Abstract
In this study, we measured the insulin-like growth factor (IGF)-I levels and evaluated the serum protein profiles of diabetic, insulin-treated, and healthy cats and dogs. The total IGF-I concentrations were 33.74 ± 3.4 ng/mL for normal, 25.8 ± 4.5 ng/mL for diabetic, and 180.4 ± 31.4 ng/mL for insulin-treated cats. IGF-I concentrations were 46.4 ± 6.6 ng/mL for normal, 25.1 ± 4.1 ng/mL for diabetic, and 303.0 ± 61.3 ng/mL for insulin-treated dogs. Total serum protein profiles were analyzed by SDS-PAGE. Fourteen bands ranging from 25 to 240 kDa in size were observed for cats, and 17 bands ranging from 25 to 289 kDa were observed for dogs. The densities of the bands differed among control, diabetic, and insulin-treated animals. In conclusion, we found that serum protein profiles and IGF-I concentrations were altered in both diabetic and insulin-treated animals. When judiciously interpreted in the light of other clinical and laboratory data, the techniques used in our study provide a valuable modality for measuring the severity of diabetes mellitus in dogs and cats.
Diabetes mellitus is one of the most common metabolic diseases in cats and dogs. This condition is characterized by increased levels of blood glucose (hyperglycemia) resulting from defects in insulin secretion, insulin action, or both [2,16]. It causes disorders in the metabolism of carbohydrates, lipids, and protein [29]. Diabetes mellitus is classified into four types; in humans, the most common are insulin dependent (type 1) and non-insulin dependent (type 2).
Type 1 diabetes is characterized by insulin secretion deficiencies and is treated with insulin injections in both dogs and humans. In cases of type 2 diabetes, insulin is secreted but glucose metabolism is inhibited due to insulin resistance. In humans and felines, this form of diabetes is characterized by amyloid deposition in Langerhans islands. Among animals, the prevalence of the different forms of diabetes varies with species. While type 1 diabetes is usually seen in dogs, type 2 more frequently develops in cats [30,31,47]. Other types of diabetes account for a smaller ratio of cases in dogs and cats. Insulin was first described by Banting and Best in 1921 [5] as a factor responsible for glucose homeostasis. This hormone facilitates glucose entrance into the cell. Its deficiency leads to increased protein catabolism [41,44] and hyperglycemia. Insulin is an anabolic agent and an important regulator of the growth hormone (GH) and insulin-like growth factors (IGF) [7].
The two major IGF-I and -II were found to be low molecular weight polypeptide hormones [34]. IGF-I is mainly synthesized in the liver, and the secretion of IGF-I was stimulated by growth hormone [38]. This polypeptide shares many structural, functional, and metabolic similarities with insulin and proinsulin [34]. Most circulating IGF-I is bound to specific carrier proteins; only free IGF-I is biologically active. Six IGF-binding proteins (IGFBP) have been identified (IGFBP-1 to IGFBP-6), four of which are important in human serum (IGFBP-1 to IGFBP-4). IGFBP-3 is the major binding protein for IGF-I that forms a 140 kDa complex in humans, and plays a role in glucose metabolism [23]. The active form of IGF-I binds to the IGF-I receptor, which is highly homologous to the insulin receptor, and activates a cascade of intracellular events that result in the stimulation of glucose utilization [19,27].
The effects of IGF-I have been investigated in several experimental animal studies [10,37]. IGF-I has been reported to have effects similar to those of insulin such as increasing glucose uptake, stimulation of glucose transport, glucose oxidation, and glycogen formation. IGF-I is also known to have anabolic effects on protein metabolism, including inhibition of whole-body protein breakdown and stimulation of protein synthesis [17]. In humans with untreated cases of type 1 or 2 diabetes, the levels of IGF-I have been reported to decrease [3,19]. IGFBP-3 concentrations have also been found to decrease in hyperglycemic humans [6] and rats [13,18]. The aim of the present study was to compare IGF-I levels between healthy, insulin-treated diabetic, and hyperglycemic cats and dogs. In addition, we evaluated the serum protein profiles of these animals.
