Abstract
The temporal expression of estrogen receptor (ER)-α and ER-β mRNA was examined in male Japanese quails. Femurs of quails receiving 17β-estradiol underwent RTPCR and histochemical analysis 1 to 15 days after treatment. Untreated quails were used as controls (day 0). Between days 0 and 5, cells lining the bone endosteal surface differentiated into osteoblasts, which in turn formed medullary bone. Expression of ER-α was already observed on day 0 and increased slightly during bone formation whereas ER-β was hardly detected throughout this process. After osteoclasts appeared on the medullary bone surface, this type of bone disappeared from the bone marrow cavity (days 7~15). ER-α expression simultaneously decreased slightly and ER-β levels remained very low. These results suggest that estrogen activity mediated by ER-α not only affects medullary bone formation but also bone resorption.
The lack of estrogen is known to be a causative factor of osteoporosis [14]. It has therefore been suggested that estrogen is related to the differentiation and/or activities of bone cells, and many studies over the last few decades have investigated the relationships between bone metabolism and estrogen. Investigations have been performed in vivo using estrogen-injected [5,25] and ovariectomized animals [1,29], and the effects of estrogen on the differentiation and/or proliferation of osteoblasts and/or osteoclasts have been examined in vitro [11,22]. In addition, the expression of estrogen receptors (ERs) in bone cells has been evaluated both in vivo [7,30] and in vitro [17,26]. In the 1980s, a novel ER subtype, ER-β, was cloned in addition to the previously identified ER-α [9,31]. This discovery further complicated the relationship between bone metabolism and estrogen.
Formation and resorption of medullary bone in female birds is under the control of circulating estrogen [3,21]. Medullary bone can also be formed in the bone marrow cavity of male birds by estrogen administration [10,12]. ERs have been found in osteoblasts, bone-lining cells, and bone marrow cells in these experimental animal models [15,16]. As found in a previous study, ER-α mRNA, but not ER-β mRNA, is expressed at constant levels throughout the differentiation of osteogenic cells isolated from medullary bone [6]. Although these experiments were performed during the bone formation period, expression of ER-α and/or ER-β during bone resorption has not been assessed. In the present study, we analyzed the temporal expression of ER-α and ER-β mRNA during medullary bone formation and resorption in estrogen-treated male Japanese quails using reverse transcription-polymerase chain reaction (RT-PCR).
Male Japanese quails (Coturnix coturnix japonica; Quail Cosmos, Japan) 8~9 weeks old were used in our study. 17β-estradiol (E2) (Progynon-Depot, 20 mg/kg; Fuji Pharma, Japan) was injected into the breast muscle at 1, 2, 3, 5, 7, 10, and 15 days before the femurs were removed (n = 3~4). Control birds (day 0) were not treated with E2. All animal experiments were carried out in strict accordance with the Institutional Guidelines of the Committee of Research Facilities for Laboratory Animal Science, Hiroshima University, Japan.
E2-treated and untreated (control) quails were sacrificed by decapitation and exsanguination. The right femurs were cut at the center of the diaphyses and fixed in 3.7% paraformaldehyde in phosphate buffered saline (PBS) (pH 7.4) at 4℃ for 5~7 days. The bone samples were then decalcified by immersion in PBS with 10% EDTA (pH 7.4) at 4℃ for 14~21 days. This solution was changed every 3 days. After decalcification, the proximal diaphyses were dehydrated in ascending grades of ethanol and embedded in plastic (Technovit 7100 kit; Heraeus Kulzer, Germany). Transverse sections of the diaphyses (2-µm thick) mounted on glass slides (Matsunami Glass Ind., Japan) were stained with hematoxylin and eosin.
The left femurs were quickly frozen in liquid nitrogen and stored at -80℃ until RNA was extracted. The diaphyses were crushed in liquid nitrogen and total RNA was obtained using a Sepasol RNA I Super commercial extraction kit (Nacalai Tesque, Japan). cDNA was synthesized from 3 µg of total RNA using a ReverTra Ace-α first-strand cDNA synthesis kit (Toyobo, Japan) and amplified with Taq DNA polymerase (Qiagen, Germany). Primers specific for ER-α (GenBank accession No. X03805), ER-β (GenBank accession No. AF045149), and β-actin (GenBank accession No. L08165) were designed using Primer 3 as a software for primer design (NCBI, USA). Table 1 summarizes the PCR amplification conditions with the specific primer sets. PCR was performed for 25 to 36 cycles. The amplified products were subjected to electrophoresis in 1.75% agarose gels and visualized with ethidium bromide staining. Band density was determined by densitometric analysis (ATTO Densitograph; ATTO, Japan). β-actin was used as an internal control.
