Abstract
Components of silk including silk fibroin have long been used as anti-diabetic remedies in oriental medicine. However, detailed mechanisms underlying these anti-diabetic effects remain unclear. In this study, we examined the anti-diabetic activity of silk fibroin hydrolysate (SFH) in C57BL/KsJ-db/db (db/db) mice, a well-known animal model of non-insulin dependent diabetes mellitus. When the db/db mice were administered SFH in drinking water for 6 weeks, hyperglycemia in the animals gradually disappeared and the level of glycosylated hemoglobin decreased, indicating that SFH plays important role in reducing the symptoms of diabetes. In addition, SFH-treated db/db mice exhibited improved glucose tolerance with increased plasma insulin levels. Immunohistochemical and morphological analyses showed that SFH up-regulated insulin production by increasing pancreatic β cell mass in the mice. In summary, our results suggest that SFH exerts anti-diabetic effects by increasing pancreatic β cell mass in a non-insulin dependent diabetes mellitus mouse model.
Type 2 diabetes mellitus is characterized by a deficiency in insulin secretion as well as insulin resistance in peripheral tissues [15]. Oral anti-hyperglycemic drugs play an important role in the treatment of type 2 diabetes mellitus. Anti-diabetic medications have been developed for targeting the destructive pathways that eliminate insulin-producing pancreatic β cells or in vivo formation, thereby improving insulin resistance in the peripheral tissues [3]. Oral hypoglycemic agents currently used in clinical practice have some side effects [5,14]. Side effect of metformin include diarrhea, nausea, and gas [8]. Recently, it has been hypothesized that compounds used in oriental medicine may offer alternative methods for treating diabetes mellitus due to their effectiveness, minimal side effects, and relatively low cost [18].
Root bark, fruit, and leaves of the mulberry tree have long been used as anti-diabetic remedies in oriental medicine. Some hypoglycemic substances were obtained from these materials. For example, Moran A, Moran 20K, and moranoline (1-deoxynojirimycin) were isolated from mulberry root bark [7,10,12]. N-containing sugars have also been found in mulberry leaves [22].
Silk is composed of two major polypeptides: sericin and fibroin. Fibroin is a core protein of silk that is composed of 18 different natural amino acids and has a molecular weight of 3.5~3.6 × 105 Da [17]. The major amino acids of silk fibroin are glycine, alanine, serine, and tyrosine [1]. Fibroin was found to enhance insulin sensitivity and glucose metabolism in 3T3-L1 adipocytes [9], and fibroin-derived peptides are able to increase glucose transport in normal and insulin-resistant 3T3-L1 cells [11]. In addition, fibroin lowers blood pressure in spontaneously hypertensive rats [24]. However, the detailed mechanism underlying these anti-diabetic effects remains unclear. In the present study, we examined the anti-diabetic effects of silk fibroin hydrolysate (SFH) and the associated mechanisms in C57BL/KsJ-db/db (db/db) mice, a well-known animal model of non-insulin-dependent diabetes mellitus.
C57BL/KsJ-db/db and normal non-diabetic mice (C57BL/KsJ-db/+ or +/+) 7 weeks of age were supplied by Japan SLC (Japan). The mice were housed one animal per cage during the experiment in a room with controlled temperature (23 ± 2℃), humidity (55 ± 10%), and light (08:00 a.m.~20:00 p.m.). The animals were fed a commercial laboratory chow for mice (CJ Cheiljedang, Korea) and water ad libitum. The mice were divided into four groups according to the treatment received: the DB group (C57BL/KsJ-db/db mice, n = 12), ND group (C57BL/KsJ-db/+ or +/+ mice, n = 12), DB-SF group (C57BL/KsJ-db/db mice, n = 12), and ND-SF group (C57BL/KsJ-db/+ or +/+ mice, n = 12). The DB-SF and ND-SF groups were given a 20% SFH solution prepared in water for 6 weeks. The animals were sacrificed at 14 weeks of age. The mice were allowed to acclimate to SFH treatment for 1 week before the experiments by receiving increasing concentrations in drinking water. This study was conducted in accordance with the Institutional Animal Care and Use Committees of Hallym University (Korea).
