Abstract
The purpose of this study was to measure the thickness of canine epidermis at various anatomical sites according to localization of cornified envelopes (involucrin and filaggrin), keratins (keratin 10, 5), and their mRNA expression. This was done in the skin of five breeds of dogs including seven poodles, six golden retrievers, six Shih Tzus, four pugs, and four Labrador retrievers. Epidermal thickness of the stratum corneum and nucleated epidermal layer was significantly different. The greatest thickness was observed in the digital web area and the thinnest epidermis was in the axilla. Epidermal thickness was also significantly different between the breeds (p < 0.05). Immunohistochemical staining scores revealed significant decreases of involucrin, filaggrin, and keratin 10 in the ventral and weight-bearing sites, and a relative increase of keratin 5 (p < 0.05). q-PCR analysis showed that their the levels of mRNA were positively correlated with expression of the corresponding proteins in skin samples (p < 0.05). The present study is the first to report the relationship between epidermal gene expression and histologic morphology of the skin in normal dogs. Further studies will be essential to fully understand the pathogenesis of skin barrier dysfunctions in canines.
Skin is an essential organ that provides a protective and defensive barrier against harmful elements from the external environment such as chemical substances, microbial pathogens, and physical factors [4,8,25]. A genetic basis for skin barrier diseases has been widely postulated as a risk factor for particular skin diseases in both humans and animals [8,22,29]. The epidermal barrier is generally composed of stratum corneum (SC) and intact nucleated epidermal layers that differentiate into corneocytes of the SC as a first level of the skin barrier. Various major cross-linked proteins, which are regulated by specific genes (e.g. involucrin and filaggrin), bind to the intermediate filament keratin to form cornified envelopes (CE) [4,25,31]. Genes encoding involucrin and filaggrin belong to a large group of late cornified envelope genes associated with skin barrier dysfunctions caused by inherited genetic defects in SC proteins [2,8,15,22,23,27]. Furthermore, increase expression of these proteins occurs during barrier repair and also inhibits the differentiation of CE proteins in some diseases [15]. Disruption of intact epidermal layers allows progressive dermatological signs, which candidate epidermal genes potentially associated with SC-related barrier dysfunctions including skin dryness, atopic dermatitis, and psoriasis [7,15,27].
In the veterinary medical literature, limited information about epidermal structure and its protein components is available. Skin formation in dogs is quite different compared to humans based on histology findings such as epidermal thickness, epidermal layering, presence of secretory glands, and epidermal cell proliferation [32]. Moreover, CE proteins and keratins are possibly linked to a primary skin barrier defect in canines [22,23]. There have been a little information examining the expression of specific CE and keratin proteins in normal dogs. Thus, the purpose of this study was to evaluate the histological structure of canine skin. We performed an immunohistochemical analysis of CE proteins and keratins in the skin from dogs of different breeds with various coat types. We also developed a primer set to measure the mRNA expression of CE proteins in normal dog skin.
Twenty-seven freshly necropsied dogs were submitted to the Pathology unit, Department of Veterinary Pathology, Faculty of Veterinary Science, Chulalongkorn University (Thailand). Seven poodles, six golden retrievers, six Shih Tzus, four pugs, and four Labrador retrievers which were either euthanized or died naturally within 24 h of analysis were included in our study. All animal procedures followed the ethical guidelines established by the Chulalongkorn University Animal Care and Use Committee (Thailand). The skin of all dogs in this study appeared clinically normal and none of the animals had a history of diseases involving skin structures. Dogs with ectoparasite infestation, skin allergies, bacterial or yeast infections, hormonal imbalances, or skin tumors were excluded from this study.
Skin samples (1 × 1 cm) were obtained from each dog in 16 different regions of the body including the ear pinnae (concave and convex), perioccular, muzzle, axilla, ventral neck, ventral thorax, lateral thorax, ventral abdomen, dorsal back, lateral flank, inguinal area, perineum, fore limb, hind limb, and digital areas. The tissues were then placed into 10% neutral buffer formalin for 24 h for routine histopathologic and immunohistochemical examination. Fresh skin samples were stored in RNAlater solution (Ambion, USA) at -20℃ until analysis.
