Abstract
Chronic wasting disease (CWD) is classified as a transmissible spongiform encephalopathy or prion disease that affects cervids. CWD has been reported in 15 US states, two Canadian provinces, and in imported elk on several farms in Korea. This study was conducted to examine the molecular biological and pathogenic characteristics of a CWD-associated prion isolated in Korea. The epidemiological origin of this pathogen was also determined. Homozygous TgElk mice were infected with a CWD-affected elk brain pool prepared from the brain of an imported Canadian elk. We measured the incubation time of the pathogen, neuropathological changes by immunohistochemical staining, the pattern(s) of scrapie prion protein (PrPSc) deposition, and PrPSc protein profiles by Western blotting. We found that TgElk mice infected with brain homogenate from the elk suffering from CWD showed incubation times, vacuolar degeneration, and PrPSc accumulation similar to those previously reported in the literature. Our results suggest that homozygous TgElk mice efficiently transmit CWD with short incubation times and that this animal can serve a valuable research model and reliable in vivo diagnostic tool.
Chronic wasting disease (CWD) is a transmissible spongiform encephalopathy or prion disease that affects cervids [9]. CWD has been found in North America and Korea. In Korea, this disease was first observed in 2001 [11] and additional cases were reported from 2004 to 2006 [4]. Although the epidemiology of clinical cases with pathological diagnoses was reported, no studies have been conducted to identify and characterize the CWD prion found in Korea. To enhance public health and safety, surveillance of CWD in cervids will continue in concert with similar activities for monitoring bovine spongiform encephalopathy (BSE).
Assessment of the biological and pathological characteristics of the current CWD isolate, which was the one found in Korea, in transgenic mice will be important for determining its relationship to other isolates obtained in future studies. Biological characteristics of this pathogen depend on an interaction between strains of transmissible spongiform encephalopathy (TSE) infectious agents and host genetic factors. Additionally, the incubation period in specified mouse strains and patterns of vacuolation in the brains of affected mice will have significant effects. Recently, several research groups developed transgenic mice expressing the cervid prion protein (PrP) that were subsequently utilized for a bioassay to detect CWD prions [1,6,7,10,12]. Transmissibility of the causal agent was demonstrated by inoculation of mice expressing the cervid PrP transgene with CWD prion, thereby identifying the disease as a form of TSE often referred to as prion disease. The agents responsible for prion diseases such as CWD have also been found to vary from strain to strain [2,10]. The molecular basis for strain diversity and the mechanisms by which different strains cause different lesion patterns or have different incubation periods are not well understood. Here, we describe the primary isolation and transmission of a CWD pathogenic agent from an imported Canadian elk in Korea clinically diagnosed with CWD to susceptible TgElk mice [7].
Breeding pairs of CWD-susceptible PRNP genotype 132M/M homozygous TgElk mice [7] were kindly provided by Dr. Richard I. Carp (Institute for Basic Research in Developmental Disabilities, USA). CWD-infected brain tissues were homogenized in 0.01 M phosphate-buffered saline (PBS) under aseptic conditions using sterile disposable instruments (Precision tissue grinder system, USA). A total of six-week-old 42 female TgElk mice were anesthetized by isoflurane inhalation and inoculated with 30 µL of 10% brain homogenates from an elk suffering from CWD through an intracerebral route. Control inoculum was prepared from the brains of uninfected TgElk mice. The mice were observed every day until neurologic symptoms developed. All animal experiments were approved by the Hallym Medical Center Institutional Animal Care and Use Committee (HMC2009-1-0723), Korea. The procedures were conducted to minimize the number of animals used and animal suffering.