The raw cocoons were purchased from Sindobiosilk (Korea). SFH was prepared from the cocoons of Bombyx mori (Chinese silkworm). Briefly, serine and other impurities were removed from raw cocoons (1 kg) with 5% Na2CO3. Silk fibroin was treated with 2 N HCl at 100℃ for 48 h and neutralized using a 2N NaOH solution. The silk fibroin solution was mixed with chymotrypsin (Sigma, USA) and allowed to undergo proteolysis [16]. Low-molecular weight silk fibroin was acquired through a desalting process using ion exchange chromatography as previously described [16]. Amino acid composition of the SFH made by mentioned above (Table 1) was determined with an amino acid analyzer (Pico-Tag System and Waters 486/600/626; Waters, USA).
Body weights of all mice were measured with an animal balance (Mettler Toledo, USA) before and every week during the experiments. Blood samples (9:00 a.m.) were collected from the infraorbital plexus of the mice while under ether anesthesia. The plasma was obtained by centrifugation and frozen until assayed for insulin contents. Thirty micro liter of whole blood was added to the test strip and blood glucose was measured with an enzymatic dry-chemistry method (Reflotron dry-chemistry analyzer; F. Hoffmann-La Roche, Switzerland). A radioimmunoassay (RIA) was used to measure plasma insulin concentrations using a commercial RIA kit (Linco Research, USA). Absorbance at 450 nm and 590 nm was measured in a microtiter plate reader (Spectramax L; Molecular Devices, USA) within 5 min.
After 6 weeks of SFH treatment, an intraperitoneal glucose tolerance test (IPGTT) was performed 3 days before the mice were sacrificed. Mice were fasted for 12 h and then 2 g/kg of a D-glucose (Sigma, USA) solution was administered intraperitoneally. Blood samples were obtained prior to, immediately after, and 30, 60, and 120 min after glucose injection. Plasma glucose and insulin levels were measured as described above. Total area under the curve (AUC) during the course of the IPGTT was calculated according to the trapezoid rule [13].
After 6 weeks of SFH treatment, whole pancreatic tissue was obtained from each mouse. Pancreatic tissues were fixed in 10% neutral buffered formalin, embedded in paraffin, and cut into 4-µm sections with an automatic rotary microtome (2050 Supercut microtome; Reichert-Jung, Germany). The sections were incubated overnight with rabbit antibodies against pancreatic insulin (1 : 300) and glucagon (1 : 300) (Dako, Denmark) at 4℃. The sections were then incubated with peroxidase-conjugated secondary antibodies (Vectastain ABC; Vector Laboratories, USA) and developed with 3, 3'-diaminobenzidine.
Islet size and β cell mass were determined as previously described [2,6,20]. To measure islet size, a 40× magnification field was chosen at random. The islets in this field and those in the immediately adjacent fields were examined until 20 islets had been evaluated. Each of these 20 islets was evaluated to determine the total islet cross-sectional area. The percentage of the insulin-positive area (insulin immunoreactive area per µm2 of pancreatic epithelium in the sections) was measured by computer-assisted stereological morphometry using an Olympus BH-2 microscope (Olympus, Japan) connected to a Hewlett-Packard (USA) computer with Image-Pro Plus software (Media Cybernetics, USA). Fibrotic tissue outside the pancreatic lobules was excluded from the measurements. Pancreatic β cell mass was calculated by multiplying the percentage of insulin-positive areas by the weight of the corresponding pancreatic tissues.
Results are presented as the mean ± SE. To make comparisons between two groups, an unpaired Student's t-test was used. For multiple comparisons, a one-way ANOVA was performed by GraphPad Prism 4 (GraphPad Software, USA). p-values less than 0.05 were considered statistically significant.