Skin samples were cut into sections (4~5 µm thickness), deparaffinized in xylene, rehydrated in graded ethanol, and then stained with hematoxylin and eosin (H&E) for routine histopathologic evaluation. For immunohistochemistry, sections were stained with a panel of primary antibodies including rabbit polyclonal antibody against filaggrin (1:2,000; Abcam, USA), mouse monoclonal antibody against involucrin (SY5, 1:1,000; Abcam, USA), and mouse monoclonal antibody against cytokeratin 10 (DE-K10, 1:600; Abcam, USA), and mouse monoclonal antibody against cytokeratin 5 (XM26, ready to use concentration; Diagnostic Biosystems, USA). Monoclonal mouse anti-human Ki-67 antibody (MIB-1; DAKO, Denmark) diluted 1:200 was used to measure epidermal proliferation. For antigen retrieval step of anti Ki-67 antibody, the slides were incubated in citrate buffer (0.01 M, pH 6.0) at 95℃ for 40 min and for others four antibodies were trypsinized by 1.0% trypsin for 15 min. Primary antibody binding was detected by a polymer-based non avidin-biotin system (EnVision; Dako, Denmark) for immunolabeling and visualized with a 3,3'-diaminobenzidine tetrahydrochloride (DAB) substrate kit (Zymed Laboratories, UK). Positive and negative control slides were prepared using normal human and canine skin with or without antibodies, respectively. The human skin sample was kindly provided by Department of Pathology, Faculty of Medicine, Siriraj Hospital, Mahidol University (Thailand). The reproducibility of these immunohistochemical procedures was performed prior study (data not shown).
Epidermal thickness was assessed by measuring total layers of epidermis, nucleated epidermis, and SC thickness in individual normal dogs with an image analysis program. Epidermal thickness was measured in 10 randomly selected fields in 16 regions of each sample. Evaluation of positively stained cells to assess protein localization was performed in the epidermis of clinically normal skin. Ten microscopic fields (×200) were randomly selected to evaluate immunostaining for the following proteins in the epidermis: involucrin, filaggrin, keratin 10, and keratin 5. The dermis, skin appendages, and blood vessels in the skin were also viewed but not analyzed. Results of the immunohistochemical staining were evaluated with an image analysis program (Image-Pro PLUS 6.0 software; Vision Machines, USA). Cytoplasmic staining intensity was divided into four different levels: 0 (negative), 1 (weak), 2 (moderate), and 3 (strong). The proportion of positive areas along with the staining intensity score represented the 'staining score'. The percentage of positive areas was determined by dividing the area with positive cytoplasmic staining (µm2) by the total epidermal area (µm2). The staining scores were derived from the summation of the staining intensity scores multiplied by the percentage of the area stained in each level divided by 100. Epidermal proliferation in each section was measured by counting the number of keratinocytes positive for Ki-67 in the epidermis of each sample. The average numbers of positive cells in the epidermis (cells per linear mm of total epidermal surface length) were determined by using Image-Pro PLUS 6.0 Programming software (Media Cybernetics, USA) for image analysis.
The 1 × 1 cm. of skin samples in RNAlater solution were extracted by homogenization with Trizol reagent (Invitrogen, USA) and a phenol/chloroform/isopropyl alcohol extraction was performed according to the manufacturer's instructions. Genomic DNA was subsequently removed from the RNA with DNase (Ambion, USA) according to the manufacturer's instructions. The DNase-treated RNA was spectrophotometrically analyzed using a NanoDrop ND-1000 Spectrophotometer V3.7 (Thermo Fisher Scientific, USA) to determine the concentration and quality of the RNA samples.
Transcript sequences of the filaggrin, involucrin, keratin were obtained from canine genome transcript sequences from NCBI (USA) for filaggrin (accession No. ENSCAFG00000023034), involucrin (accession No. M34442), keratin 10 (accession No. NM_001013425), and keratin (accession No. XM_003433511) and then crosschecked with Ensembl (UK). Primers were designed using Primer 3 software version 0.4.0 (USA) and then checked using BLAST analysis for homologies (USA). Primer pairs were evaluated for dog genome specificity (CanFam2.0, May 2005 assembly) in silico using In-Silico PCR at the University of California Santa Cruz Genome Bioinformatics Site (USA). The primer sequences, melting temperatures, and predicted product size are shown in Table 1.
