Abstract
Object recognition memory and contextual fear conditioning task performance in adult C57BL/6 mice exposed to cranial fast neutron irradiation (0.8 Gy) were examined to evaluate hippocampus-related behavioral dysfunction following acute exposure to relatively low doses of fast neutrons. In addition, hippocampal neurogenesis changes in adult murine brain after cranial irradiation were analyzed using the neurogenesis immunohistochemical markers Ki-67 and doublecortin (DCX). In the object recognition memory test and contextual fear conditioning, mice trained 1 and 7 days after irradiation displayed significant memory deficits compared to the sham-irradiated controls. The number of Ki-67- and DCX-positive cells decreased significantly 24 h post-irradiation. These results indicate that acute exposure of the adult mouse brain to a relatively low dose of fast neutrons interrupts hippocampal functions, including learning and memory, possibly by inhibiting neurogenesis.
Radiation induces various types of damage in human and animals. Among the different radiation sources, high-linear energy transfer (LET) neutrons are several times more effective than low-LET X- and gamma (γ)-radiation for specifically damaging tissues [3]. A main cause of cell death induced by high-LET radiation is non-reparable double strand DNA breaks which are produced at a higher rate compared to low-LET irradiation [3]. In humans, exposure to neutrons can occur during nuclear fission reactions usually associated with the production of nuclear energy and cosmic radiation in the environment [1,2,5]. Consequently, it is very important to study the direct effects of neutrons on human organs and tissues to precisely ascertain the risk of high-LET radiation exposure. High-LET components, especially neutrons, contribute principally to this risk so that even small doses of neutron become magnified in the serious effects of radiation exposure [4].
Ionizing radiation has a variety of effects on the brain. Even though the adult brain is less vulnerable to irradiation than other organs, less tissue injury can occur after relatively low-dose irradiation with low-LET γ-rays, resulting in varying degrees of cognitive impairment in adult mammals [7,8,14]. Radiation-induced cognitive deficits are associated with inhibition of hippocampal neurogenesis in adult animals [7,8,13]. A recent study compared the level of neural progenitor cell death in mouse hippocampus following exposure to high-LET fast neutrons with low-LET γ-rays per unit of absorbed dose [15]. Exposure to high-LET iron-56-particle radiation suppresses hippocampal neurogenesis and promotes the development of cognitive deficits in rodents [9,11,12]. However, the behavioral aspect and precise mechanism underlying the effects of relatively low doses of high-LET fast neutrons on experimental animals remain poorly understood.
The purpose of this study was to evaluate hippocampus-related behavioral dysfunction following acute exposure to relatively low doses of fast neutrons. For this, we measured the object recognition memory and contextual fear conditioning task performance of adult C57BL/6 mice exposed to cranial fast neutron irradiation (0.8 Gy). In addition, neurogenesis in the dentate gyrus (DG) of hippocampi from adult C57BL/6 mice treated with cranial fast neutron irradiation (0.8 Gy) was evaluated using two immunohistochemical markers of neurogenesis, Ki-67 (a proliferating cell marker) and doublecortin (DCX; an immature progenitor cell marker).
Eight-week-old male C57BL/6 mice were obtained from Orient Bio. (Korea). The mice were fed a standard animal diet (Samyang Feed, Korea). All animal experiments followed a protocol approved by the Committee for Animal Experimentation at Chonnam National University, Korea.
Fast neutrons were generated by the Korea Cancer Center Hospital (Korea) cyclotron using a proton-beryllium reaction. The estimated forward neutron spectrum had a peak energy of 35 MeV. For fast neutrons, the mean dose rate was 94 mGy/min. The irradiation doses were measured with an IC-17 ionization chamber (Far West Technology, USA) and a RDM 2A electrometer (Therados, Sweden).
Behavioral dysfunction of the mice was evaluated after cranial irradiation with 0.8 Gy of fast neutrons by open field analysis (n = five mice/group), object recognition memory test (n = seven mice/group), and contextual fear conditioning (n = seven mice/group) on 1, 7, and 14 days post-irradiation (p.i.). Sham-irradiated control mice were also transported to the irradiation facility but were not irradiated (1 day p.i.).
To observe the detrimental effect of fast neutrons on neurogenesis in adult hippocampi, the mice were cranially irradiated with 0.8 Gy of fast neutrons. The mice were sacrificed 24 h later and hippocampi from the mice in each group were removed (n = three mice/group). Samples of the hippocampi were fixed in 4% paraformaldehyde (Sigma-Aldrich, USA) in phosphate buffered saline (PBS, pH 7.4) and embedded in paraffin for histological analysis.