In order to determine whether SFH can decrease blood glucose levels in db/db mice, SFH was administered to the mice for 6 weeks via drinking water. High blood glucose concentrations (18.05 ± 3.59 mmol/L) of the db/db mice gradually decreased throughout the treatment period. After 6 weeks of SFH treatment, blood glucose levels (8.83 ± 3.35 mmol/L) of the db/db animals decreased to that of the normal mice (Fig. 1A). In contrast, blood glucose levels of untreated db/db mice gradually increased from 22.94 ± 2.92 mmol/L to 32.95 ± 6.59 mmol/L within 6 weeks. SFH did not have any hypoglycemic effect on the normal mice as expected.
Throughout the experimental period, SFH treatment did not induce changes of body weight in either normal or db/db mice (Fig. 1B), indicating that SFH treatment did not have a marked nutritional effect. Glycosylated hemoglobin (HbA1c) levels of the SFH-treated db/db mice were significantly lower compared to those of the untreated db/db mice (6.55 ± 0.76 vs. 10.11 ± 1.51%, p < 0.01), indicating that glucose levels in SFH-treated db/db mice were maintained at near normal levels throughout the treatment period (Fig. 1C). Taken together, these results suggest that SFH had an anti-diabetic effect on the db/db mice.
To verify whether SFH improves glucose tolerance, we performed an IPGTT on db/db mice that had been administered SFH for 6 weeks. Blood glucose levels of the SFH-treated db/db mice were significantly lower at 30, 60, and 120 min after glucose injection compared to the untreated db/db mice (Fig. 2A), suggesting that SFH improves glucose tolerance. Plasma insulin levels of SFH-treated db/db mice were higher at 30, 60, and 120 min after glucose injection compared to the untreated db/db mice (Fig. 2B). Results from the IPGTT (Fig. 2C) showed that the AUC for glucose levels in SFH-treated db/db mice was significantly less than that of untreated db/db mice (2,751.2 ± 756.7 vs. 4,538.2 ± 425.9 mmol/L × min, respectively; p < 0.01). In addition, the AUC for insulin levels of the SFH-treated db/db mice (Fig. 2C) was significantly larger than that of untreated db/db mice (609.2 ± 197.6 vs. 292.7 ± 2.65 pmol/L × min, respectively; p < 0.01), indicating that SFH improved pancreatic β cell function. Taken together, these results suggest that SFH improved pancreatic β cell function and glucose tolerance of the db/db mice.
Since SFH treatment had a dramatic anti-diabetic effect on db/db mice, we examined histological changes in the pancreas. Pancreatic islets of the control mice (ND) contained well-granulated β cells with normal insulin immunoreactivity and peripherally located α cells (Fig. 3A and D). In comparison, insulin immunoreactivity was weak and glucagon-immunoreactive cells were observed in the center of the islets from the pancreas of control db/db mice (Fig. 3B and E). Islets from SFH-treated db/db mice had intense insulin immunoreactivity and glucagon-immunoreactive cells were redistributed at the islet periphery (Fig. 3C and F).
In order to determine how SFH increased insulin production in the db/db mice, pancreatic β cell volume was measured by morphometric analysis. Results of the analyses showed that islet density and β cell mass in the SFH-treated db/db mice were significantly increased compared to untreated db/db mice (Table 2). In addition, α cell volume was significantly decreased in the SFH-treated db/db mice relative to the untreated db/db mice (Table 2). These immunohistochemical observations were closely correlated to our biochemical measurements demonstrating the anti-diabetic effects of SFH, suggesting that this compound helped maintain pancreatic β cell integrity.
The present study was conducted to determine whether SFH has an anti-diabetic effect on a murine model of non-insulin dependent diabetic mellitus (db/db mice). The main findings of our investigation were that SFH decreased blood glucose levels and ameliorated impaired glucose tolerance in the db/db mice. SFH also improved insulin secretion by preventing the loss of β cell mass in the diabetic mice.
