 37℃ under 5% CO2.
For the colocalization study of the endoplasmic reticulum (ER) and EGFP fused protein signal by confocal microscopic analysis, the ER of transfected cells were counterstained with ER-Tracker Red marker (E34250 Invitrogen, USA). Transfected cells were incubated with ER tracker diluted in Hank's Buffered Salt Solution (Gibco, USA) to a final concentration of 1 mM at 37℃ under 5% CO2 for 30 min and then washed with PBS.
Cells were immersed with VECTASHIELD Mounting Medium purchased from Vector Laboratories (USA) to prevent the loss of fluorescence and rapid drying of cells. Consequently, the cover slip was transferred to a glass slide and visualized using the inverted confocal laser scanning microscope system (FV-1000 spectral Olympus, Japan).
The GFP fusion proteins were visualized with the GFP filter (488 nm) and the ER tracker was visualized with the Ds-Red2 filter (543 nm). For each construct, at least 30 cells were analyzed at 40× and the representative images of the cells were captured at 63× magnification using the objective lens and 3.5× software magnification at 40 µs/pixel. The captured image was analyzed with Olympus FluoView FV10-ASW software. The images of GFP fusion constructs and ER Tracker were overlaid for the colocalization study.
Computational analysis of GP3 showed that this ORF contains one signal sequence at the N-terminus (1-26 a.a.) with the expected cleavage site between amino acids 26 and 27. Additionally, the GP3 was expected to have two different transmembrane domains at amino acids 106-116 and 188-197, respectively. Based on analysis of the amino acid sequence, it contains an N-terminal signal sequence, C-terminal hydrophobic region, and several N-glycosylation sites. Additional amino acid analysis revealed that this ORF does not contain any domain for myristoylation or palmitoylation.
Interestingly, we found that the signal sequence of GP3 is rich in hydrophobic sequencesthat were similar to that of cytochrome P450, which is retained in the ER owing to its high hydrophobicity [1,31]. These findings led us to examine the possibility that GP3 is also retained in the ER and find the portion of the protein that affects localization of the protein.
We designed seven different protein constructs with or without signal sequences or trans-membrane domains based on the predicted signal sequence and trans-membrane domain prediction program (Fig. 1) and analyzed them by confocal microscopic analysis. The majority of the GP3-GFP construct was expressed in the cytoplasm without a typical rim-like staining pattern (Fig. 2A). The results also showed signals distributed at the perinucleus. Additional ER Tracker counter staining showed that the GFP signal co-localized with the ER Tracker, implyingthat this fusion protein is mainly localized in the ER (Fig. 2B and C). These results agree with the assumptions suggested by previous study [22].
In previous reports, the transmembrane domain was mainly regarded as the ER retention signal in viral protein showing the ER retention property [3,7,8,20,31]. However, GP3 had a signal sequence domain with hydrophobicity, similar to that of cytochrome P450, which acts as an ER retention signal [1]. Therefore, we constructed several GP3 variants that removed signal sequences or transmembrane domains and fused to GFP (Fig. 1) to examine the possibilities of having an ER retention signal in the signal sequences or transmembrane domain.
Upon confocal microscopic examination, GP3ΔTM2-GFP showed a distribution pattern similar to that of GP3-GFP (Fig. 3A). However, GP3ΔTM1.2-GFP localized throughout the cytoplasmwith punctuate accumulation of signals, which was similar to the results of previous studies demonstrating that the bubble-like punctuate signal in the cytoplasm is the result of accumulation of protein in the ER (Fig. 3D) [1,9,30,33]. The ER counterstaining of these two constructs also showed that they colocalized well with ER, implying that the protein is retained in the ER even after deletion of whole transmembrane domains. These result strongly support the possibility that the signal sequence is the ER retention signal, while the transmembrane domains are not (Fig. 3B, C, E and F).
