Abstract
Adipose-derived stem cells (ASCs) are believed to have potential use for treating many illnesses. Most cells, including ASCs, are generally cultured in medium containing fetal bovine serum (FBS). However, FBS, which could induce an immune response or infection, is not recommended for clinical applications. In the present study, we evaluated the morphology, proliferation rate, and characterization of rabbit ASCs grown in medium containing autologous serum (AS) and compared these cells to ones cultured with FBS. Morphological changes were monitored by microscopy and flow cytometry. Proliferation rates were assessed with cell counting and ASC phenotypes were characterized by flow cytometry using representative surface markers (CD44 and CD45). Expression of epidermal growth factor, brain-derived neurotrophic factor, and vascular endothelial growth factor was measured by reverse transcription-polymerase chain reaction. Results of our study showed that ASCs had a greater expansion rate in AS without developing morphological heterogeneity than cells grown in FBS. AS-cultured ASCs expressed representative growth factors, CD44 but not CD45, similar to cells cultured in FBS. Expression levels of some growth factors were different between AS and FBS. In conclusion, our findings indicated that AS could potentially be used as a culture medium supplement for the expansion of autologous ASCs.
Stem cells have been evaluated as a therapeutic candidate and source of cells for clinical applications. Among these, adipose-derived stem cells (ASCs) isolated from adipose tissue are well known for their accessibility, pluripotency, and ability to differentiate into mesenchymal and non-mesenchymal lineages [6,7,27,28]. Recent studies have reported that stem cells secrete various beneficial growth factors and modulate hostile environments during illness. This has been called a 'bystander effect' or 'paracrine effect' in many reports [1,9,17,19]. ASCs also express and secrete multiple factors that promote a bystander effect and have a high proliferation rate with lower senescence ratio than other types of adult stem cells [14,19,21]. For these reasons, ASCs are regarded as potential sources of cells for stem cell-based therapy.
Since the number of ASCs initially recovered may not be sufficient for clinical or experimental use, ex vivo expansion of these cells is necessary. Culture media for ASC expansion require supplementation with serum containing growth factors. One of these is fetal bovine serum (FBS). However, addition of FBS is unsuitable for clinical use due to the possibility of inducing immune reactions and bovine protein contamination [16,25]. In addition, utilization of FBS is associated with possible infection with prions that can cause variant forms of Creutzfeldt-Jakob disease [13,26]. Autologous serum (AS), which also contains the growth factors and proteins necessary for the expansion of ASCs, could be a solution for overcoming these concerns. The addition of AS to primary cultures of stem cells for clinical application has been investigated, and it was shown that AS could be a safer alternative to animal-derived or allogenic serum [5,11,12,15,23].
Rabbits, a representative non-rodent laboratory animal, are physiologically more similar to humans than mice and can be easily handled in the laboratory. For these reasons, rabbits have been extensively used for many stem cell-based studies [3,8,10]. Rabbit-derived stem cells are regarded as important tools for studying human stem cells, but additional characterization and physiology studies are needed. A question of whether the expansion of rabbit-derived ASCs with AS may induce changes in morphology, proliferation rate, and the expression of surface markers and growth factors has not been investigated. Here, we explored the effect of AS on the therapeutic characteristics of rabbit-derived ASCs.
All experimental procedures were approved by the Experimental Animal Committee of the Clinical Research Institute of Seoul National University Hospital (Korea). Subcutaneous adipose samples were acquired from four 12-week-old male New Zealand rabbits (Yonam Laboratory Animals, Korea) weighing approximately 3.5 kg. Approximately 1 g of adipose tissue was chopped with fine scissors and digested in ten mL of 0.075% collagenase type 1 solution (Invitrogen, USA) with gentle agitation for 1 h at 37℃. The upper adipocyte fractions were removed from the stromal vascular fractions (SVFs) by centrifugation at 1,200 × g for 10 min at room temperature. The remaining SVFs were treated with 3 mL red blood cell lysis buffer (Sigma-Aldrich, USA) for 10 min at room temperature, filtered through a 100-µm nylon mesh (BD Biosciences, USA), and centrifuged at 1,200 × g for 10 min at room temperature. The SVFs were re-suspended and cultured in Dulbecco's modified Eagle's medium (Welgene, Korea), containing 5% FBS (Invitrogen, USA) or rabbit-derived AS. The media were changed at 48-h intervals until the cells became confluent. Cells were passaged repeatedly after achieving a density of 80%.