Our results were in accordance with those of previous studies [9,17] and add new information about how SFH works in a non-insulin dependent diabetic mellitus animal model. Our findings clearly showed that SFH helps maintain integrity of pancreatic β cells and up-regulates the production of insulin in the hyperglycemic db/db mouse. Reactive oxygen species (ROS) formation is increased to response to high glucose levels in db/db mice [4]. It is well known that pancreatic β cells are extremely sensitive to ROS, and ROS are involved in the progression of pancreatic β cell dysfunction [4]. Since SFH was found to decrease ROS formation in a pancreatic β cell line [19], the reduction of ROS by SFH treatment is likely due to elimination of glucose toxicity resulting in protection from the toxic effects of ROS. In the present study, SFH treatment decreased glucose levels and maintained β cell mass.
It has been reported that amino acids such as glycine, alanine, and serine have stimulatory effects on insulin and/or glucagon secretion in humans [21] and animals [22,23]. The SFH used in our study contained a mixture of various-sized peptides and individual amino acids. Therefore, it is possible that the single amino acids may have promoted insulin secretion in the diabetic animals. To exclude this possibility, db/db mice were treated with an amino acid mixture of the same composition. These amino acids did not decrease blood glucose levels in the db/db mice (data not shown). Recently, Kim et al. [11] reported that a fibroin-derived peptide (GAGAGY) enhances glucose transport in normal and insulin-resistant 3T3-L1 cells. It would be interesting to determine whether the SFH used in our study contains the same peptide.
In conclusion, we examined the anti-diabetic activity of SFH in C57BL/KsJ-db/db (db/db) mice. SFH improved hyperglycemia, glucose tolerance and the level of glycosylated hemoglobin (HbA1c). Immunohistochemical analyses showed that SFH up-regulated insulin production by increasing pancreatic β cell mass in the C57BL/KsJ-db/db (db/db) mice. Taken together, our studies demonstrated that SFH exerted an anti-diabetic effect in a non-insulin dependent diabetic mellitus animal model (C57BL/KsJ-db/db mice) by preventing the loss of β cells. These results suggest that dietary supplementation with SFH can be potentially useful for treating patients with non-insulin dependent diabetes mellitus.
Figures and Tables
Fig. 1
Silk fibroin hydrolysate (SFH) decreases (A) blood glucose and (C) glycosylated hemoglobin (HbA1c) levels in C57BL/KsJ-db/db mice. SFH treatment did not induce changes in body weight of either normal or db/db mice (B). Data are presented as the mean ± SE. *p < 0.05 and **p < 0.01 compared to the DB group. DB: C57BL/KsJ-db/db mice (n = 12), ND: C57BL/KsJ-db/+ or +/+ mice (n = 12), DB-SF: SFH-treated C57BL/KsJ-db/db mice (n = 12), ND-SF: SFH-treated C57BL/KsJ-db/+ or +/+ mice (n = 12).
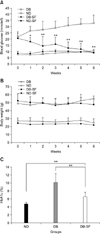
Fig. 2
SFH improves glucose tolerance in C57BL/KsJ-db/db mice. (A) Blood glucose, (B) plasma insulin, and (C) area under the curve (AUC) after glucose tolerance test in 12 week-old C57BL/sJ-db/db mice. AUC was calculated by trapezoid rule. Data are presented as the mean ± SE. **p < 0.01 compared to the DB group.
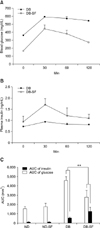
Fig. 3
SFH helps maintain pancreatic β cell integrity in the C57BL/KsJ-db/db mice. β cell morphology and distribution in the SFH-treated db/db mice were well organized. Insulin-specific (INS) (A~C) and glucagon-specific (GLU) immunohistochemical (D~F) staining, ×200.
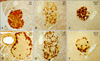
Acknowledgments
This work was supported by the Priority Research Centers Program (2012R1A6A1048184) and Basic Research Program (2011-0024062) through the National Research Foundation of Korea (NRF) funded by the Ministry of Education, Science and Technology, and by the Hallym University Research Fund, 2012 (HRF-201207-005), Korea.
References
1. Asano N, Oseki K, Tomioka E, Kizu H, Matsui K. N-containing sugars from Morus alba and their glycosidase inhibitory activities. Carbohydr Res. 1994. 259:243–255.