To examine histochemical changes in the bone marrow cavity following E2 treatment, transverse sections of femurs were stained with hematoxylin and eosin. In untreated male quails (day 0), the bone-lining cells appeared flat and were arranged on the endosteal bone surface. The bone marrow cavity was filled with bone marrow cells and adipose tissue (Fig. 1A). After E2 treatment, the bone-lining cells differentiated into cuboidal osteoblasts (day 1; Fig. 1B). These cells formed a part of the matrix between the endosteal surface and cells lining the bone (day 2; Fig. 1C). On day 3, matrices formed by the osteoblasts extended towards the bone marrow cavity and contained embedded osteocytes (Fig. 1D). These matrices were reticularly developed and many mature osteoblasts were seen on their surfaces on day 5 (Fig. 1E). Seven days after E2 treatment, volume of the matrices in the bone marrow cavity was reduced. This decrease was accompanied by an increase in osteoclasts and a decrease in osteoblasts on the surface of the matrices (Fig. 1F). After 10 days, the bone matrices were only observed in the vicinity of cortical bone while the marrow cavity began to refill with bone marrow cells and adipose tissue (Fig. 1G). After 15 days, the matrices had disappeared from the bone marrow cavity and the endosteal surface was again covered with flat bone-lining cells (Fig. 1H).
To assess the expression patterns of ER-α and ER-β mRNA during medullary bone formation and resorption, a semi-quantitative RT-PCR analysis was performed (Figs. 2 and 3). On day 0 (untreated quails), ER-α mRNA was already expressed but ER-β mRNA was barely detectable. The level of ER-α mRNA expression increased during bone formation although no significant difference was observed between days 0 and 5. Following this, ER-α mRNA expression decreased insignificantly from day 7 to day 15. ER-β mRNA was very weakly expressed throughout this period.
In the present study, we measured the temporal expression of ER-α and ER-β mRNA during medullary bone formation and resorption in male Japanese quails treated with E2. Histochemical analysis demonstrated that medullary bone was formed in the bone marrow cavity by osteoblasts derived from bone-lining cells on the endosteal surface following E2 treatment. Many osteoclasts appeared on the surface of this bone 5 days after E2 administration. Following this, medullary bone disappeared from the bone marrow cavity and bone-lining cells reappeared on the endosteal surface after 15 days. This medullary bone model is therefore useful for examining the process of bone remodeling along with the relationship between estrogen and bone metabolism. This process of bone formation and resorption has not previously been reported in an experimental mammalian model. Several studies have previously investigated the relationship between mammalian osteoblasts and estrogen. Estrogen treatment stimulates cancellous bone formation in female rats [5]. Samuels et al. [24,25] demonstrated that the bone marrow cavity is filled with bone arising from cancellous bone in the proximal metaphysis after administering high doses of estrogen to mice. In addition, many studies using ovariectomized rats have reported the prevention of bone loss by estrogen [1,13,29].
In humans, ER-α and ER-β are expressed in cortical and cancellous bone, respectively, in cells such as osteoblasts, osteocytes, and osteoclasts [2,4,7,17,23]. Thus, the two ER isoforms may have different functions in different types of bone [4]. Batra et al. [2] demonstrated that the expression of ER-α and ER-β in human bone varied according to age, gender, and cell type. Oreffo et al. [17] found that ER-α mRNA is expressed in preosteoclasts but not mature osteoclasts. On the other hand, both ER-α and ER-β are expressed in osteoblasts, osteocytes, bone-lining cells, and osteoclasts on metaphyseal trabecular bone in rodents [4,7,32]. The maturation of osteoclasts found among human peripheral blood mononuclear cells [23] as well as murine bone marrow monocytes and RAW264.7 cells [26] is directly inhibited by estrogen. Additionally, the inhibition of bone resorption by estrogen via ER-α is mediated by a reduction of human osteoclastogenesis rather than by suppressing resorptive activity [27]. These results suggest that estrogen may affect bone formation and resorption through both ER-β and ER-α. Thus, it is not clear whether one or both types of ER is involved in the effect of estrogen on bone metabolism in humans and rodents.