Frozen brains of TgElk mice were homogenized with a 10% volume of modified radioimmunoprecipitation assay buffer (20 mM Tris-HCl, pH 7.5; 150 mM NaCl, 1% Triton X-100, 0.5% sodium deoxycholate, 0.1% sodium dodecyl sulfate, 1% NP-40, 10 mM NaF, 1 mM Na3VO4, 1 mM EDTA, 1 mM EGTA, and 1 mM PMSF) containing a complete protease inhibitor cocktail (Roche, Switzerland). For Western blotting, aliquots of total tissue homogenate frozen at -70℃ were used. The protein content was determined using a bicinchoninic acid assay (Thermo Scientific, USA). Equal amounts of protein (100 µg) were heated to 95℃ for 5 min before being loaded into each lane in 15% SDS gel with a loading buffer containing 0.125 M Tris-HCl, pH 6.8; 20% glycerol, 5% SDS, 10% mercaptoethanol, and 0.002% bromophenol blue. The proteins were separated by gel electrophoresis and transferred to nitrocellulose-membranes (Amersham, UK) using an electrophoretic transfer system (BioRad, USA) at 60 V for 1 h. The membranes were washed with a Tris-buffered saline solution (pH 7.6) containing 0.05% Tween 20 (TBST) and then blocked in TBST containing 5% skim milk (Santa Cruz Biotechnology, USA) for 60 min at room temperature. The membranes were then incubated overnight at 4℃ with primary antibody against PrP (mouse monoclonal 1C5, 1:5,000 dilution) [3] diluted in TBST containing 3% bovine serum albumin (Santa Cruz Biotechnology, USA). After washing in TBST, the membranes were incubated with horseradish peroxidase-conjugated secondary antibodies against mouse IgG (1:5,000 dilution; Thermo Scientific, USA) for 30 min at room temperature, and washed in TBST again. The blots were finally developed using a chemiluminescence kit (Thermo Scientific, USA) and detected on X-ray film (Agfa, USA).
Immunohistochemical analyses were performed using an ABC kit (Vector Laboratories, USA) according to a modified avidin-biotin-peroxidase method [3]. Briefly, 6-µm sections of brain were deparaffinized with xylene, hydrated in a graded ethanol series, and then treated with 0.3% hydrogen peroxide in methyl alcohol for 30 min to block endogenous peroxidase activity at room temperature. The sections were then incubated with 5% normal donkey serum (Jackson ImmunoResearch, USA) and incubated overnight at 4℃ with rabbit polyclonal antibody specific for glial fibrillary acidic protein (anti-GFAP, 1:500 dilution; Dako, Denmark). To stain the prion protein, the sections were subjected to heat-induced antigen retrieval in a microwave oven (LG electronics, Korea) for 5 min prior to initiation of the immunohistochemistry protocol. After three washes in PBS, the sections were incubated with 10% normal donkey serum for 1 h at room temperature and then mouse monoclonal antibody specific for prion protein (mouse monoclonal 1C5, 1:1,000 dilution) overnight at 4℃ [3]. After washing in PBS, the sections were sequentially treated with biotinylated anti-mouse IgG or anti-rabbit IgG (1:1,000 dilution respectively, Jackson ImmunoResearch) and avidin-biotin peroxidase complexes (1:25; Vector Laboratories, USA), and developed with a diaminobenzidine (DAB)-hydrogen peroxide solution (0.003% DAB and 0.03% hydrogen peroxide in 50 mmol/L Tris buffer). Next, the sections were counterstained with hematoxylin solution, Gill No. 3 (Sigma, USA) and examined under a light microscope (Olympus BX51; Olympus, Japan). In addition, double-labeling was performed to examine the distribution of microglia and astrocytes. After DAB development, endogenous avidin/biotin activity in the sections was blocked by avidin-biotin blocking kit (Invitrogen, USA) for 10 min, and the sections were subsequently incubated with 5% normal donkey serum at room temperature. The sections were incubated with secondary antibody (Anti-GFAP, 1:500; Dako, Denmark or Anti-CD11b, 1:50; Serotec, USA) for 1 h at room temperature and then stained sequentially with biotinylated IgG (anti-rabbit or anti-rat 1:1,000 dilution respectively; Jackson ImmunoResearch, USA) for 30 min and avidin-biotin alkaline phosphatase complexes (Vectastain ABC-AP kit; Vector Laboratories, USA) for 30 min. Alkaline phosphatase activity was identified by staining with a freshly prepared solution of NBT/BCIP (nitro blue tetrazolium chloride/5-bromo-4-chloro-3-indolyl phosphate) substrate (Vector Laboratories, USA) with 1% levamisole (Vector Laboratories, USA) added to block endogenous alkaline phosphatase activity. The sections were mounted in permount (Thermo Fisher Scientific, USA). Control sections were stained as described above without the first or second primary antibody.