Seven cats (6 striped and 1 Turkish angora) with diabetes mellitus and seven cats (4 striped, 2 Turkish angora and 1 Scottish fold) with insulin-treated were used for study. Twenty dogs (13 mongrel, 5 wolfhound, 1 Huskey and 1 Turkish kangal) with diabetes mellitus and seven dogs (4 mongrel and 3 wolfhound) with insulin-treated mellitus were also included in our study. The animals used in the study were selected from the animals which were come to the polyclinics for routine health controls or diabetes treatment. Finally, seven healthy cats (5 striped, 1 Turkish angora and 1 Turkish van) and seven healthy dogs (3 mongrel, 2 wolfhound, and 2 Turkish kangal) served as control groups. The age of all animals ranged from 4 to 16 years (median, 10 years) and average body weights ranged from 2.5 to 9.8 kg (median, 5.5 kg). The diagnosis of diabetes mellitus was determined based on clinical signs such as polyuria, polydipsia, and weight loss, and laboratory findings indicating the presence of hyperglycemia and glucosuria.
The neutral protamine Hagedorn form of insulin (Humulin-N; Eli Lilly, USA) was subcutaneously injected at a concentration of 0.4~0.7 IU/kg for dogs and 0.25~0.5 IU/kg for cats with insulin-treated diabetes. Blood samples were collected 1.5 h after insulin injection from cats and dogs fasted for approximately 8 h. The samples were kept at room temperature for 15 min to allow clotting and were then centrifuged (4℃, 1,550 × g, 10 min). The sera recovered from the samples were stored at -80℃ until use. The levels of glucose, total protein, albumin, and globulin were determined using a bench-top immunoassay auto-analyzer (Autolab; AMS Srl, The Netherlands).
The concentration of IGF-I in the serum samples was analyzed by using a sandwich enzyme-linked immunoassay (ELISA) kit (E90050Ca; Uscn Life Science, China). The ELISA was performed according to manufacturer's instructions as follows; the wells of precoated kits were determined for diluted standards, blank and samples. Each of dilutions of standards, blank and samples were added about 100 µL to the wells and incubated for 2 h at 37℃. After washing step, 100 µL of Detection Reagent A working solution was added to each well and incubated for 1 h at 37℃. The wells were washed and 100 µL of Detection Reagent B working solution was added to each well. After incubation for 30 min at 37℃, the washing process was repeated. TMB Substrate Solution (90 µL to each well) was added and incubated for 15~25 min at 37℃. Then, 50 µL of Stop Solution was added to each well and measurement was conducted at 450 nm.
SDS-PAGE was carried out on 10% polyacrylamide gels under denaturing conditions [20]. Serum samples were diluted (v/v; 1/50) with a sterile physiologic saline solution, mixed with 15.5 mmol/L Tris-HCl, 3% SDS, 10% glycerol and 0.02% bromophenol blue (pH 6.8); and heated at 100℃ for 5 min followed by gentle cooling. Samples (20 µL) were loaded onto the stacking gel and electrophoresis was performed at a constant voltage (200 V). Following electrophoresis, the gel was stained with Blue Silver [12]. Molecular mass standards (S8445; Sigma, USA) were run in parallel in order to calculate protein molecular weights using Molecular Imaging Software (Kodak, USA). The SDS-PAGE results were evaluated for variation of band densities as well as similarities in the number and patterns of the bands. Dendograms (UPGMA) were created based on the Dice similarity coefficient including the genetic relatedness between groups (cats and dogs; control and diabetic cats; control and diabetic dogs; insulin-treated cats and dogs) using CHEF-DR III and Quantity One Software (Bio-Rad Laboratories, USA).
Normal distribution of the data was determined with the Shapiro-Wilk test and while general distribution parameters of data were evaluated with descriptive statistics. To investigate statistical differences among the IGF-I, albumin, globulin, and total protein concentrations between the groups in this study, we initially performed Duncan's multiple range test. Pearson Correlations Analysis was also used to evaluate the relationships among the traits we examined in the present study. Statistical analyses were performed with SAS, statistical package release 8.1 (SAS, USA).