The presence of ERs in avian medullary bone was previously examined during the early and active periods of medullary bone formation [15,16] using an ER-α-specific antibody [28]. Consequently, ERs were found in osteogenic cells such as bone-lining cells, osteoblasts, and alkaline phosphatase-positive bone marrow cells [16]. Using in situ hybridization, Imamura et al. [8] demonstrated that osteoblasts express ER-α mRNA but not ER-β mRNA. Furthermore, a previous study we performed indicated that osteogenic cells derived from medullary bone express ER-α mRNA, but not ER-β mRNA, during bone formation [6]. These results suggest that estrogen, acting through ER-α but not ER-β, might influence medullary bone formation by osteogenic cells.
Results of the RT-PCR analysis in the present study demonstrated that ER-β mRNA levels were very low while ER-α mRNA was stably expressed at higher levels during medullary bone formation and resorption. Although these results showed that expression of ER-α mRNA increased slightly, there was no significant change throughout the bone formation period. The variation in ER-α mRNA expression patterns might be due to differences between in vivo and in vitro studies, or could be related to the isolation of osteogenic cells during active medullary bone formation. Avian osteoclasts have been shown to express ERs [18,19], but Imamura et al. [8] recently demonstrated that osteoclasts do not express either ER-α or ER-β mRNA during the active medullary bone formation period. Although the presence of ERs in osteoclasts from medullary bone is debatable, the resorption activity of osteoclasts does seem to be inhibited by estrogen, suggesting that estrogen may regulate the expression of lysosomal genes [19] and the expression level of ERs in osteoclasts [20]. Reduced numbers of osteoclasts, as well as reduced osteoblast numbers and activity, might therefore be responsible for the slight decrease in ER-α expression we observed during bone resorption. As suggested by our RT-PCR analysis of total RNA extracted from the diaphyses of femurs containing cortical bone, medullary bone, bone cells, and other bone marrow cells, estrogen may act on osteoclasts via ER-α during medullary bone resorption.
In conclusion, our results showed that estrogen might affect both the formation and resorption of medullary bone through ER-α but not ER-β. Further studies are required to clarify which cell types, osteoblasts and/or osteoclasts, express ER-α during these processes. Moreover, we found that estrogen-induced medullary bone formation in male Japanese quails is a useful model for examining the relationship between bone metabolism and estrogen.
Figures and Tables
Fig. 1
Light micrographs of transverse femur sections from E2-treated male quails taken during medullary bone formation and resorption. In the untreated quails, bone-lining cells were observed on the endosteal surface (A: day 0). These cells differentiated into cuboidal shaped osteoblasts after E2 treatment (B: day 1). Subsequently, medullary bone was formed between these osteoblasts and the endosteal surface (C: day 2), and developed reticularly towards the center of the bone marrow cavity (D: day 3). Further medullary bone development was accompanied by the embedding of osteocytes in the bone matrices (E: day 5). Thereafter, appearance of multinucleated osteoclasts on the medullary bone surface induced the reduction of these bone matrices (F: day 7 and G: day 10). This bone subsequently disappeared from the bone marrow cavity and bone-lining cells reappeared on the endosteal surface (H: day 15). Arrowheads indicate bone-lining cells on the endosteal bone surface, arrows indicate cuboidal osteoblasts, small arrows indicate osteocytes, double small arrows indicate osteoclasts, and asterisks indicate medullary bone. CB: cortical bone, H&E stain. Scale bars = 50 µm.
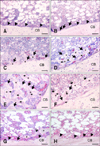
Fig. 2
Semi-quantitative RT-PCR analysis of ER-α and ER-β gene expression in femurs from E2-treated male quails. Asterisk indicates the days taken after E2 treatment, respectively.
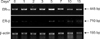
Acknowledgments
This study was supported by a grant-in-aid from the Ministry of Education, Science, Sports and Culture of Japan (18791351 in 2006-2007 to S.H.).
References
1. Andersson N, Islander U, Egecioglu E, Löf E, Swanson C, Movérare-Skrtic S, Sjögren K, Lindberg MK, Carlsten H, Ohlsson C. Investigation of central versus peripheral effects of estradiol in ovariectomized mice. J Endocrinol. 2005. 187:303–309.