Thirty mL of rabbit whole blood was taken from femoral artery under anesthesia and incubated for 2 h at room temperature and centrifuged at 1,800 × g at 4℃ for 10 min. AS was collected and filtered through a 0.2-µm membrane (BD Biosciences, USA), aliquoted (2 mL volume), and stored at -20℃ before use. The AS was heated for 30 min at 56℃ prior to the experiment.
Cell counting was performed to measure cell proliferation. Following detachment of cells with 0.25% trypsin-EDTA (Invitrogen, USA), ASCs from passage 3 were seeded in a 6-well plate (SPL, Korea) at a density of 5 × 104 cells per well. Cells were detached from the plate using TrypLE Express (Invitrogen, USA) and counted every day using a hematocytometer (Fisher Scientific, USA). This experiment was performed in quintuplicate wells.
Flow cytometry was performed on rabbit ASCs grown in 5% AS or 5% FBS using mouse monoclonal anti-rabbit CD44-FITC (BD Biosciences, USA) and anti-rabbit CD45-FITC (Antigenix America, USA) antibodies. In brief, rabbit ASCs from three passages were detached using TrypLE Express (Invitrogen, USA) and washed twice with PBS. The cell pellet was resuspended at a concentration of 1 × 106 cells / 100 µL of PBS with 1% FBS per sample. 20 µL of CD44 or CD45 antibody was added to samples except the control samples and all samples were incubated for 30 min at room temperature in the dark. The cells were then washed three times with PBS with 1% FBS and maintained on ice until analysis with a flow cytometer (FACSCalibur; BD Biosciences, USA) using the FL1 channel. To monitor morphological changes of the ASCs, a scatter plot analysis was performed using the forward/side scatter channel of the flow cytometer. This experiment was performed three times.
Total RNA was extracted from rabbit ASCs grown in 5% AS or 5% FBS at passage 3 using Trizol (Invitrogen, USA) according to the manufacturer's protocol. 2 µg of RNA was reverse-transcribed at 50℃ for 60 min with SuperScript III cDNA synthesis kit (Invitrogen, USA) containing random hexamers, 10× RT buffer, 25 mM MgCl2, 0.1 M DTT, 10 mM dNTP mix and SuperScript III RT. 2 µL of cDNA was amplified using Takara Taq HotStart Version (Takara, Japan) and the following primers: epidermal growth factor (EGF), 5' TGC CAA CTG GGG GTG CAC AG 3' (forward) and 5' CTG CCC GTG GCC AGC GTG GC 3' (reverse); brain-derived neurotrophic factor (BDNF), 5' TGA GCC TGT GTG ACA GTA TT 3' (forward) and 5' CAG CCT TCT TTT GTG TAA CC 3' (reverse); vascular endothelial growth factor (VEGF), 5' GTG GAC ATC TTC CAG GAG TA 3' (forward) and 5' TCT GAC TTC ACA TTT GTT GT 3' (reverse); glyceraldehyde-3-phosphate dehydrogenase (GAPDH), 5' CCT TCA TTG ACC TCA ACT AC 3' (forward) and 5' GGA AGG CCA TGC CAG TGA GC 3' (reverse). PCR was performed using Mastercycler (Eppendorf, Germany) under the following conditions : 95℃ for 5 min, then 32 cycles of 95℃ for 30 sec, 55℃ for 30 sec and 72℃ for 1 min, followed by 72℃ for 10 min.
Bands were visualized using SafeView (NBS biologicals, UK) and the intensity of each band was quantified using ImageJ software (National Institute of Health, USA) and normalized by those of GAPDH. This experiment was performed three times.
Error bars in the figures represent the standard error of the mean. Differences of growth factor expression profiles and doubling times between groups were evaluated with Mann-Whitney U-test. p-values < 0.05 were considered statistically significant. All calculations were performed using the Statistical Package for the Social Sciences (ver. 14; IBM, USA).
The isolation and culturing of ASCs from rabbit adipose tissue were performed with media containing either AS and FBS. We observed the morphology of ASCs grown in 5% AS or 5% FBS during primary culturing. During this time, ASCs isolated and expanded in 5% AS were morphologically similar to those grown in 5% FBS. On the other hand, heterogeneous morphology was observed in FBS-treated ASCs and homogeneous morphology was observed in AS-cultured ASCs at passage 3 (Fig. 1A). Results of the flow cytometry analysis conducted at passage 3 demonstrated that AS-treated ASCs constituted a crowded cell population whereas cells grown in FBS had a dispersed population on the scatter plot (Fig. 1B). To measure the ASC growth rate, we counted the number of cells cultured with 5% AS or 5% FBS every day after seeding at passage 3. As shown in Fig. 1C, the proliferation rate was faster for cells grown in 5% AS than those cultured with 5% FBS. Doubling times during the log phase of growth for these two populations were 19 h and 26 h, respectively (Fig. 1C). These results showed that AS can induce ASC proliferation at a slightly faster rate than FBS without promoting morphological heterogeneity.