The concentrations of IGF-I were 25.8 ± 4.50 g/mL and 25.1 ± 4.10 ng/mL in diabetic cats and dogs, respectively. These levels were significantly lower than those of the control groups (33.7 ± 3.40 ng/mL and 46.4 ± 6.60 ng/mL for control cats and dogs, respectively). The IGF-I concentrations for insulin-treated cats and dogs were 180.4 ± 31.40 ng/mL and 303.0 ± 61.30 ng/mL, respectively. These results showed that the concentration of IGF-I in sera of diabetic cats and dogs were significantly lower than those of both healthy and insulin-treated animals (p < 0.05). IGF-I concentrations increased significantly with insulin treatment in diabetic animals. The importance control of differences among the groups' means with Duncan's multiple range test are shown in Tables 1 and 2.
There was a negative correlation between the serum albumin and globulin concentration in cats (r = -0.751) and dogs (r = -0.534). A positive correlation (r = 0.656) between serum glucose and globulin concentrations was found in cats. A positive correlation was also observed between total protein and globulin (r = 0.687) levels. The correlation between some serum parameters of cats and dogs are presented in Tables 3 and 4, respectively.
In the SDS-PAGE analysis of total serum proteins of all cats and dogs, 14 bands between 25 to 240 kDa in size and 17 bands between 25 to 289 kDa were observed. The density of the bands which were approximately 29, 34, 42, 44, and 240 kDa in size were different between control, diabetic, and insulin-treated animals (Fig. 1). The similarities of the sizes of protein bands were evaluated with software as described above. Serum profiles were found to have a similarity of 72% between cats and dogs, 75% between control and diabetic cats, 79% between control and diabetic dogs, 83% between diabetic and insulin-treated cats, and 93% between diabetic and insulin-treated dogs.
Diabetes is a very complex disease and differences in some serum parameters between diabetic cats and dogs are still unclear. IGF-I is a hormone which resembles insulin in terms of molecular structure and biochemical properties. Recent study has suggested that IGF-I plays a role in regulating glucose metabolism [39]. The results of the present study confirmed that diabetes in cats and dogs adversely affected serum IGF-I concentration. We measured serum concentrations of IGF-I in diabetic cats and dogs that had been previously treated with insulin as well as untreated animals. The serum IGF-I concentrations in untreated diabetic cats and dogs were significantly lower than those of the control cats and dogs. However, the serum IGF-I concentrations in the insulin-treated diabetic cats and dogs were higher than those in healthy as well as untreated diabetic animals. Several studies [7,15,33,38,42] have also measured serum IGF-I concentrations in diabetic cats and dogs; these concentrations were reduced in these animals. Similarly, IGF-I concentrations were also reported to be decreased in type 1 and type 2 diabetic humans [4,11,14] and rats [1,38].
Insulin and sex steroids have stimulatory effects on the synthesis and secretion of IGF-I [9]. The major source of circulating IGF-1 is produced by the liver and is dependent on appropriate insulin levels [41]. Low levels of insulin secretion over a long time result in reduced IGF-I secretion. Although the structure, function, and regulation of IGF-I in humans, rodents, and non-human primates have been reported, few studies about IGF-I in diabetic cats and dogs exist in the literature [43]. It has been found that the reduction of circulating IGF-I causes decreases in insulin levels [40], increased protein synthesis, and inhibited protein catabolism [36]. The levels of IGF-I have been reported to be high in diabetic and acromegalic cats and dogs [8,15]. However, no changes was found in diabetes mellitus for IGF-I concentrations [18]. Albumin constitutes a large proportion of all plasma protein [46]. In hyperglycemia, serum albumin levels have been reported to decrease [26] while the urinary excretion of albumin has been reported to increase with diabetes [45]. In the present study, the total protein, globulin, and albumin concentrations of all dogs were not significantly different. The levels of albumin in diabetic cats were lower than those of the insulin-treated diabetic and control cats. However, an increase in serum globulin concentrations was observed in diabetic cats. Globulins constitute a much smaller fraction of the total serum protein content. The increased levels of globulin in our study might have been due to increased β-1 or β-2 globulins reported to occur with diabetes mellitus [28]. We also noted a statistically significant increase in the serum glucose concentration of diabetic cats and dogs (p < 0.001).