Open field analysis was used to measure the activity of the mice in a novel environment. Total moving distance (cm), movement time (sec), number of movement episodes, and rest time (sec) were determined over 5 min using the TruScan Photo Beam Activity System (Coulbourn Instruments, USA).
Eight-week-old mice were acclimated to the training/testing acryl chamber (42 cm length, 28 cm width, and 20 cm height) for 24 h. The objects used for recognition testing were made from plastic and had three different shapes: cubes, pyramids, and cylinders. Each object was 3.5 cm high and could not be displaced by the mice. The chamber area and objects were cleaned with 75% ethanol between trials to prevent the buildup of olfactory cues. During training, two randomly selected objects with different shapes were presented to each mouse for 10 min. At 24 h after training, another set of objects (one previously presented object and one novel object) was presented to the trained mice. For example, if the cube- and pyramid-shaped objects were presented during training, the cylinder-shaped object was used as a novel object during testing. Interactions of the mouse with each object, including approaches and sniffing, were scored [7,10,16,17]. If the mouse retained the memory of a previously encountered object, it would show preference for the novel object during testing. The percentage preference was defined as the number of interactions with a specific object divided by the total number of interactions with both objects.
During training for fear conditioning, mice were placed in an operant chamber with a metal grid floor (TruScan chamber with photobeam sensors; Coulbourn Instruments, USA) for 30 sec after which a mild electric foot shock was delivered (0.3 mA for 1 sec). At 24 h after training, mice were tested and scored to determine the percentage of freezing behavior (defined as immobility except for breathing) every 2 sec for 2 min.
Coronal sections (5 µm-thick) were cut using a microtome (SM2010R; Leica, Germany) and deparaffinized using routine protocols before being incubated citrate buffer (0.01 M, pH 6.0) and heated in an autoclave for 10 min. All subsequent steps were performed at room temperature. The sections were treated with 0.3% hydrogen peroxide in methyl alcohol for 20 min to block endogenous peroxidase activity. After three washes with PBS, the sections were blocked with 10% normal goat serum (Vector Laboratories, USA) in PBS for 1 h and then incubated for 2 h with immunohistochemical markers for proliferating cells (monoclonal rabbit anti-Ki-67 antibody, 1 : 200 dilution; Acris Antibodies GmbH, Germany) and immature progenitor cells (polyclonal rabbit anti-DCX antibody, 1 : 400 dilution; Cell Signaling Technology, USA). After three washes in PBS, the sections were incubated with a 1 : 100 dilution of biotinylated goat anti-rabbit IgG (Vector Laboratories, USA) for 45 min. After three washes in PBS, the sections were incubated for 45 min with an avidin-biotin peroxidase complex (Vector Laboratories, USA) prepared according to the manufacturer's instructions. The sections were then washed three times in PBS, and antibody binding was detected by incubating for 3 min in a diaminobenzidine substrate (Vector Laboratories, USA) prepared according to the manufacturer's instructions. As a negative control, the primary antibodies were omitted when processing a few test sections from each experiment. After completion of color development, the sections were counterstained with Harris's hematoxylin (Sigma-Aldrich, USA) for 20 sec, washed in running tap water for 20 min, dehydrated by incubating in a graded series of ethanol solutions, washed with xylene, and mounted with Canadian balsam (Sigma-Aldrich, USA).
The numbers of cells showing the specific characteristics of proliferating (immunopositive for Ki-67) and immature progenitor (immunopositive for DCX) cells in the hippocampus were scored using a histomorphometric approach [6,7,10,16,17]. The observer was blind to the sample identities. Brain samples from each mouse were taken approximately 2.12 mm caudal to the bregma. For each mouse, a standardized counting area included the DG regions in three 5 µm-thick coronal sections chosen from a one-in-ten series of sections representing the rostral/mid-hippocampus. One coronal section was chosen from each of the three regions of the hippocampus (about 50 µm apart) such that the sections did not overlap. All positively immunolabeled cells within the subgranular zone of the supra- and infra-pyrimidal blades of the DG were counted. The number of immunopositive cells was averaged across the DG region in each of the three brain sections so that the mean number of immunopositive cells in the three sections of each mouse was n = 1.