To assess the effects of signal sequence deletion on the localization of GP3, the constructs GP3ΔS.S-GFP, GP3ΔS.S.TM2-GFP and GP3ΔS.S.TM1.2-GFP were also constructed. In GP3ΔS.S-GFP, which lacked the signal sequence domain of the original GP3,localization of the GFP signal was detected in the cytoplasm of the cells with similar distribution as seen in the GP3-GFP construct. However, the signal was also detected in the nucleus with the form of a web, which is thought to be affected by the diffusion-driven movement of the protein from the cytoplasm (Fig. 3G). It is thought that the signal sequence may act as a leader sequence to determine the localization and is required for a protein to be retained in the ER. In the GP3ΔS.S.TM2-GFP construct, the localization of the GFP signal was also observed in both the nucleus and cytoplasm (Fig. 3E). However, we observed partial accumulation of the GFP signal at the nuclear membrane and cytoplasmic membrane, in contrast to the results of GP3ΔS.S-GFP (marked as an arrowhead). Interestingly, the cytoplasmic signal of this construct was observed to be more granular than that of GP3ΔS.S-GFP. The GFP signal of GP3ΔS.S.TM1.2-GFP was not significantly different from that of GP3ΔS.S.TM2-GFP, which was distributed in the cytoplasm and nucleus with a punctuate signal (Fig. 3F). Partial accumulation of the GFP signal at the nuclear and cytoplasmic membrane was also observed (marked as an arrow and dotted-arrow, respectively).
Finally, to establish whether the signal sequence itself can determine the ER retention of protein, we constructed the S.S.-GFP (Fig. 1). The localization of the GFP signal was also confined to the cytoplasm and the colocalization assay of GFP and dsRed signal showed that these two signals overlap (Fig. 4A, B, and C). We also could not observe the typical membrane protein signal surrounding the cell surface. These resultsstrongly support our hypothesis that the signal sequence of GP3 may have played a role as an ER retention signal in protein synthesis.
Certain proteins move to predetermined locations under control of the signal sequence, which tends to be located at the N-terminus [4,5,21]. Signal sequences are generally recognized by the signal recognition particle (SRP),which binds to the signal sequence and is immediately cleaved from the ribosome once it is synthesized. The SRP and ribosome complex is delivered to the ER to synthesize protein with modifications including glycosylation. Modified proteins are then moved to the Golgi complex for further modification and secreted following the secretory pathway or moved to the plasma membrane [17]. ER retention is generally known to be triggered by the C-terminal -KDEL or -KKXX sequence [34].
However several studies have reported that ER retention is not controlled by specific KDEL or KXXX signals. Indeed, some viral proteins have been shown to remain in the ER, even without traditional ER retention signals, and authors have assumed that this would be due to the high hydrophobicity of the transmembrane domain [3,7,8,20,31].
In these cases, it has been suggested that the ER retention is mediated by the transmembrane domain rather than the signal sequence. In contrast to these results, the ER retention of GP3 of type 2 PRRSV did not disappear after the removal of two transmembrane domains, even though aggregation of the GFP signal increased. These results support the findingthat the ER retention is mediated by the signal sequence rather than the transmembrane signal. Additionally, the GFP fused with the signal sequence also showed an ER localization signal, strongly suggesting that the signal sequence is an indispensible ER retention signal for GP3. All variants with signal sequences (GP3-GFP, GP3ΔTM2-GFP, GP3ΔTM1.2-GFP) showed ER specific localization and were absent from the nucleus and plasma membrane. The first construct, which was designated as GP3-GFP, was localized in the ER (Fig. 3A-C), while the GP3ΔTM2-GFP construct, which removed the second transmembrane domain of GP3, showed a similar localization pattern. Additionally, the GP3ΔTM1.2-GFP showed an ER localization signal with a higher aggregation pattern.
The variants with the signal sequence removed (GP3ΔS.S.-GFP, GP3ΔS.S.TM2-GFP, GP3ΔS.S.TM1.2-GFP) showed similar patterns, but the signals were also observed in the nucleus. The significant difference in localization between the groups with and without the signal sequence is believed to be caused by the retention ability of the signal sequence of GP3 in the ER. Moreover, the lack of ER retention in response to deletion of the signal sequence and transmembrane domain from the original sequence of GP3 supports the possibility that the functional domain of this protein does not have an ER retention signal.
In EAV, which is also classified asArteriviridae, GP3 has attracted interest regarding its localization and the non-cleavage of the signal sequence after translation. In this study, GP3 was mainly located in the perinuclear area, and the molecular weight of the expressed GP3 without microsomal transfection showed similar movement to that of normal GP3 treated with Endo-H, implying that the signal sequence of the GP3 of EAV is not cleaved by signal peptidase [15,37].