RNA samples were reversely transcribed into cDNA and amplified using a SuperScript III First-strand synthesis with Platinum Taq DNA polmerase (Invitrogen, USA) according to the manufacturer's instructions. RT-PCR was performed with a 96-well GeneAmp Thermal Cycler 9700 (Applied Biosystems, USA) at 50℃ 30 min for cDNA synthesis. Amplification conditions were as follows: 95℃ for 2 min then 40 cycles of 94℃ for 15 sec, 60℃ for 30 sec, and 68℃ for 30 sec; and a final elongation at 68℃ for 5 min.
q-PCR assays were performed in triplicate using an ABI PRISM 7300 SDS (Applied Biosystems, USA) with a 96-well plate format. Each 10 µL reaction contained 1 × KAPA SYBR Fast qPCR Master Mix Universal (KAPA Biosystems, USA), 200 nM of each primer, and the 1 µL cDNA template per reaction. The thermal cycling conditions were as follows: 95℃ for 2 min for one cycle followed by 40 cycles of annealing at 95℃ for 3 sec, of extension 60℃ for 20 sec, and elongation at 72℃ for 30 sec. Standard curves were generated using the data for 10-fold serial dilutions of total RNA to confirm that the efficiency of the assay was between 97% and 110%, and the R2 value was greater than 0.98. Prior to the present study, a suitable reference gene has been assessed. The housekeeping gene RPS19, which has been previously described [3,33], was used to normalize the q-PCR data.
Statistical analyses of epidermal thickness, immunohistochemical staining, and gene expression data were performed using GraphPad Prism (ver 5.0; GraphPad Software, USA). The repeated-measures ANOVA for the difference between regions and breeds were analyzed and the significant differences were identified with a Tukey-Kramer test. Correlation analyses of mRNA and protein levels were carried out using Pearson's correlation test. p-values < 0.05 were considered statistically significant.
The skin of all different regions was composed of at least two to four well-defined nucleated epidermal layers. The canine skin was divided into four layers including the stratum (s.) basale, s. spinosum, s. granulosum, and keratinized anucleated epidermis (SC). The dermis contained a matrix of connective tissue and epidermal appendages such as hair follicles, sebaceous glands, apocrine glands, exocrine glands, and a few mononuclear cells. Thicknesses of the nucleated epidermal layers and SC in the 27 dogs were significantly different (p < 0.01) among the various body regions as shown in Fig. 1. Thickness of the digital web was the highest (mean, 37.23 ± 0.27 µm for the nucleated epidermis and 35.04 ± 0.25 µm for SC) while the lowest was in the axilla (mean, 8.76 ± 0.69 µm for the nucleated epidermis and 6.23 ± 0.16 µm for SC). Differences in total epidermal thickness between each breed were analyzed. The thickest epidermis was observed in the Labradors and thinnest was in poodles (p < 0.05). Among the five breeds (Fig. 2), there were significant differences in thickness of either nucleated or SC between each breed which SC of Labradors were greater than poodles and Shih Tzus (p < 0.05) and also their nucleated epidermal thickness were more greater than poodles, Shih tzus, and pugs (p < 0.01).
Immunohistochemical staining of involucrin and filaggrin to evaluate protein localization is shown in Fig. 3. Involucrin and filaggrin staining had a strong granular intracytoplasmic pattern in the whole layer of the nucleated epidermis. There was high expression in the s. basale and slightly reduced levels in the upper epidermal layers. Keratin 10 staining was clearly observed along the suprabasal layers of normal canine epidermis with strong staining in the cytoplasm of epidermal cells. Expression of keratin 5, a basal marker of differentiation, was obviously revealed in the basal layer of epidermis. The patterns of involucrin, filaggrin, and keratin immunohistochemical staining were similar in different skin regions. Image analysis demonstrated that the staining score for involucrin was significantly decreased in the inguinal area whereas filaggrin and keratin 10 levels were reduced in the digital web. The expression of keratin 5 was significantly increased in the muzzle and digital web (p < 0.01). Immunostaining for Ki-67, a marker of proliferative activity, was confined to the nuclei of nucleated epidermal cells. Large numbers of positive nuclei were observed in the basal layer of the muzzle, axilla, inguinal area, and digital web. No significant difference of Ki-67 between the breeds was found (p > 0.05). Staining scores for all antibodies in various regions are shown in Table 2.