The basal locomotor activity of sham-irradiated controls and mice 1, 7, and 14 days p.i. was examined in a novel environment using open field analysis (n = five in each group) because different levels of anxiety can affect the motivation and performance in learning and memory tests. There were no significant differences in movement distance (p = 0.854 [1 day], p = 0.521 [7 days], and p = 0.922 [14 days] vs. the sham-irradiated controls), movement time (p = 0.741 [1 day], p = 1.000 [7 days], and p = 0.587 [14 days] vs. the sham-irradiated controls), movement episodes (p = 0.158 [1 day], p = 0.347 [7 days], and p = 0.129 [14 days] vs. the sham-irradiated controls), and resting time (p = 0.741 [1 day], p = 1.000 [7 days], and p = 0.587 [14 days] vs. the sham-irradiated controls) between the sham-irradiated controls and mice 1, 7 and 14 days p.i. All mice had comparable moving distances, movement times and episodes, and resting times (Table 1). This suggested that acute cranial irradiation with 0.8 Gy of fast neutrons did not alter basal locomotor activity that can contribute to potential differences in hippocampus-related learning and memory behavior test results.
Mice (n = seven in each group) were examined using the object recognition memory sensitive hippocampus-dependent paradigm [7,10,16,17]. The sham-irradiated controls and mice examined 1, 7, and 14 days after acute irradiation displayed an equal preference for a pair of two objects with different shapes during training (Fig. 1A). Object recognition memory deficits were evident during testing in mice trained 1 day (p < 0.01 vs. the sham-irradiated controls) and 7 days (p < 0.05 vs. the sham-irradiated controls) p.i. whereas the mice trained 14 days p.i. did not show any object recognition memory deficit (Fig. 1B). During the test, preferences (mean ± SE) for a novel object were 68.6 ± 1.9% for the sham-irradiated controls, 57.6 ± 2.2% for the mice trained 1 day p.i., 56.0 ± 4.9% for mice trained 7 days p.i., and 64.6 ± 2.7% for mice trained 14 days p.i. There was no significant difference in the total number of interactions during training between the sham-irradiated control and mice examined on 1 (p = 0.105), 7 (p = 0.568), and 14 (p = 0.718) days p.i. (Table 2). This suggests that all mice had comparable levels of attention, motivation, and visual perception.
Contextual fear conditioning, another hippocampus-related learning paradigm [10], was used to examine seven mice in each group. During training, both sham- and fast neutron-irradiated mice displayed minimal freezing (Fig. 2). Sensitivity to electric foot-shock was further tested and no significant differences were observed in the threshold current required to elicit stereotypic responses, including flinching, vocalization, and jumping/vocalization, between the sham- and fast neutron-irradiated mice (data not shown). This finding suggests that the mice had comparable levels of sensitivity to the electric foot-shock. The memory retention trials (testing) were carried out 24 h after the acquisition trial (training). Sham-irradiated mice displayed a significant increase in freezing (61.3 ± 6.2%; mean ± SE) when tested 24 h after training (1 day after sham irradiation with 0 Gy; Fig. 2). The mice trained 1 and 7 days p.i. displayed significantly lower levels of freezing during the test (1 day p.i., 35.2 ± 5.8%, p < 0.01 vs. the sham-irradiated control; 7 days p.i., 26.3 ± 2.8%, p < 0.001 vs. the sham-irradiated control; Fig. 2). However, the mice trained 14 days p.i. did not show a significant deficit in contextual fear conditioning (Fig. 2).
Ki-67- (Figs. 3A and B; 19.0 ± 1.5 cells/DG in three mice) and DCX-positive cells (Figs. 3C and D; 62.7 ± 6.7 cells/DG in three mice) were consistently observed in the hippocampal DG of the adult control mice. The number of Ki-67-positive cells in the DG markedly declined 24 h following irradiation with 0.8 Gy of fast neutrons (3.7 ± 0.7 cells/DG, p < 0.001 vs. the sham-irradiated control; Figs. 3A and B). In comparison, the number of DCX-positive cells in the DG declined 24 h p.i. (18.3 ± 1.5 cells/DG, p < 0.01 vs. the sham-irradiated control; Figs. 3C and D).
The present study demonstrated that mice trained 1 and 7 days after acute exposure to a relatively low dose of fast neutrons (0.8 Gy) exhibited significant memory deficits in two hippocampus-related learning paradigms: object recognition memory testing and contextual fear conditioning. In addition, decreases in the level of neurogenesis were evident in the DG 24 h p.i. following irradiation with 0.8 Gy of fast neutrons, indicating a correlation between hippocampal neurogenesis and cognitive impairment caused by cranial fast neutron irradiation.