Serum protein profiling provides a valuable tool for diagnosing many diseases [21,29]. In the serum SDS-PAGE analysis performed in this study, we identified 14 bands ranging from 25 to 240 kDa in size in cats, and 17 bands between 25 in size 289 kDa in dogs. The intensities of the 25, 29, 34, 32, 42, 44, and 240 kDa bands were different when comparing control, diabetic, and insulin-treated dogs and cats. Most circulating IGF-I is bound to specific proteins; only free IGF-I is biologically active. So far, six different IGFBP isoforms with molecular weights ranging between 25~150 kDa have been found [35]. Four of these are important in human serum (IGFBP-1 to 4) in which IGF-I is predominantly bound to IGFBP-3 [32]. On the other hand, a previous study [25] reported that IGFBP-3 appears as doublet (39 and 43 kDa) in cats. Lewitt et al. [22] has also reported that cat serum contains IGFBP-3 doublets bands with molecular masses of approximately 45 kDa and 25~35 kDa. We observed doublet bands with molecular masses of 42~44 kDa and 32~34 kDa in cats and dogs, respectively. The intensities of the 32, 34, 42, and 44 kDa bands in diabetic cats were found to be lower than those of insulin-treated and control cats. On the other hand, the intensities of 32, 34, 42, and 44 kDa bands in diabetic dogs were higher than those of insulin-treated dogs.
A 29 kDa band has been previously identified as IGFBP-1 in cats by immunoblotting [25]. In our study, the density of 29 kDa protein band was found as decreased in insulin-treated cats whereas the density of 25 kDa protein band increased in diabetic compared to insulin-treated cats. However, an increase in the densities of the 29 and 25 kDa protein bands was observed in insulin-treated dogs. In diabetic cats and dogs, the density of 240 kDa band decreased; however, the density of this band increased after insulin treatment. In summary, the densities of the 240, 42, 44, 32, 34, and 25 kDa bands increased but the 29 kDa band decreased in insulin-treated cats. In insulin-treated dogs, the 240, 49, 29, and 25 kDa band densities increased but the density of the 42 and 44 kDa bands decreased. These results indicate that the protein factors corresponding to the 240, 44, 42, 25, and 29 kDa bands may have important effects on glucose homoeostasis in both cats and dogs.
SDS-PAGE band patterns have been increasingly used to determine the relationships or similarities between two groups (cats and dogs; control and diabetic cats; control and diabetic dogs; insulin-treated cats and dogs). Computerized comparison of electrophoretic protein patterns is a fast, easy, and powerful analytical method. SDS-PAGE patterns can be quickly obtained, are reproducible, and do not require any relatively sophisticated and expensive reagents or equipment [24]. No comparison of serum protein profiles among cats and dogs (diabetic, insulin-treated, and control) has been reported in the literature. Our SDS-PAGE analysis demonstrated that the serum profiles between the different groups of animals were generally similar (72 to 93%).
In conclusion, the findings obtained in this study suggested that variations of serum protein profiles and IGF-I concentrations in cats and dogs with diabetic or insulin-treated may be associated with glucose metabolism. Serum protein profiles, when supported by other clinical and laboratory data, are a valuable additional way to measure the severity of diabetes. However, further studies are required for fully understanding the mechanisms underlying this disease.
Figures and Tables
Fig. 1
(A) SDS-PAGE analysis and (B) dendogram analysis (phylophenotic tree created according to the SDS-PAGE profiles) of the representative serum from control, insulin-treated, diabetic dogs and cats. Lane 1: control cats, Lane 2: diabetic cats, Lane 3: insulin-treated cats, Lane 4: control dogs, Lane 5: diabetic dogs, Lane 6: insulin-treated dogs, Lane M: marker.
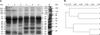
References
1. Asada T, Takakura S, Ogawa T, Iwai M, Kobayashi M. Overexpression of glucose transporter protein 5 in sciatic nerve of streptozotocin-induced diabetic rats. Neurosci Lett. 1998. 252:111–114.