To compare surface marker expression profiles, ASCs grown in medium containing 5% AS or 5% FBS at passage 3 were detached and subjected to flow cytometry analysis. As shown in Fig. 2, ASCs grown in either 5% AS or 5% FBS were positive for CD44 expression and negative for CD45 expression. These data imply that ASCs could be maintained in AS without undergoing significant changes in surface marker profiles.
Growth factors secreted by ASCs include VEGF, EGF, and BDNF, implying that these cells exert a paracrine effect during cell therapy. We measured mRNA expression of these growth factors in cells cultured with either AS or FBS. ASCs were harvested at passage 3 and RT-PCR analysis was performed. As shown in Fig. 3A, both AS and FBS induced the expression of EGF, VEGF, and BDNF. EGF (FBS: 52.6 ± 5.8%, p = 0.05 compared with AS) and BDNF (FBS: 265.4 ± 15.8%, p = 0.05 compared with AS) expression levels were different to some degree while VEGF expression was similar when comparing the two cell populations (Fig. 3B). According to these findings, AS can help maintain the expression of growth factors by ASCs.
In the present study, we determined whether medium containing AS is appropriate for maintaining and expanding cultures of rabbit ASCs. Effects on the morphology, expansion rate, cell surface marker expression, and growth factor production were measured. Our data showed that the proliferation rate of ASCs cultured in AS was higher than that of cells maintained with FBS. ASC populations isolated based on their adherence ability are very heterogeneous [20]. Our microscopy observations and flow cytometry analysis demonstrated that AS-treated ASCs have a homogeneous morphology at passage 3. On the other hand, FBS-cultured ASCs were morphologically heterogenic. Scatter plot analysis also showed that AS-cultured cells represent a consistent cell population. Morphological alterations could be considered unwanted changes of stem cells [24]. For clinical application, phenotypic stability and homogeneity should be guaranteed. Our data suggest that AS could be more suitable for maintaining ASCs without inducing changes in characteristics compared to FBS.
Biological characteristics of ASCs are similar to those of bone marrow-derived mesenchymal stem cells (MSCs). Both ASCs and MSCs express the CD44 cell surface marker, but not the leukocyte marker CD45 [2,4,22]. ASCs grown in AS were CD44-positive and CD45-negative, similar to those grown in FBS.
Recent stem cell studies have described the therapeutic effects of cytokines and growth factors expressed by stem cells on several disorders. This ability is the so-called bystander effect and an important stem cell characteristic [1,9,14,17,19,21]. Expression of growth factors such as EGF, VEGF, and BDNF by ASCs cultured with AS was confirmed. Findings from our study suggest that homogenous populations of ASCs could be grown in AS at a high rate while maintaining a stem cell phenotype and ability to elicit a bystander effect.
Autologous ASCs are regarded as promising candidates for clinical use in cell-based therapies. One major barrier to their application is safety of the serum used to supplement all media currently used for ASC expansion. AS could be an appropriate supplement for expanding and maintaining ASC cultures since the possibility of inducing an immune response or contamination with xenoproteins is rare. There are conflicting opinions about the effects of AS on the isolation and expansion of autologous stem cells, and the efficacy of AS will need to be confirmed before using this reagent for clinical procedures [5,11,18].
Based on our data, we determined that AS appears to be a good alternative to FBS for culturing ASCs derived from rabbits, a non-rodent animal model useful for studying stem cell biology and transplantation [3,8,10]. Ours is the first study to demonstrate the suitability of AS for expanding and maintaining rabbit-derived ASC cultures. In conclusion, AS obtained from peripheral blood could be a safer medium supplement for ASCs and serve as a useful tool for human disease research.
Acknowledgments
This study was funded by Grant-in-Aid for Seoul National University Hospital (No. 04-2009-033), Korea.
References
1. Chen L, Tredget EE, Wu PYG, Wu Y. Paracrine factors of mesenchymal stem cells recruit macrophages and endothelial lineage cells and enhance wound healing. PLoS One. 2008; 3:e1886. PMID: 18382669.