2. Butler AE, Janson J, Bonner-Weir S, Ritzel R, Rizza RA, Butler PC. β-cell deficit and increased β-cell apoptosis in humans with type 2 diabetes. Diabetes. 2003. 52:102–110.


3. Cheng AYY, Fantus IG. Oral antihyperglycemic therapy for type 2 diabetes mellitus. CMAJ. 2005. 172:213–226.


4. Drews G, Krippeit-Drews P, Düfer M. Oxidative stress and beta-cell dysfunction. Pflugers Arch. 2010. 460:703–718.


5. Fujimoto S. Therapeutic utility of biguanides in the treatment of NIDDM. Nihon Rinsho. 1999. 57:657–662.
6. Gedulin BR, Nikoulina SE, Smith PA, Gedulin G, Nielsen LL, Baron AD, Parkes DG, Young AA. Exenatide (exendin-4) improves insulin sensitivity and β-cell mass in insulin-resistant obese fa/fa Zucker rats independent of glycemia and body weight. Endocrinology. 2005. 146:2069–2076.


7. Hikino H, Mizuno T, Oshima Y, Konno C. Isolation and hypoglycemic activity of moran A, a glycoprotein of Morus alba root barks. Planta Med. 1985. (2):159–160.
8. Hoffmann IS, Roa M, Torrico F, Cubeddu LX. Ondansetron and metformin-induced gastrointestinal side effects. Am J Ther. 2003. 10:447–451.


9. Hyun CK, Kim IY, Frost SC. Soluble fibroin enhances insulin sensitivity and glucose metabolism in 3T3-L1 adipocytes. J Nutr. 2004. 134:3257–3263.


10. Joubert PH, Venter CP, Joubert HF, Hillebrand I. The effect of a 1-deoxynojirimycin derivative on post-prandial blood glucose and insulin levels in healthy black and white volunteers. Eur J Clin Pharmacol. 1985. 28:705–708.


11. Kim ED, Bayaraa T, Shin EJ, Hyun CK. Fibroin-derived peptides stimulate glucose transport in normal and insulin-resistant 3T3-L1 adipocytes. Biol Pharm Bull. 2009. 32:427–433.


12. Kim ES, Park SJ, Lee EJ, Kim BK, Huh H, Lee BJ. Purification and characterization of Moran 20K from Morus alba. Arch Pharm Res. 1999. 22:9–12.


13. Le Floch JP, Escuyer P, Baudin E, Baudon D, Perlemuter L. Blood glucose area under the curve. Methodological aspects. Diabetes Care. 1990. 13:172–175.


14. Lebovitz HE. Glipizide: a second-generation sulfonylurea hypoglycemic agent. Pharmacology, pharmacokinetics and clinical use. Pharmacotherapy. 1985. 5:63–77.


16. Nahm JH, Oh YS. A study of pharmacological effect of silk fibroin. RDA J Agric Sci. 1995. 37:145–157.
17. Nojima H, Kimura I, Chen FJ, Sugihara Y, Haruno M, Kato A, Asano N. Antihyperglycemic effects of N-containing sugars from Xanthocercis zambesiaca, Morus bombycis, Aglaonema treubii, and Castanospermum australe in streptozotocin-diabetic mice. J Nat Prod. 1998. 61:397–400.


18. Obatomi DK, Bikomo EO, Temple VJ. Anti-diabetic properties of the African mistletoe in streptozotocin-induced diabetic rats. J Ethnopharmacol. 1994. 43:13–17.


19. Park JH, Nam Y, Park SY, Kim JK, Choe NH, Lee JY, Oh YS, Suh JG. Silk fibroin has a protective effect against high glucose induced apoptosis in HIT-T15 cells. J Biochem Mol Toxicol. 2011. 25:238–243.


20. Rooman I, Lardon J, Bouwens L. Gastrin stimulates β-cell neogenesis and increases islet mass from transdifferentiated but not from normal exocrine pancreas tissue. Diabetes. 2002. 51:686–690.


21. Scheen AJ. Benefits and limitations of protein diets in obese patients with type 2 diabetes. Ann Endocrinol (Paris). 1999. 60:443–450.
22. Taniguchi S, Asano N, Tomino F, Miwa I. Potentiation of glucose-induced insulin secretion by fagomine, a pseudo-sugar isolated from mulberry leaves. Horm Metab Res. 1998. 30:679–683.