2. Batra GS, Hainey L, Freemont AJ, Andrew G, Saunders PT, Hoyland JA, Braidman IP. Evidence for cell-specific changes with age in expression of oestrogen receptor (ER) α and β in bone fractures from men and women. J Pathol. 2003. 200:65–73.


3. Bloom W, Bloom MA, McLean FC. Calcification and ossification. Medullary bone changes in the reproductive cycle of female pigeons. Anat Rec. 1941. 81:443–475.


4. Bord S, Horner A, Beavan S, Compston J. Estrogen receptors α and β are differentially expressed in developing human bone. J Clin Endocrinol Metab. 2001. 86:2309–2314.


5. Chow JW, Lean JM, Chambers TJ. 17 beta-estradiol stimulates cancellous bone formation in female rats. Endocrinology. 1992. 130:3025–3032.


6. Hiyama S, Sugiyama T, Kusuhara S, Uchida T. Evidence for the expression of estrogen receptors in osteogenic cells isolated from hen medullary bone. Acta Histochem. 2009. 111:501–507.


7. Hoyland JA, Mee AP, Baird P, Braidman IP, Mawer EB, Freemont AJ. Demonstration of estrogen receptor mRNA in bone using in situ reverse-transcriptase polymerase chain reaction. Bone. 1997. 20:87–92.


8. Imamura T, Sugiyama T, Kusuhara S. Expression and localization of estrogen receptors α and β mRNA in medullary bone of laying hens. Anim Sci J. 2006. 77:223–229.


9. Krust A, Green S, Argos P, Kumar V, Walter P, Bornert JM, Chambon P. The chicken oestrogen receptor sequence: homology with v-erbA and the human oestrogen and glucocorticoid receptors. EMBO J. 1986. 5:891–897.


10. Kusuhara S, Schraer H. Cytology and autoradiography of estrogen-induced differentiation of avian endosteal cells. Calcif Tissue Int. 1982. 34:352–358.


11. Masuyama A, Ouchi Y, Sato F, Hosoi T, Nakamura T, Orimo H. Characteristics of steroid hormone receptors in cultured MC3T3-E1 osteoblastic cells and effect of steroid hormones on cell proliferation. Calcif Tissue Int. 1992. 51:376–381.


12. Miller SC, Bowman BM. Medullary bone osteogenesis following estrogen administration to mature male Japanese quail. Dev Biol. 1981. 87:52–63.


13. Mödder UIL, Riggs BL, Spelsberg TC, Fraser DG, Atkinson EJ, Arnold R, Khosla S. Dose-response of estrogen on bone versus the uterus in ovariectomized mice. Eur J Endocrinol. 2004. 151:503–510.