2. Chung JY, Kim W, Im W, Yoo DY, Choi JH, Hwang IK, Won MH, Chang IB, Cho BM, Hwang HS, Moon SM. Neuroprotective effects of adipose-derived stem cells against ischemic neuronal damage in the rabbit spinal cord. J Neurol Sci. 2012; 317:40–46. PMID: 22475376.


3. de Girolamo L, Arrigoni E, Stanco D, Lopa S, Di Giancamillo A, Addis A, Borgonovo S, Dellavia C, Domeneghini C, Brini AT. Role of autologous rabbit adipose-derived stem cells in the early phases of the repairing process of critical bone defects. J Orthop Res. 2011; 29:100–108. PMID: 20607837.


4. De Ugarte DA, Alfonso Z, Zuk PA, Elbarbary A, Zhu M, Ashjian P, Benhaim P, Hedrick MH, Fraser JK. Differential expression of stem cell mobilization-associated molecules on multi-lineage cells from adipose tissue and bone marrow. Immunol Lett. 2003; 89:267–270. PMID: 14556988.


5. Drewa T, Biliński PJ, Gałazka P, Czaplewski A, Drewa Ł, Woźniak A, Sir J. Influence of autologous serum on rabbit chondrocytes proliferation in vitro. Comparing study to standard sera supplements. Ortop Traumatol Rehabil. 2003; 5:751–757. PMID: 18034068.
6. Ebrahimian TG, Pouzoulet F, Squiban C, Buard V, André M, Cousin B, Gourmelon P, Benderitter M, Casteilla L, Tamarat R. Cell therapy based on adipose tissue-derived stromal cells promotes physiological and pathological wound healing. Arterioscler Thromb Vasc Biol. 2009; 29:503–510. PMID: 19201690.


7. Estes BT, Diekman BO, Gimble JM, Guilak F. Isolation of adipose-derived stem cells and their induction to a chondrogenic phenotype. Nat Protoc. 2010; 5:1294–1311. PMID: 20595958.


8. Froehlich H, Gulati R, Boilson B, Witt T, Harbuzariu A, Kleppe L, Dietz AB, Lerman A, Simari RD. Carotid repair using autologous adipose-derived endothelial cells. Stroke. 2009; 40:1886–1891. PMID: 19286583.


9. Gnecchi M, Zhang Z, Ni A, Dzau VJ. Paracrine mechanisms in adult stem cell signaling and therapy. Circ Res. 2008; 103:1204–1219. PMID: 19028920.