The first glycosylation site of GP3 in both EAV and PRRSV is located at site N-29. In previous studies, it was hypothesized that N-29 that is very close to the cleavage site (a.a. 26-27) may hinder cleavage of the signal sequence of the GP3 by signal peptidase [15]. It can be speculated that the high homology between the GP3 of EAV and PRRSV causeshindrance of cleavage of the signal sequence and subsequently may have caused similar localization of the GP3 in both viruses, facilitating ER localization of the protein.
In previous studies, sensitivity to Endo-H was observed in the single transfection product of GPs 2, 4 and 5, which supported the assumption that these proteins may reside somewhere between the ER and the Golgi complex [22,23,27,28,38]. Moreover, in an immunoprecipitation study, GPs 2, 3, 4 and 5 were shown to interact together to form a complex [11,38]. Similarly, GPs 2, 3 and 4 of EAV form a trimer via cysteine linkage [37]. It is also possible that the reason PRRSV and EAV lacking any GPs have reduced infectivity even though the viral particles were released [29,38] may be their inability to release the protein complex, which acts as a ligand to bind to the cellular receptor.
Based on the results of this and previous studies, it is feasible that the signal sequence of GP3 may be sufficient for ER retention of GP3. This retention may also be required for the assembly of PRRSV viral proteins in the ER. In future studies, we will focus on revealing the key signal releasing the GP complex.
Figures and Tables
Fig. 1
Schematic pictures of GFP fusion construct of GP3 variants. The constructs were designed based on computational analysis of the amino acid sequence of GP3. Constructs containing original GP3 and mutants with deletion of the signal sequence or transmembrane domain fused with GFP were created. Additionally, to confirm the effects of the signal sequence on localization of the GP3, constructs containing signal sequence fused with GFP were also built. Each construct was designated based on the domains that the fusion protein contained. SS and TM represent signal sequence and transmembrane domain, respectively.
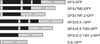
Fig. 2
Confocal microscopic examination of BHK-21 cells transfected with GP3-GFP construct. (A) The GP3-GFP construct showed an evenly distributed GFP localization signal (green) in the perinucleus area excluding the nucleus. No rim-like signals at the nuclear or plasma membrane were observed, which is a typical sign of a transmembrane protein. (B) Endoplasmic reticulum (ER) was also counter stained with ER-Tracker (red) and visualized. (C) When these two signals were overlaid, strong colocalization signal was observed. Scale bars = 10 µm.
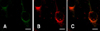
Fig. 3
Confocal microscopic examination of cells transfected with variants of GP3-GFP. (A~C) GP3ΔTM2-GFP shows distribution of the GFP signal (green) in the cytoplasm excluding the nucleus and its colocalization with ER counterstaining (red). (D~F) GP3ΔTM1,2-GFP showed similar distribution to that of GP3ΔTM2-GFP. However, the GFP signal showed granular accumulation. (G~I) GP3ΔS.S.-GFP, GP3ΔS.S.TM2-GFP, and GP3ΔS.S.TM1.2-GFP showed distribution of the GFP signal in both the nucleus and cytoplasm. Characteristically, the GFP signals of GP3ΔS.S.TM2-GFP and GP3ΔS.S.TM1,2-GFP were observed to be granular and this tendency was increased in GP3ΔS.S.TM1,2-GFP. Additionally, weak signals were observed in the nuclear membrane (arrow) in both the construct and the plasma membrane (dotted-arrow) in GP3ΔS.S.TM1.2-GFP. (J) Cells transfected with EGFP-N1 showed even distribution in the nucleus and cytoplasm. Scale bars = 10 µm.
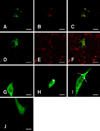
Fig. 4
Signal sequence of GP3 was fused with GFP to confirm its effect on localization. (A) GFP signal (green) was observed in the cytoplasm excluding the nucleus. No signal was observed in the plasma membrane and nuclear membrane. This distribution pattern is similar to that of GP3ΔTM1,2-GFP (Fig. 3D). (B and C) ER was counterstained with ER Tracker (red) and overlaid with GFP signal, which revealed that S.S.-GFP colocalized with ER. Scale bars = 10 µm.
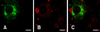
Acknowledgments
This work was supported by grant no. 610001-03-1-SU000 from Technology Development Program for Ministry of Agriculture, Food and Rural Affairs, Korea.
References
1. Ahn K, Szczesna-Skorupa E, Kemper B. The amino-terminal 29 amino acids of cytochrome P450 2C1 are sufficient for retention in the endoplasmic reticulum. J Biol Chem. 1993; 268:18726–18733.