As shown in Fig. 4, mRNA expression of cornified envelope genes and keratins was found in skin samples, collecting from ventral abdomen. Established primer sets specific for CE and keratin genes were effectively used to measure mRNA gene expression. RT-PCR analysis (Fig. 5) showed that the mRNA levels of each gene (involucrin, filaggrin, keratin 10, and keratin 5) corresponded to protein expression levels in all skin samples (p < 0.05). No significant differences between breeds were observed. The mRNA levels of CEs and keratins were positively correlated to expression of the corresponding protein [involucrin (r = 0.74, p = 0.012), filaggrin (r = 0.801, p = 0.005), keratin 10 (r = 0.87, p = 0.020), and keratin 5 (r = 0.58, p = 0.045)].
This study measured the epidermal thickness in the skin from various regions in normal dogs of several breeds with different coat types. The SC thickness at the inguinal fold and the back was significant difference that agreed to Lloyd and Garthwaite [20]. While, the thickness of nucleated epidermis was shown significantly difference, in contrast to previous reports, that there were no significant difference in the thickness between skin areas and hair coat by measuring epidermal layers [1] or by areas (mm2) [32] as well as by direct measurement [20]. However, due to the limitation of this present study, the small number of sample sizes or dog breeds might be associated to the skin morphology and thickness. In the present study, dogs of five different breeds with various skin coats were examined. Pugs are categorized as having a short coat and Labrador retrievers were considered to have medium length coats [17] which had the thickest epidermis among all the breed with different coat types studied. Dogs with long coats and no undercoat include poodles and Shih Tzus, whereas golden retrievers have long coats with a dense undercoat [17] that possessed thinner epidermis than the short coat one. As stated in a previous report, different coat types have variations in hair follicle cycles and quality [17]. And, the dogs with long coats and no undercoat rarely shed [10]. Therefore, it is suggested that epidermal thickness in these dogs might be less affected than in the dogs with shedding coat more frequently. Areas of skin with thin or lacking hair are easily exposed or sensitively come into contacted with the external environment. Subsequently, the epidermal layer in this skin has adapted by increasing cell proliferation and the mechanisms underlying canine skin thickening possibly varies by breed or coat types. Thus, breeds, coat types and the skin morphology would be the predisposing causes on skin pathology.
Immunohistochemical staining of the CE proteins, involucrin and filaggrin in normal dog skin from different regions was performed in the present study. Filaggrin-specific staining had a fine granular-like intracytoplasmic pattern in all nucleated cell in the epidermal layers of all skin specimens. This corresponds to the results of a previous study that evaluated filaggrin expression in normal healthy beagle dogs [23] and also showed that staining for this protein produces a discrete granular pattern in all nucleated epidermal layers. The staining pattern of filaggrin in normal human skin has been previously reported in the upper granular layers and SC, and it was found that the arrangement of filaggrin in humans is different than that in dogs [5,15]. It has also been found that normal dog skin has less intercellular matrix than other species, resulting in a thin epidermis with dense epidermal layers [20]. Therefore, it could be hypothesized that CE proteins in the dog epidermis would possibly have biochemical characteristics different from those in other species.
Involucrin, which is an essential cell envelope component, formed an unmistakable granular intracytoplasmic pattern in all nucleated cells of the epidermal layer in normal canine epidermis. Our finding is in contrast to those of a previous report that involucrin expression was not detected [18]. Cloning and sequencing of the involucrin gene from beagle dogs have been previously reported [30]. Antibodies against human involucrin also cross-react with antigenic epidermal proteins in dogs [19]. However, the involucrin staining pattern, we observed in dogs was different from that in humans as reported in the literature showing that human involucrin is diffusely distributed in the upper spinous layers and s. granulosum [15]. Differences in staining patterns between humans and dogs might be influenced by the skin-differentiation process. In addition, staining scores for both involucrin and filaggrin calculated in the present study were decreased in some areas, especially the ventral side and weight-bearing site. From these reasons, the CE might involve skin barrier defects or alteration of differentiation at the affected areas.