10. Honda A, Hirose M, Hatori M, Matoba S, Miyoshi H, Inoue K, Ogura A. Generation of induced pluripotent stem cells in rabbits: potential experimental models for human regenerative medicine. J Biol Chem. 2010; 285:31362–31369. PMID: 20670936.
11. Im W, Chung JY, Kim SH, Kim M. Efficacy of autologous serum in human adipose-derived stem cells; cell markers, growth factors and differentiation. Cell Mol Biol (Noisy-le-grand). 2011; 57(Suppl):OL1470–OL1475. PMID: 21396339.
12. Johnson LF, deSerres S, Herzog SR, Peterson HD, Meyer AA. Antigenic cross-reactivity between media supplements for cultured keratinocyte grafts. J Burn Care Rehabil. 1991; 12:306–312. PMID: 1939301.


13. Klein R, Dumble LJ. Transmission of Creutzfeldt-Jakob disease by blood transfusion. Lancet. 1993; 341:768. PMID: 8095678.


14. Lee ST, Chu K, Jung KH, Im WS, Park JE, Lim HC, Won CH, Shin SH, Lee SK, Kim M, Roh JK. Slowed progression in models of Huntington disease by adipose stem cell transplantation. Ann Neurol. 2009; 66:671–681. PMID: 19938161.


15. Lindroos B, Boucher S, Chase L, Kuokkanen H, Huhtala H, Haataja R, Vemuri M, Suuronen R, Miettinen S. Serum-free, xeno-free culture media maintain the proliferation rate and multipotentiality of adipose stem cells in vitro. Cytotherapy. 2009; 11:958–972. PMID: 19903107.


16. Mackensen A, Dräger R, Schlesier M, Mertelsmann R, Lindemann A. Presence of IgE antibodies to bovine serum albumin in a patient developing anaphylaxis after vaccination with human peptide-pulsed dendritic cells. Cancer Immunol Immunother. 2000; 49:152–156. PMID: 10881694.


17. Martino G, Pluchino S. The therapeutic potential of neural stem cells. Nat Rev Neurosci. 2006; 7:395–406. PMID: 16760919.


18. Mazlyzam AL, Aminuddin BS, Saim L, Ruszymah BHI. Human serum is an advantageous supplement for human dermal fibroblast expansion: clinical implications for tissue engineering of skin. Arch Med Res. 2008; 39:743–752. PMID: 18996287.


19. Nakagami H, Maeda K, Morishita R, Iguchi S, Nishikawa T, Takami Y, Kikuchi Y, Saito Y, Tamai K, Ogihara T, Kaneda Y. Novel autologous cell therapy in ischemic limb disease through growth factor secretion by cultured adipose tissue-derived stromal cells. Arterioscler Thromb Vasc Biol. 2005; 25:2542–2547. PMID: 16224047.


20. Rada T, Reis RL, Gomes ME. Distinct stem cells subpopulations isolated from human adipose tissue exhibit different chondrogenic and osteogenic differentiation potential. Stem Cell Rev. 2011; 7:64–76. PMID: 20396979.


21. Rehman J, Traktuev D, Li J, Merfeld-Clauss S, Temm-Grove CJ, Bovenkerk JE, Pell CL, Johnstone BH, Considine RV, March KL. Secretion of angiogenic and antiapoptotic factors by human adipose stromal cells. Circulation. 2004; 109:1292–1298. PMID: 14993122.


22. Rodriguez AM, Pisani D, Dechesne CA, Turc-Carel C, Kurzenne JY, Wdziekonski B, Villageois A, Bagnis C, Breittmayer JP, Groux H, Ailhaud G, Dani C. Transplantation of a multipotent cell population from human adipose tissue induces dystrophin expression in the immunocompetent mdx mouse. J Exp Med. 2005; 201:1397–1405. PMID: 15867092.


23. Schallmoser K, Bartmann C, Rohde E, Reinisch A, Kashofer K, Stadelmeyer E, Drexler C, Lanzer G, Linkesch W, Strunk D. Human platelet lysate can replace fetal bovine serum for clinical-scale expansion of functional mesenchymal stromal cells. Transfusion. 2007; 47:1436–1446. PMID: 17655588.


24. Shafaei H, Esmaeili A, Mardani M, Razavi S, Hashemibeni B, Nasr-Esfahani MH, Shiran MB, Esfandiari E. Effects of human placental serum on proliferation and morphology of human adipose tissue-derived stem cells. Bone Marrow Transplant. 2011; 46:1464–1471. PMID: 21217787.