Semi-quantitative vacuolar degeneration grading was performed in nine standard sites of each mouse brain using previously established methods [5,7]. Briefly, areas of vacuolar degeneration in the mouse brains were counted in paraffin sections under a light microscope (BX51, 100× magnification; Olympus, Japan). Three tissue sections from each of the four tissue blocks for each mouse were used for lesion counting. The scoring system assessed the degree and magnitude of scrapie prion protein (PrPSc) accumulation, and the number and size of vacuolar changes were expressed with the following scale: 0, no vacuolation; 1, a few vacuoles widely and unevenly scattered; 2, a few vacuoles evenly scattered; 3, a moderate number of vacuoles evenly scattered; and 4, marked vacuolation.
To test the susceptibility of TgElk mice to brain homogenates from a diseased elk, we intracerebrally inoculated mice that overexpress the elk PrP allele. The inoculum contained 10% homogenates of the obex region as confirmed by Western blotting and immunohistochemical diagnosis of PrPSc performed at our institute. We noted when the infected mice developed clinical symptoms and assessed survival activity (Fig. 1). Average incubation times are noted in Table 1. The TgElk mice survived up to 1 to 2 weeks after the onset of clinical signs. Infected animals displayed symptoms similar to ME7 scrapie-infected mice including weight loss, lack of grooming, gait abnormalities including paresis, kyphosis, inactivity, and disorientation after an early phase of hyperactivity and aggressiveness (Fig. 2F). All 13 mice injected with the Korean CWD inoculate died with a mean incubation period of 93.5 ± 4.94 days. The mock-infected control mice survived up to 500 days without developing any symptoms (Figs. 1 and 2A). Homogenates of CWD-positive TgElk mice were then injected into naive TgElk mice (second passage), resulting in a mean incubation period of 91.5 ± 8.01 days. According to a report, TgElk mice develop symptoms with incubation periods of 94~95 days for primary passage. Upon secondary passage, the incubation time is reduced to 90 days.
Western blot analysis of disease-associated prion proteins in brain homogenate from the infected TgElk mice showed the presence of protease-resistant PrPSc as expected (Fig. 3). The strongest signal was observed for diglycosylated PrPSc whereas the unglycosylated form (lower band) showed the weakest signal. Analysis of the PrPSc profiles in brain homogenate from the infected TgElk mice showed that the molecular weight and glycosylation patterns of PrPSc were similar to those of the CWD elk brain homogenate used to infect the mice.
Histologic analysis of the TgElk mice following injection with the CWD elk brain pool revealed neuropathologic changes similar to those previously reported. Hematoxylin and eosin staining revealed widespread spongiform degeneration in all brain regions compared to the mock-infected control mice (Fig. 4) with particularly intense vacuolation in the olfactory bulb, hippocampus, anterior cortex, and periventricular zone of the medullar oblongata (Figs. 2B and G). Immunostaining with antibodies against GFAP revealed characteristic intense astrocytic gliosis typical of prion diseases (Figs. 2C and H) while antibodies against CD11b produced evidence of activating microgliosis (Figs. 2D and I). Immunohistochemical staining with monoclonal 1C5 antibody showed a neuroanatomical distribution of elk PrP in the TgElk mouse brain similar to the patters previously reported for CWD. Numerous PrP-immunoreactive plaques (Fig. 2C), which are common in the brain of elk with CWD were only seen in the brains of infected TgElk mice (Figs. 2E and J). Most plaques were periventricular but some were located away from the ventricle, particularly in the corpus callosum, and formed a rosary-like shape (Fig. 2N). Multifocal plaques were also observed in the septal area, anterior cortex, and hippocampal neuropil along with florid plaques (Fig. 2L).