Many studies have been conducted on increased transepidermal water loss (TEWL) associated with decreased ceramide content in cases of canine atopic dermatitis [26,28]. Analysis of skin samples taken from the ventral areas (e.g., the inguinal areas and caudal abdomen) revealed increases in TEWL at these sites along with reduced levels of epidermal lipids. Similar to findings from the present study, the levels of CE proteins were also found to have decreased staining scores at the sites evaluated. Therefore, it should be noted that reduction of these CE proteins indicated relatively decreased to the amount of other CEs involved compartments (e.g., ceramide and the organization of keratins). There was also evidence of alteration of these substrates with canine skin barrier diseases such as atopic dermatitis. Involucrin and filaggrin functioning during the late stage of skin differentiation causes aggregation of keratin intermediated filaments and combination with binding lipids (e.g., ceramides) in the skin barrier. Configurations of these CE proteins vary with structure charges, sequence, and amino acid composition of the components [4,21,25,34]. Thus, involucrin and filaggrin represent a group of proteins rather than individual entities. For these reasons, the antibodies that can react with different epitopes should be created and specified to filaggrin and involucrin.
Keratin 10 levels were decreased in the digital areas whereas, a relative increased of keratin 5 presenting in muzzle, perineum and digital areas. There were significantly reduced of keratin and related to a dryness skin in aged humans [7,9]. In dogs, the mutated keratins have been reported that affected to the inherited keratinizing skin disease including, epidermolytic hyperkeratosis and epidermolytic ichthyosis [6,24]. Ki-67 is a proliferative marker that is responsible for epidermal regeneration in the basal layer through mitotic figures. The number of Ki-67-positive epidermal cells increased in areas with significant difference in staining scores for involucrin, filaggrin, keratin 10, and keratin 5. Our findings suggest that these areas have alterations in proteins involved in skin dryness and formation. Hence, increasing epidermal cell proliferation will compensate to the structure of skin, and possibly the alteration of CE proteins and keratins.
The mRNA expression of involucrin, filaggrin, keratin 10, and keratin 5 was examined by RT-PCR. In this study, q-PCR showed high degrees of sensitivity and accuracy for measuring the expression of these genes. Immunohistochemistry has confirmed the PCR results on the protein level, showing that these methods are similar to previous dermatological studies [12,13,16]. mRNA levels and protein expression corresponded in our current study. It is interesting that alterations of both expression levels were remarkably changed in some skin regions in our study. Therefore, we suggest that these areas are possibly susceptible to specific skin disorders such as canine allergic skin diseases [11,14]. In conclusion, we successfully developed primers specific for four genes, involucrin,filaggrin, keratin 10 and keratin 5, based on cDNA sequences to measure expression in dog skin. These sets of primers could be useful for quantitative determination of mRNA and protein levels in further molecular studies of skin diseases in dogs. This study is the first report on the relationship of the CE gene expression and histologic morphology of the skin in normal dog. Additionally, the epidermal structure, thickness of skin from dogs of different regions, breeds, and coat types are suggested to be the predisposing causes on susceptibility of skin diseases. The obtained results could be useful informations for the better understanding on pathogenesis of skin barrier dysfunction such as canine atopic dermatitis and possibly used as an animal model in human skin diseases.
Acknowledgments
The authors thank the Office of the Higher Education Commission, Thailand for supporting the Strategic Scholarships for Frontier Research Network for the Join Ph.D. Program Thai Doctoral degrees and the Faculty of Veterinary Science, Chulalongkorn University Research Fund (2010). Additionally, the authors thank Dr. Komkrid Charngkaew, Department of Pathology, Faculty of Medicine, Siriraj Hospital, Mahidol University, for kindly provide skin tissue and technical assistance, and Assist. Prof. Dr. Sayamon Srisuwatanasagul, Department of Anatomy, Faculty of Veterinary Science, Chulalongkorn University, Thailand for her kind help on image analysis.
References
1. Auxilia ST, Hill PB. Mast cell distribution, epidermal thickness and hair follicle density in normal canine skin: possible explanations for the predilection sites of atopic dermatitis? Vet Dermatol. 2000; 11:247–254.