25. Tuschong L, Soenen SL, Blaese RM, Candotti F, Muul LM. Immune response to fetal calf serum by two adenosine deaminase-deficient patients after T cell gene therapy. Hum Gene Ther. 2002; 13:1605–1610. PMID: 12228015.


26. Will RG, Ironside JW, Zeidler M, Cousens SN, Estibeiro K, Alperovitch A, Poser S, Pocchiari M, Hofman A, Smith PG. A new variant of Creutzfeldt-Jakob disease in the UK. Lancet. 1996; 347:921–925. PMID: 8598754.


27. Zuk PA, Zhu M, Ashjian P, De Ugarte DA, Huang JI, Mizuno H, Alfonso ZC, Fraser JK, Benhaim P, Hedrick MH. Human adipose tissue is a source of multipotent stem cells. Mol Biol Cell. 2002; 13:4279–4295. PMID: 12475952.


28. Zuk PA, Zhu M, Mizuno H, Huang J, Futrell JW, Katz AJ, Benhaim P, Lorenz HP, Hedrick MH. Multilineage cells from human adipose tissue: implications for cell-based therapies. Tissue Eng. 2001; 7:211–228. PMID: 11304456.


Fig. 1
Morphology and proliferation rate of adipose-derived stem cells (ASCs). (A) Rabbit ASCs were grown in medium containing 5% autologous serum (AS) (left panels) or 5% fetal bovine serum (FBS) (right panels) until passage 3, ×100. Scale bar = 50 µm. (B) Forward scatter vs. side scatter dot plot of AS- (green dot) or FBS-treated (black dot) ASCs. (C) ASCs grown in AS or FBS at passage 3 were counted at 48, 72, and 96 h (n = 4) after seeding at the same density. PC: primary culture, P3: passage 3. *p < 0.05 according to Mann-Whitney U-test.