In addition, double-labeling for GFAP or CD11b verified that activated and recruited glial cells were surrounded by numerous PrP-immunopositive plaques (Figs. 2N and O). CD11b immunostaining for microglia was detected primarily in the hippocampal CA3 site (Fig. 2M). TgElk injected with the Korean CWD isolate developed vacuolar profiles similar to those associated with a CWD isolate from the US (Fig. 4). However, the profile of TgElk mice infected with the Korean elk isolate was markedly different from the lesion profile of TgElk mice infected with a mule deer isolate from the US (Fig. 4).
Thus far, Korea appears to be free of both scrapie- and BSE-positive animals. In contrast, CWD is present in Korea due to the inadvertent importation of CWD-positive elk from North America. The purpose of the current study was to identify the characteristics of TgElk mice infected with Korean CWD isolates. With this information, it will be possible to identify future isolates. It will also be important to distinguish additional cases of CWD from other prion diseases. Furthermore, the animal model described in our study can be used to perform future pathogenesis studies having identified the basic characteristics of TgElk mice afflicted with CWD. It is also useful to have an easily infected, well-characterized model with a comparably short incubation period for epidemiological studies. We found it interesting that the lesion profiles of TgElk mice infected with isolates from an elk with CWD are markedly different from those inoculated with mule deer homogenates.
The findings of the current study are similar to those obtained in TgElk mice injected with CWD isolates from elk in the US [7]. In the report by LaFauci et al. [7], intracerebral injection of elk CWD isolates into TgElk mice led to disease development with incubation periods of 94~95 days. Upon secondary passage, the incubation time was reduced to 90 days. The Korean CWD pool in the present study had similar incubation periods in the TgElk mice. This result was obtained with challenge concentrations 10-fold higher than the 1% inocula used in the LaFauci et al. study [7]. However, it has been found that there is no relationship between incubation time and inoculation titer at these high ranges of infectivity [8]. The TgElk mice in our study also developed the disease more rapidly than transgenic mice used in a number of other investigations [1,6,10,12]. It is still unclear why there were differences of incubation periods among the various studies. It is most probable that this is a function of different CWD transgenes [1,6]. In the present study, the pathological appearances of TgElk mice infected with brain homogenates from a Canadian elk with CWD and those with CWD due to an endemic outbreak in Korea were identical.
In conclusion, findings from the present study indicate that intracerebral challenge of TgElk mice with brain homogenates from Korean endemic CWD cases results in spongiform encephalopathy marked by PrPSc accumulation in the central nervous system and incubation periods comparable to those previously reported [7]. Based on Western blotting, morphological and immunohistochemical characteristics, clinical findings, and the incubation period, effects of the Korean elk brain homogenates cannot be discriminated from those of CWD isolates from North American elk with the currently available diagnostic techniques. We found that our CWD elk-TgElk mouse model system is suitable for analyzing biochemical and structural properties of the infectious process as well as diagnosing mammalian prion diseases.
Figures and Tables
Fig. 1
Survival curves of cervidized Tg mice (TgElk) mice after injection of brain homogenates from chronic wasting disease (CWD)-positive elk and from CWD-positive TgElk mice (second passage).
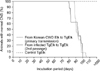
Fig. 2
Pathological findings in brains of TgElk mice infected with brain homogenate from CWD-positive elk. Sections from the brain at the level of the hippocampus of an age-matched healthy control (A~E) and a Korean CWD isolate-infected TgElk mouse (F~O). Each section was stained with hematoxylin and eosin or subjected to immunohistochemistry to monitor the presence glial fibrillary acidic protein, CD11b, and PrP. CC: corpus callosum, CA3: cornu ammonis3, arrows: vacuole, asterisks: florid plaque. Scale bars = 500 µm (B, C, D, E, G, H, I, J, M, and N), and 50 µm (K, L, and O).