2. Barnes KC. An update on the genetics of atopic dermatitis: scratching the surface in 2009. J Allergy Clin Immunol. 2010; 125:16–29. PMID: 20109730.


3. Brinkhof B, Spee B, Rothuizen J, Penning LC. Development and evaluation of canine reference genes for accurate quantification of gene expression. Anal Biochem. 2006; 356:36–43. PMID: 16844072.


4. Candi E, Schmidt R, Melino G. The cornified envelope: a model of cell death in the skin. Nat Rev Mol Cell Biol. 2005; 6:328–340. PMID: 15803139.


5. Chervet L, Galichet A, McLean WHI, Chen H, Suter MM, Roosje PJ, Müller EJ. Missing C-terminal filaggrin expression, NFkappaB activation and hyperproliferation identify the dog as a putative model to study epidermal dysfunction in atopic dermatitis. Exp Dermatol. 2010; 19:e343–e346. PMID: 20626465.


6. Credille KM, Barnhart KF, Minor JS, Dunstan RW. Mild recessive epidermolytic hyperkeratosis associated with a novel keratin 10 donor splice-site mutation in a family of Norfolk terrier dogs. Br J Dermatol. 2005; 153:51–58. PMID: 16029326.


7. Ekanayake-Mudiyanselage S, Aschauer H, Schmook FP, Jensen JM, Meingassner JG, Proksch E. Expression of epidermal keratins and the cornified envelope protein involucrin is influenced by permeability barrier disruption. J Invest Dermatol. 1998; 111:517–523. PMID: 9740250.
8. Elias PM, Steinhoff M. "Outside-to-inside" (and now back to "outside") pathogenic mechanisms in atopic dermatitis. J Invest Dermatol. 2008; 128:1067–1070. PMID: 18408746.


9. Engelke M, Jensen JM, Ekanayake-Mudiyanselage S, Proksch E. Effects of xerosis and ageing on epidermal proliferation and differentiation. Br J Dermatol. 1997; 137:219–225. PMID: 9292070.


10. Favarato ES, Conceição LG. Hair cycle in dogs with different hair types in a tropical region of Brazil. Vet Dermatol. 2008; 19:15–20. PMID: 18177286.


11. Griffin CE, DeBoer DJ. The ACVD task force on canine atopic dermatitis (XIV): clinical manifestations of canine atopic dermatitis. Vet Immunol Immunopathol. 2001; 81:255–269. PMID: 11553388.


12. Gu X, Lundqvist EN, Coates PJ, Thurfjell N, Wettersand E, Nylander K. Dysregulation of TAp63 mRNA and protein levels in psoriasis. J Invest Dermatol. 2006; 126:137–141. PMID: 16417229.


13. Gu X, Nylander E, Coates PJ, Nylander K. Effect of narrow-band ultraviolet B phototherapy on p63 and microRNA (miR-21 and miR-125b) expression in psoriatic epidermis. Acta Derm Venereol. 2011; 91:392–397. PMID: 21373745.
14. Jaeger K, Linek M, Power HT, Bettenay SV, Zabel S, Rosychuk RAW, Mueller RS. Breed and site predispositions of dogs with atopic dermatitis: a comparison of five locations in three continents. Vet Dermatol. 2010; 21:118–123. PMID: 20187918.


15. Jensen JM, Fölster-Holst R, Baranowsky A, Schunck M, Winoto-Morbach S, Neumann C, Schütze S, Proksch E. Impaired sphingomyelinase activity and epidermal differentiation in atopic dermatitis. J Invest Dermatol. 2004; 122:1423–1431. PMID: 15175033.


16. Kim BE, Leung DYM, Boguniewicz M, Howell MD. Loricrin and involucrin expression is down-regulated by Th2 cytokines through STAT-6. Clin Immunol. 2008; 126:332–337. PMID: 18166499.


17. Kirzeder EM, Frank LA, Sowers KD, Rohrbach BW, Donnell RL. Influence of inflammation and coat type on oestrogen receptor immunohistochemistry. Vet Dermatol. 2008; 19:264–270. PMID: 18927952.


18. Kozaki M, Nakamura Y, Iguchi M, Kano R, Watanabe S, Fujiwara K, Hasegawa A. Immunohistochemical analysis of cytokeratin expression in dog skin. J Vet Med Sci. 2001; 63:1–4. PMID: 11217052.


19. Kubilus J, Phillips SB, Goldaber MA, Kvedar JC, Baden HP. Involucrin-like proteins in non-primates. J Invest Dermatol. 1990; 94:210–215. PMID: 1688904.


20. Lloyd DH, Garthwaite G. Epidermal structure and surface topography of canine skin. Res Vet Sci. 1982; 33:99–104. PMID: 7134655.


21. Mack JW, Steven AC, Steinert PM. The mechanism of interaction of filaggrin with intermediate filaments. The ionic zipper hypothesis. J Mol Biol. 1993; 232:50–66. PMID: 7687298.
22. Marsella R, Olivry T, Carlotti DN; International Task Force on Canine Atopic Dermatitis. Current evidence of skin barrier dysfunction in human and canine atopic dermatitis. Vet Dermatol. 2011; 22:239–248. PMID: 21414049.