Radiation has deleterious effects on the adult brain. Neural progenitor cells in the DG of the adult hippocampus are particularly vulnerable to irradiation which may impair hippocampus-dependent learning and memory [7]. Relatively low doses of irradiation with low-LET γ-rays results in varying degrees of cognitive impairment in adult mammals [7,8,14]. In comparison, high-LET iron-56-particle radiation also suppresses hippocampal neurogenesis and promotes cognitive deficit in rodents [9,11,12]. However, little is known about the detrimental effects of high-LET fast neutrons on hippocampal functions in adult animals. Therefore, it is important to clarify the behavioral aspects associated with and precise mechanisms underlying the effects of relatively low doses of high-LET fast neutrons on experimental animals, and the vulnerability of neural progenitor cells of the adult hippocampus DG to relatively low doses of fast neutrons.
The doses of irradiation needed to kill proliferating progenitor cells in an adult brain without inducing serious short-term side effects has been reported [7,13]. A previous study showed that a 10 Gy dose of X-rays results in significant and prolonged loss of DG proliferating cells and their progeny 3~4 months after irradiation in adult mice [8]. However, a recent study reported that 0.5~4.0 Gy of γ-irradiation produces few changes in the hippocampal structure in adult mice. However, the amount of γ-irradiation altered hippocampal neurogenesis in the mice, and reduced hippocampal neurogenesis temporally correlated with hippocampal-dependent memory retention deficits. Furthermore, recovered hippocampal neurogenesis correlated with the recovery of hippocampal-dependent memory retention [7]. In the current study, irradiation with a relatively low dose (0.8 Gy) of fast neutrons had a reversible effect on two hippocampus-related learning paradigms, object recognition memory testing and contextual fear conditioning, in adult mice. Our findings suggest that this model is suitable for studying short-term cognitive impairment caused by transient inhibition of hippocampal neurogenesis. However, more studies are needed to identify an unequivocal link between changes in hippocampal neurogenesis and behavior. Furthermore, since other brain regions were not evaluated in this analysis, the possibility remains that neural changes in other brain regions may contribute to the fast neutron-induced cognitive impairment observed in the present study.
In conclusion, our data showed that exposure of adult mouse brain to high-LET fast neutrons causes hippocampus-related memory dysfunction, possibly by reducing the rate of hippocampal neurogenesis. These findings suggest that this type of irradiation can transiently interrupt hippocampus functioning, including learning and memory, in adult mice.
Figures and Tables
Fig. 1
Acute fast neutron irradiation transiently decreases object recognition memory in mice. The sham-irradiated controls (1 day after sham-irradiation) and mice 1, 7, and 14 days after acute irradiation with 0.8 Gy of fast neutrons were examined (n = seven for each group). During training, two objects were presented to each mouse for 10 min. After 24 h, one of the previously presented objects was replaced with a novel object (testing). If the mouse remembered the re-presented object, we hypothesized that it would spend more time with the novel object during testing as indicated by a higher percentage of object preference. (A) The sham-irradiated controls and mice 1, 7, and 14 days after irradiation showed equal preference for the two objects during training. (B) During testing, sham-irradiated control and mice trained 14 days after irradiation exhibited a significant preference for the novel object. A significant difference in novel object preference was found between sham-irradiated controls and mice trained 1 and 7 days post-irradiation (p.i). Data are reported as the mean ± SE. *p < 0.05 and **p < 0.01 vs. the sham-irradiated controls.
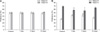
Fig. 2
Irradiated mice display a transient deficit in contextual fear conditioning. Sham-irradiated controls (1 day after sham-irradiation) and mice examined 1, 7, and 14 days after acute irradiation with 0.8 Gy of fast neutrons underwent contextual fear conditioning (n = seven for each group). The freezing behavior of the mice was evaluated 24 h after training. Mice trained 1 and 7 days p.i. exhibited lower levels of learning and memory formation than the sham-irradiated controls as indicated by significantly lower rates of freezing during testing. Data are reported as the mean ± SE. **p < 0.01 and ***p < 0.001 vs. sham-irradiated controls.
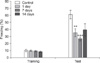
Fig. 3
Representative images showing Ki-67-positive proliferating cells and DCX-positive immature progenitor cells in the adult hippocampus dentate gyrus (DG) after fast neutron irradiation. Ki-67 (panels A and B) and DCX immunoreactivity (panels C and D) in the subgranular zone of the DG decreased after exposure to 0.8 Gy of fast neutrons. GCL: granular cell layer. Scale bars = 40 µm.
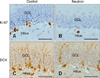
Table 1
Open-field analysis of mice 1, 7, and 14 days after acute exposure to fast neutrons in a novel environment