14. Notelovitz M. Estrogen therapy and osteoporosis: principles & practice. Am J Med Sci. 1997. 313:2–12.


15. Ohashi T, Kusuhara S. Immunoelectron microscopic detection of estrogen target cells in the bone marrow of estrogen-treated male Japanese quail. Bone Miner. 1993. 20:31–39.


16. Ohashi T, Kusuhara S, Ishida K. Estrogen target cells during the early stage of medullary bone osteogenesis: immunohistochemical detection of estrogen receptors in osteogenic cells of estrogen-treated male Japanese quail. Calcif Tissue Int. 1991. 49:124–127.


17. Oreffo ROC, Kusec V, Virdi AS, Flanagan AM, Grano M, Zambonin-Zallone A, Triffitt JT. Expression of estrogen receptor-alpha in cells of the osteoclastic lineage. Histochem Cell Biol. 1999. 111:125–133.


18. Oursler MJ, Osdoby P, Pyfferoen J, Riggs BL, Spelsberg TC. Avian osteoclasts as estrogen target cells. Proc Natl Acad Sci USA. 1991. 88:6613–6617.


19. Oursler MJ, Pederson L, Pyfferoen J, Osdoby P, Fitzpatrick L, Spelsberg TC. Estrogen modulation of avian osteoclast lysosomal gene expression. Endocrinology. 1993. 132:1373–1380.


20. Pederson L, Kremer M, Foged NT, Winding B, Ritchie C, Fitzpatrick LA, Oursler MJ. Evidence of a correlation of estrogen receptor level and avian osteoclast estrogen responsiveness. J Bone Miner Res. 1997. 12:742–752.


21. Riddle O, Rauch VM, Smith GC. Action of estrogen on plasma calcium and endosteal bone formation in parathyroidectomized pigeons. Endocrinology. 1945. 36:41–47.


22. Robinson JA, Harris SA, Riggs BL, Spelsberg TC. Estrogen regulation of human osteoblastic cell proliferation and differentiation. Endocrinology. 1997. 138:2919–2927.


23. Saintier D, Burde MA, Rey JM, Maudelonde T, de Vernejoul MC, Cohen-Solal ME. 17β-estradiol downregulates β3-integrin expression in differentiating and mature human osteoclasts. J Cell Physiol. 2004. 198:269–276.


24. Samuels A, Perry MJ, Goodship AE, Fraser WD, Tobias JH. Is high-dose estrogen-induced osteogenesis in the mouse mediated by an estrogen receptor? Bone. 2000. 27:41–46.


25. Samuels A, Perry MJ, Tobias JH. High-dose estrogen induces de novo medullary bone formation in female mice. J Bone Miner Res. 1999. 14:178–186.


26. Shevde NK, Bendixen AC, Dienger KM, Pike JW. Estrogens suppress RANK ligand-induced osteoclast differentiation via a stromal cell independent mechanism involving c-Jun repression. Proc Natl Acad Sci USA. 2000. 97:7829–7834.


27. Sørensen MG, Henriksen K, Dziegiel MH, Tankó LB, Karsdal MA. Estrogen directly attenuates human osteoclastogenesis, but has no effect on resorption by mature osteoclasts. DNA Cell Biol. 2006. 25:475–483.


28. Stossi F, Barnett DH, Frasor J, Komm B, Lyttle CR, Katzenellenbogen BS. Transcriptional profiling of estrogen-regulated gene expression via estrogen receptor (ER) α or ERβ in human osteosarcoma cells: distinct and common target genes for these receptors. Endocrinology. 2004. 145:3473–3486.


29. Turner RT, Colvard DS, Spelsberg TC. Estrogen inhibition of periosteal bone formation in rat long bones: down-regulation of gene expression for bone matrix proteins. Endocrinology. 1990. 127:1346–1351.


30. Westerlind KC, Sarkar G, Bolander ME, Turner RT. Estrogen receptor mRNA is expressed in vivo in rat calvarial periosteum. Steroids. 1995. 60:484–487.