23. Marsella R, Samuelson D, Harrington L. Immunohistochemical evaluation of filaggrin polyclonal antibody in atopic and normal beagles. Vet Dermatol. 2009; 20:547–554. PMID: 20178493.


24. Mecklenburg L, Hetzel U, Ueberschär S. Epidermolytic ichthyosis in a dog: clinical, histopathological, immunohistochemical and ultrastructural findings. J Comp Pathol. 2000; 122:307–311. PMID: 10805985.
25. Proksch E, Brandner JM, Jensen JM. The skin: an indispensable barrier. Exp Dermatol. 2008; 17:1063–1072. PMID: 19043850.


26. Reiter LV, Torres SMF, Wertz PW. Characterization and quantification of ceramides in the nonlesional skin of canine patients with atopic dermatitis compared with controls. Vet Dermatol. 2009; 20:260–266. PMID: 19659537.


27. Sandilands A, Sutherland C, Irvine AD, McLean WHI. Filaggrin in the frontline: role in skin barrier function and disease. J Cell Sci. 2009; 122:1285–1294. PMID: 19386895.


28. Shimada K, Yoon JS, Yoshihara T, Iwasaki T, Nishifuji K. Increased transepidermal water loss and decreased ceramide content in lesional and non-lesional skin of dogs with atopic dermatitis. Vet Dermatol. 2009; 20:541–546. PMID: 20178492.


29. Sugarman JL. The epidermal barrier in atopic dermatitis. Semin Cutan Med Surg. 2008; 27:108–114. PMID: 18620132.


30. Tseng H, Green H. The involucrin genes of pig and dog: comparison of their segments of repeats with those of prosimians and higher primates. Mol Biol Evol. 1990; 7:293–302. PMID: 2385171.
31. Wickett RR, Visscher MO. Structure and function of the epidermal barrier. Am J Infect Control. 2006; 34(Suppl):S98–S110.
32. Wilkie JSN, Yager JA, Eyre P, Parker WM. Morphometric analyses of the skin of dogs with atopic dermatitis and correlations with cutaneous and plasma histamine and total serum IgE. Vet Pathol. 1990; 27:179–186. PMID: 2353419.


33. Wood SH, Clements DN, McEwan NA, Nuttall T, Carter SD. Reference genes for canine skin when using quantitative real-time PCR. Vet Immunol Immunopathol. 2008; 126:392–395. PMID: 18824265.


34. Yaffe MB, Beegen H, Eckert RL. Biophysical characterization of involucrin reveals a molecule ideally suited to function as an intermolecular cross-bridge of the keratinocyte cornified envelope. J Biol Chem. 1992; 267:12233–12238. PMID: 1601889.