Acknowledgments
This work was supported by a National Research Foundation (NRF) of Korea grant (2010-0017537) funded by the Korean government and a grant from the Korean Ministry of Education, Science, and Technology (Regional Core Research Program/Biohousing Research Institute). This work was also supported by the Biohousing Research Center and Animal Medical Institute, Korea.
References
1. Dudkin VE, Potapov YuV, Akopova AB, Melkumyan LV, Benton EV, Frank AL. Differential neutron energy spectra measured on spacecraft in low earth orbit. Int J Rad Appl Instrum D. 1990. 17:87–91.


2. Dyer CS, Truscott PR, Evans H, Sims AJ, Hammond N, Comber C. Secondary radiation environments in heavy space vehicles and instruments. Adv Space Res. 1996. 17:53–58.


3. Eguchi-Kasai K, Murakami M, Itsukaichi H, Fukutsu K, Kanai T, Furusawa Y, Sato K, Ohara H, Yatagai F. The role of DNA repair on cell killing by charged particles. Adv Space Res. 1996. 18:109–118.


4. Grahn D. Genetic risks associated with radiation exposures during space flight. Adv Space Res. 1983. 3:161–170.


5. Keith JE, Badhwar GD, Lindstrom DJ. Neutron spectrum and dose-equivalent in shuttle flights during solar maximum. Int J Rad Appl Instrum. 1992. 20:41–47.


6. Kim JS, Jung J, Lee HJ, Kim JC, Wang H, Kim SH, Shin T, Moon C. Differences in immunoreactivities of Ki-67 and doublecortin in the adult hippocampus in three strains of mice. Acta Histochem. 2009. 111:150–156.


7. Kim JS, Lee HJ, Kim JC, Kang SS, Bae CS, Shin T, Jin JK, Kim SH, Wang H, Moon C. Transient impairment of hippocampus-dependent learning and memory in relatively low-dose of acute radiation syndrome is associated with inhibition of hippocampal neurogenesis. J Radiat Res (Tokyo). 2008. 49:517–526.


8. Raber J, Rola R, LeFevour A, Morhardt D, Curley J, Mizumatsu S, VandenBerg SR, Fike JR. Radiation-induced cognitive impairments are associated with changes in indicators of hippocampal neurogenesis. Radiat Res. 2004. 162:39–47.


9. Rola R, Otsuka S, Obenaus A, Nelson GA, Limoli CL, VandenBerg SR, Fike JR. Indicators of hippocampal neurogenesis are altered by 56Fe-particle irradiation in a dose-dependent manner. Radiat Res. 2004. 162:442–446.


10. Seo HS, Yang M, Song MS, Kim JS, Kim SH, Kim JC, Kim H, Shin T, Wang H, Moon C. Toluene inhibits hippocampal neurogenesis in adult mice. Pharmacol Biochem Behav. 2010. 94:588–594.


11. Shukitt-Hale B, Casadesus G, Cantuti-Castelvetri I, Rabin BM, Joseph JA. Cognitive deficits induced by 56Fe radiation exposure. Adv Space Res. 2003. 31:119–126.
12. Shukitt-Hale B, Casadesus G, McEwen JJ, Rabin BM, Joseph JA. Spatial learning and memory deficits induced by exposure to iron-56-particle radiation. Radiat Res. 2000. 154:28–33.


13. Snyder JS, Hong NS, McDonald RJ, Wojtowicz JM. A role for adult neurogenesis in spatial long-term memory. Neuroscience. 2005. 130:843–852.


14. Tada E, Parent JM, Lowenstein DH, Fike JR. X-irradiation causes a prolonged reduction in cell proliferation in the dentate gyrus of adult rats. Neuroscience. 2000. 99:33–41.


15. Yang M, Kim JS, Song MS, Kim JC, Shin T, Lee SS, Kim SH, Moon C. Dose-response and relative biological effectiveness of fast neutrons: induction of apoptosis and inhibition of neurogenesis in the hippocampus of adult mice. Int J Radiat Biol. 2010. 86:476–485.