2. Ansari IH, Kwon B, Osorio FA, Pattnaik AK. Influence of N-linked glycosylation of porcine reproductive and respiratory syndrome virus GP5 on virus infectivity, antigenicity, and ability to induce neutralizing antibodies. J Virol. 2006; 80:3994–4004.


3. Ashiru O, Bennett NJ, Boyle LH, Thomas M, Trowsdale J, Wills MR. NKG2D ligand MICA is retained in the cis-Golgi apparatus by human cytomegalovirus protein UL142. J Virol. 2009; 83:12345–12354.


4. Blobel G, Dobberstein B. Transfer of proteins across membranes. I. Presence of proteolytically processed and unprocessed nascent immunoglobulin light chains on membrane-bound ribosomes of murine myeloma. J Cell Biol. 1975; 67:835–851.


5. Blobel G, Dobberstein B. Transfer of proteins across membranes. II. Reconstitution of functional rough microsomes from heterologous components. J Cell Biol. 1975; 67:852–862.


6. Calvert JG, Slade DE, Shields SL, Jolie R, Mannan RM, Ankenbauer RG, Welch SK. CD163 expression confers susceptibility to porcine reproductive and respiratory syndrome viruses. J Virol. 2007; 81:7371–7379.


7. Cocquerel L, Duvet S, Meunier JC, Pillez A, Cacan R, Wychowski C, Dubuisson J. The transmembrane domain of hepatitis C virus glycoprotein E1 is a signal for static retention in the endoplasmic reticulum. J Virol. 1999; 73:2641–2649.


8. Cocquerel L, Meunier JC, Pillez A, Wychowski C, Dubuisson J. A retention signal necessary and sufficient for endoplasmic reticulum localization maps to the transmembrane domain of hepatitis C virus glycoprotein E2. J Virol. 1998; 72:2183–2191.


9. Collins JE, Benfield DA, Christianson WT, Harris L, Hennings JC, Shaw DP, Goyal SM, McCullough S, Morrison RB, Joo HS. Isolation of swine infertility and respiratory syndrome virus (isolate ATCC VR-2332) in North America and experimental reproduction of the disease in gnotobiotic pigs. J Vet Diagn Invest. 1992; 4:117–126.


10. Cserzö M, Wallin E, Simon I, von Heijne G, Elofsson A. Prediction of transmembrane alpha-helices in prokaryotic membrane proteins: the dense alignment surface method. Protein Eng. 1997; 10:673–676.


11. Das PB, Dinh PX, Ansari IH, de Lima M, Osorio FA, Pattnaik AK. The minor envelope glycoproteins GP2a and GP4 of porcine reproductive and respiratory syndrome virus interact with the receptor CD163. J Virol. 2010; 84:1731–1740.


12. de Lima M, Ansari IH, Das PB, Ku BJ, Martinez-Lobo FJ, Pattnaik AK, Osorio FA. GP3 is a structural component of the PRRSV type II (US) virion. Virology. 2009; 390:31–36.


13. Gagnon CA, Lachapelle G, Langelier Y, Massie B, Dea S. Adenoviral-expressed GP5 of porcine respiratory and reproductive syndrome virus differs in its cellular maturation from the authentic viral protein but maintains known biological functions. Arch Virol. 2003; 148:951–972.


14. Gonin P, Mardassi H, Gagnon CA, Massie B, Dea S. A nonstructural and antigenic glycoprotein is encoded by ORF3 of the IAF-Klop strain of porcine reproductive and respiratory syndrome virus. Arch Virol. 1998; 143:1927–1940.


15. Hedges JF, Balasuriya UB, MacLachlan NJ. The open reading frame 3 of equine arteritis virus encodes an immunogenic glycosylated, integral membrane protein. Virology. 1999; 264:92–98.


16. Jiang W, Jiang P, Li Y, Wang X, Du Y. Analysis of immunogenicity of minor envelope protein GP3 of porcine reproductive and respiratory syndrome virus in mice. Virus Genes. 2007; 35:695–704.


17. Kanner EM, Klein IK, Friedlander M, Simon SM. The amino terminus of opsin translocates "posttranslationally" as efficiently as cotranslationally. Biochemistry. 2002; 41:7707–7715.


18. Katz JB, Shafer AL, Eernisse KA, Landgraf JG, Nelson EA. Antigenic differences between European and American isolates of porcine reproductive and respiratory syndrome virus (PRRSV) are encoded by the carboxyterminal portion of viral open reading frame 3. Vet Microbiol. 1995; 44:65–76.


19. Keffaber KK. Reproductive failure of unknown etiology. Am Assoc Swine Pract Newsl. 1989; 1:1–9.
20. Krogerus C, Samuilova O, Pöyry T, Jokitalo E, Hyypiä T. Intracellular localization and effects of individually expressed human parechovirus 1 non-structural proteins. J Gen Virol. 2007; 88(Pt 3):831–841.