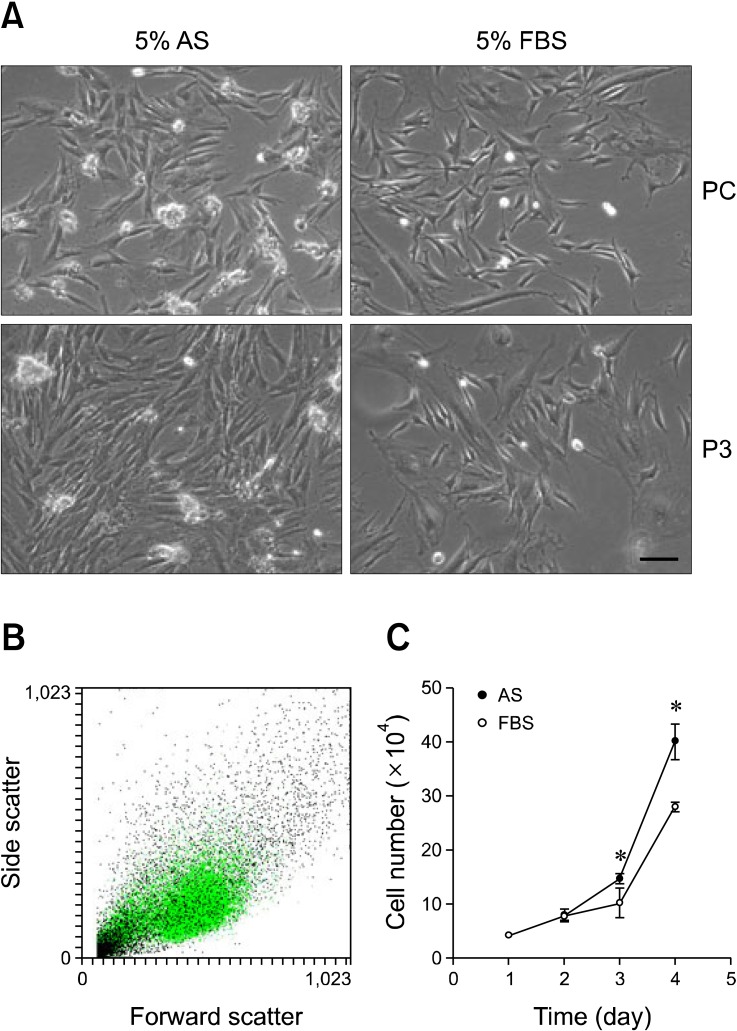
Fig. 2
Surface marker expression of the ASCs. Expression of two surface markers on ASCs cultured in AS or FBS was analyzed by flow cytometry. Detached ASCs were treated with anti-CD44 and anti-CD45 antibodies. The positive population was evaluated relative to a baseline determined with a negative control.
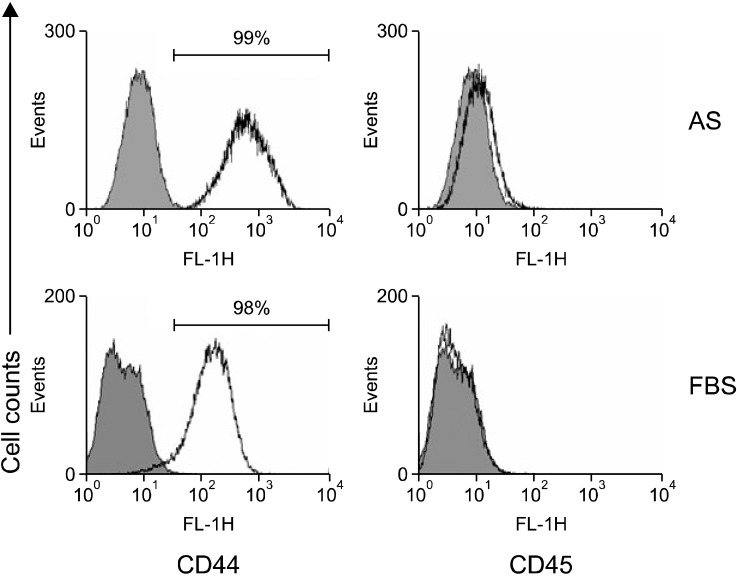
Fig. 3
Expression of growth factors by the ASCs. Rabbit ASCs were grown for three passages in 5% AS or 5% FBS, and an RT-PCR analysis was performed. (A) mRNA expression of growth factors, including epidermal growth factor (EGF), vascular endothelial growth factor (VEGF), and brain-derived neurotrophic factor (BDNF), was measured in cells cultured under both conditions. Glyceraldehyde-3-phosphate dehydrogenase (GAPDH) was used as a loading control. (B) The graph shows relative mRNA expression levels. Relative values were defined as each expression level normalized to that of GAPDH. *p < 0.05 according to Mann-Whitney U-test.
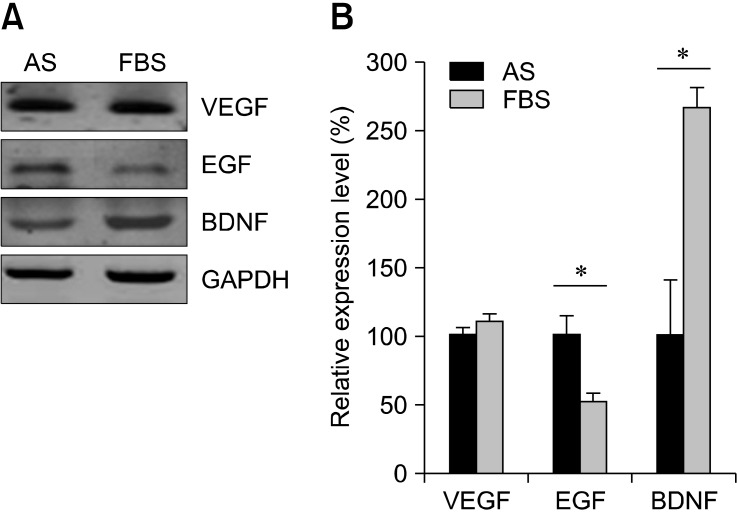