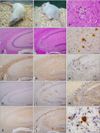
Fig. 3
Scrapie prion protein (PrPSc) Western blot patterns of brain homogenate from CWD-positive elk and infected TgElk mice. Lanes 1 and 2: homogenates from the donor elk with CWD; lanes 3~8: three control TgElk mice brains; lanes 9~16: four infected TgElk mice brains. '-': untreated brain homogenates, '+': brain homogenates treated with proteinase K (PK).
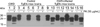
Acknowledgments
This work was supported by the National Research Foundation of Korea Grant funded by the Korean Government (No. NRF-2011-619-E0001) and Basic Science Research Program through the National Research Foundation of Korea (NRF) funded by the Ministry of Education, Science and Technology (No. 2012-0000308), Korea.
References
1. Browning SR, Mason GL, Seward T, Green M, Eliason GAJ, Mathiason C, Miller MW, Williams ES, Hoover E, Telling GC. Transmission of prions from mule deer and elk with chronic wasting disease to transgenic mice expressing cervid PrP. J Virol. 2004. 78:13345–13350.


2. Bruce ME, Fraser H, McBride PA, Scott JR, Dickinson AG. Prusiner SB, Collinge J, Powell J, Anderton B, editors. The basis of strain variation in scrapie. Prion Diseases of Humans and Animals. 1992. New York: Ellis Horwood;497–508.
3. Choi JK, Park SJ, Jun YC, Oh JM, Jeong BH, Lee HP, Park SN, Carp RI, Kim YS. Generation of monoclonal antibody recognized by the GXXXG motif (glycine zipper) of prion protein. Hybridoma (Larchmt). 2006. 25:271–277.


4. Kim TY, Shon HJ, Joo YS, Mun UK, Kang KS, Lee YS. Additional cases of chronic wasting disease in imported deer in Korea. J Vet Med Sci. 2005. 67:753–759.


5. Kim YS, Carp RI, Callahan SM, Natelli M, Wisniewski HM. Vacuolization, incubation period and survival time analyses in three mouse genotypes injected stereotactically in three brain regions with the 22L scrapie strain. J Neuropathol Exp Neurol. 1990. 49:106–113.


6. Kong Q, Huang S, Zou W, Vanegas D, Wang M, Wu D, Yuan J, Zheng M, Bai H, Deng H, Chen K, Jenny AL, O'Rourke K, Belay ED, Schonberger LB, Petersen RB, Sy MS, Chen SG, Gambetti P. Chronic wasting disease of elk: transmissibility to humans examined by transgenic mouse models. J Neurosci. 2005. 25:7944–7949.


7. LaFauci G, Carp RI, Meeker HC, Ye X, Kim JI, Natelli M, Cedeno M, Petersen RB, Kascsak R, Rubenstein R. Passage of chronic wasting disease prion into transgenic mice expressing Rocky Mountain elk (Cervus elaphus nelsoni) PrPC. J Gen Virol. 2006. 87(Pt 12):3773–3780.


8. McLean AR, Bostock CJ. Scrapie infections initiated at varying doses: an analysis of 117 titration experiments. Philos Trans R Soc Lond B Biol Sci. 2000. 355:1043–1050.


9. Miller MW, Williams ES. Chronic wasting disease of cervids. Curr Top Microbiol Immunol. 2004. 284:193–214.


10. Raymond GJ, Raymond LD, Meade-White KD, Hughson AG, Favara C, Gardner D, Williams ES, Miller MW, Race RE, Caughey B. Transmission and adaptation of chronic wasting disease to hamsters and transgenic mice: evidence for strains. J Virol. 2007. 81:4305–4314.