Fig. 1
Total epidermal thickness according to stratum corneum (SC) and nucleated epidermal layers (µm) from 16 regions in 27 dogs. Solid lines and dash lines indicate significant differences in SC and nucleated epidermal layer, respectively. Data are expressed as the mean ± SD (*p < 0.05 and **p < 0.01).
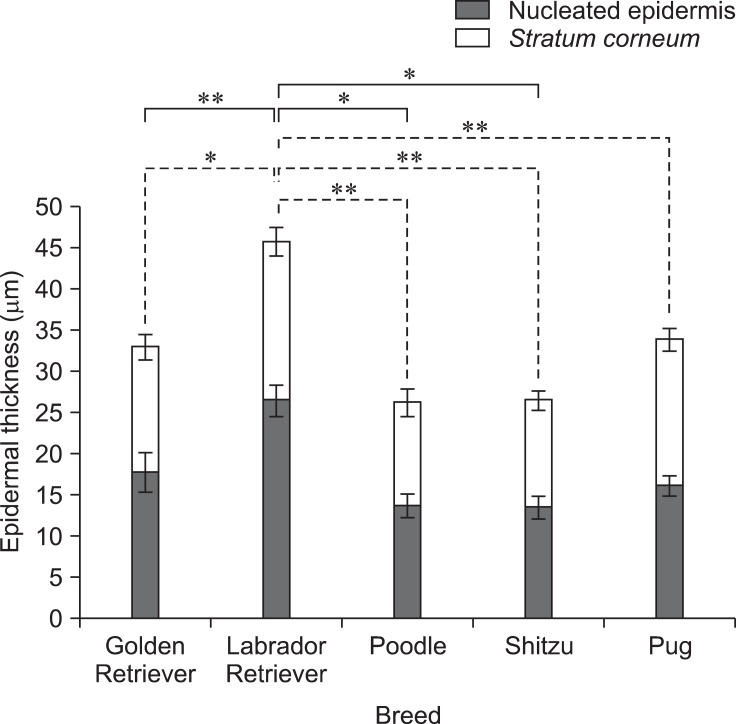
Fig. 2
The average total epidermal thickness of all skin samples in five different breeds of dogs. Solid lines and dash lines indicate significant differences in SC and nucleated epidermal layer, respectively. Data are expressed as the mean ± SD (*p < 0.05 and **p < 0.01).
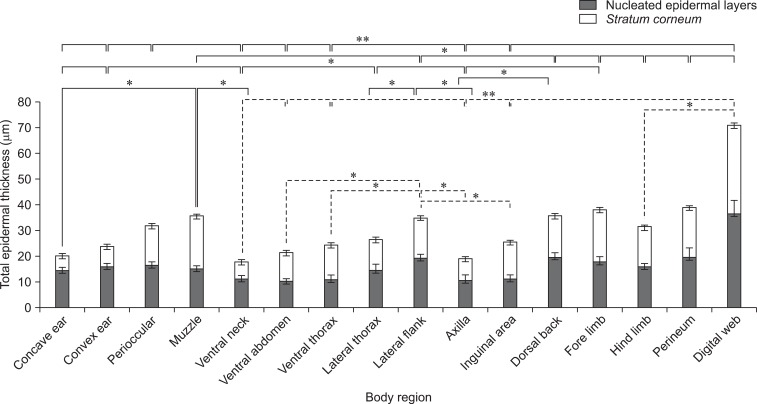
Fig. 3
Immunohistochemical localization of various proteins expressed in the epidermis of normal dog skin from the abdominal areas. A: involucrin, B: filaggrin, C: keratin 10, D: keratin 5. DAB and Meyer's hematoxylin counterstain, ×200. Scale bars = 25 µm.
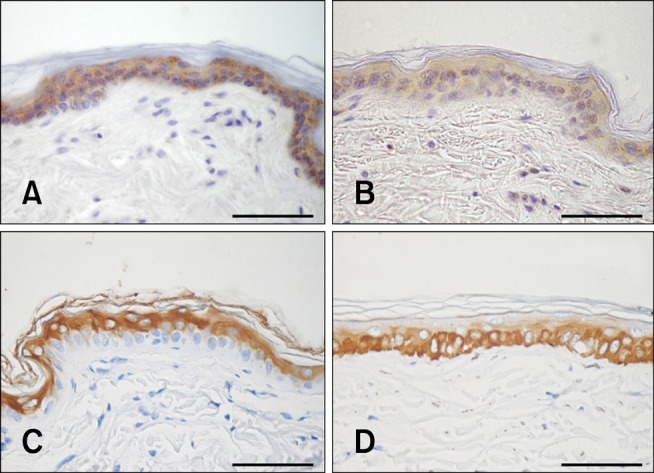
Fig. 4
mRNA expression of involucrin, filaggrin, keratin 10, and keratin 5 in abdominal skin from five clinically normal poodles (lane 1~5). Lane M: 100 bp DNA ladder.

Fig. 5
mRNA was collected from the skin of dogs. The levels of involucrin, filaggrin, keratin 10, and keratin 5 expression were then evaluated by RT-PCR. Different symbols (*for involucrin, †for filaggrin, ‡for keratin 10, and §for keratin 5) indicate significant differences (p < 0.05) of each target gene.
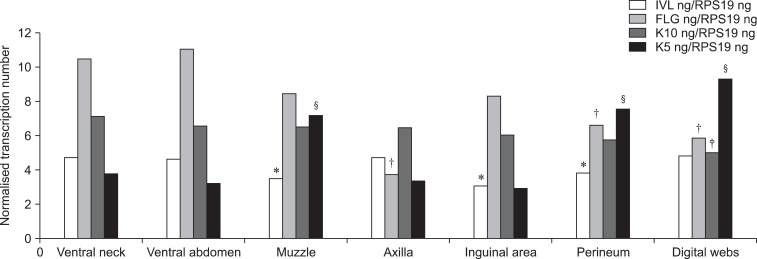