21. Lingappa VR, Katz FN, Lodish HF, Blobel G. A signal sequence for the insertion of a transmembrane glycoprotein. Similarities to the signals of secretory proteins in primary structure and function. J Biol Chem. 1978; 253:8667–8670.


22. Mardassi H, Gonin P, Gagnon CA, Massie B, Dea S. A subset of porcine reproductive and respiratory syndrome virus GP3 glycoprotein is released into the culture medium of cells as a non-virion-associated and membrane-free (soluble) form. J Virol. 1998; 72:6298–6306.


23. Mardassi H, Massie B, Dea S. Intracellular synthesis, processing, and transport of proteins encoded by ORFs 5 to 7 of porcine reproductive and respiratory syndrome virus. Virology. 1996; 221:98–112.


25. Meng XJ, Paul PS, Halbur PG, Morozov I. Sequence comparison of open reading frames 2 to 5 of low and high virulence United States isolates of porcine reproductive and respiratory syndrome virus. J Gen Virol. 1995; 76(Pt 12):3181–3188.


26. Meulenberg JJ, Petersen-den Besten A. Identification and characterization of a sixth structural protein of Lelystad virus: the glycoprotein GP2 encoded by ORF2 is incorporated in virus particles. Virology. 1996; 225:44–51.


27. Meulenberg JJM, Petersen-den Besten A, De Kluyver EP, Moormann RJM, Schaaper WM, Wensvoort G. Characterization of proteins encoded by ORFs 2 to 7 of Lelystad virus. Virology. 1995; 206:155–163.


28. Meulenberg JJ, van Nieuwstadt AP, van Essen-Zandbergen A, Langeveld JP. Posttranslational processing and identification of a neutralization domain of the GP4 protein encoded by ORF4 of Lelystad virus. J Virol. 1997; 71:6061–6067.


29. Molenkamp R, van Tol H, Rozier BC, van der Meer Y, Spaan WJ, Snijder EJ. The arterivirus replicase is the only viral protein required for genome replication and subgenomic mRNA transcription. J Gen Virol. 2000; 81:2491–2496.


30. Ostrowski M, Galeota JA, Jar AM, Platt KB, Osorio FA, Lopez OJ. Identification of neutralizing and nonneutralizing epitopes in the porcine reproductive and respiratory syndrome virus GP5 ectodomain. J Virol. 2002; 76:4241–4250.


31. Pedrazzini E, Villa A, Borgese N. A mutant cytochrome b5 with a lengthened membrane anchor escapes from the endoplasmic reticulum and reaches the plasma membrane. Proc Natl Acad Sci U S A. 1996; 93:4207–4212.


32. Petersen TN, Brunak S, von Heijne G, Nielsen H. SignalP 4.0: discriminating signal peptides from transmembrane regions. Nat Methods. 2011; 8:785–786.


33. Snijder EJ, van Tol H, Roos N, Pedersen KW. Non-structural proteins 2 and 3 interact to modify host cell membranes during the formation of the arterivirus replication complex. J Gen Virol. 2001; 82:985–994.


34. Stornaiuolo M, Lotti LV, Borgese N, Torrisi MR, Mottola G, Martire G, Bonatti S. KDEL and KKXX retrieval signals appended to the same reporter protein determine different trafficking between endoplasmic reticulum, intermediate compartment, and Golgi complex. Mol Biol Cell. 2003; 14:889–902.


35. Van Gorp H, Van Breedam W, Delputte PL, Nauwynck HJ. Sialoadhesin and CD163 join forces during entry of the porcine reproductive and respiratory syndrome virus. J Gen Virol. 2008; 89:2943–2953.


36. Wensvoort G, Terpstra C, Pol JMA, ter Laak EA, Bloemraad M, de Kluyver EP, Kragten C, van Buiten L, den Besten A, Wagenaar F, Broekhuijsen JM, Moonen PLJM, Zetstra T, de Boer EA, Tibben HJ, de Jong MF, van't Veld P, Groenland GJR, van Gennep JA, Voets MT, Verheijden JHM, Braamskamp J. Mystery swine disease in The Netherlands: the isolation of Lelystad virus. Vet Q. 1991; 13:121–130.


37. Wieringa R, de Vries AAF, Raamsman MJB, Rottier PJM. Characterization of two new structural glycoproteins, GP3 and GP4, of equine arteritis virus. J Virol. 2002; 76:10829–10840.