2. Aytuğ N. İmren HY, editor. Metabolizma hastalıkları. Kedi ve Köpek Hastalıkları. 1998. Ankara: Medisan Yayınları;345–346.
3. Bach LA, Rechler MM. Insulin-like growth factors and diabetes. Diabetes Metab Rev. 1992. 8:229–257.


4. Bach MA, Chin E, Bondy CA. The effects of subcutaneous insulin-like growth factor-I infusion in insulin-dependent diabetes mellitus. J Clin Endocrinol Metab. 1994. 79:1040–1045.


5. Banting FG, Best CH. Pancreatic extracts. 1922. J Lab Clin Med. 1990. 115:254–272.
6. Baxter RC. Characterization of the acid-labile subunit of the growth hormone-dependent insulin-like growth factor binding protein complex. J Clin Endocrinol Metab. 1988. 67:265–272.


7. Bereket A, Lang CH, Wilson TA. Alterations in the growth hormone-insulin-like growth factor axis in insulin dependent diabetes mellitus. Horm Metab Res. 1999. 31:172–181.


8. Berg RIM, Nelson RW, Feldman EC, Kass PH, Pollard R, Refsal KR. Serum insulin-like growth factor-I concentration in cats with diabetes mellitus and acromegaly. J Vet Intern Med. 2007. 21:892–898.


9. Blum WF. Ranke MB, editor. Insulin-like growth factors and their binding proteins. Diagnostics of Endocrine Function in Children and Adolescents. 1996. Heidelberg: Barth Verlag;190–218.


10. Boulware SD, Tamborlane WV, Rennert NJ, Gesundheit N, Sherwin RS. Comparison of the metabolic effects of recombinant human insulin-like growth factor-I and insulin. Dose-response relationships in healthy young and middle-aged adults. J Clin Invest. 1994. 93:1131–1139.


11. Brismar K, Fernqvist-Forbes E, Wahren J, Hall K. Effect of insulin on the hepatic production of insulin-like growth factor-binding protein-1 (IGFBP-1), IGFBP-3, and IGF-I in insulin-dependent diabetes. J Clin Endocrinol Metab. 1994. 79:872–878.


12. Candiano G, Bruschi M, Musante L, Santucci L, Ghiggeri GM, Carnemolla B, Orecchia P, Zardi L, Righetti PG. Blue silver: a very sensitive colloidal Coomassie G-250 staining for proteome analysis. Electrophoresis. 2004. 25:1327–1333.


13. Dai J, Baxter RC. Regulation in vivo of the acid-labile subunit of the rat serum insulin-like growth factor-binding protein complex. Endocrinology. 1994. 135:2335–2341.


14. Dunger DB, Acerini CL. IGF-I and diabetes in adolescence. Diabetes Metab. 1998. 24:101–107.
15. Eigenmann JE, Becker M, Kammermann B, Leemann W, Heimann R, Zapf J, Froesch ER. Decrease of non-suppressible insulin-like activity after pancreatectomy and normalization by insulin therapy. Acta Endocrinol (Copenh). 1977. 85:818–822.


16. Expert Committee on the Diagnosis and Classification of Diabetes Mellitus. Report of the expert committee on the diagnosis and classification of diabetes mellitus. Diabetes Care. 2003. 26:Suppl 1. S5–S20.
17. Fryburg DA. Insulin-like growth factor I exerts growth hormone- and insulin-like actions on human muscle protein metabolism. Am J Physiol. 1994. 267(2 Pt 1):E331–E336.


18. Frystyk J, Baxter RC. Competitive binding assay for determination of rat insulin-like growth factor binding protein-3. Endocrinology. 1998. 139:1454–1457.


19. Kratzsch J, Keliner K, Zilkens T, Schmidt-Gayk H, Selisko T, Scholz GH. Growth hormone-binding protein related immunoreactivity is regulated by the degree of insulinopenia in diabetes mellitus. Clin Endocrinol (Oxf). 1996. 44:673–678.


20. Laemmli UK. Cleavage of structural proteins during the assembly of the head of bacteriophage T4. Nature. 1970. 227:680–685.


21. Laronga C, Becker S, Watson P, Gregory B, Cazares L, Lynch H, Perry RR, Wright GL Jr, Drake RR, Semmes OJ. SELDI-TOF serum profiling for prognostic and diagnostic classification of breast cancers. Dis Markers. 2003-2004. 19:229–238.


22. Lewitt MS, Hazel SJ, Church DB, Watson ADJ, Powell SE, Tan K. Regulation of insulin-like growth factor-binding protein-3 ternary complex in feline diabetes mellitus. J Endocrinol. 2000. 166:21–27.


23. Löfqvist C, Andersson E, Gelander L, Rosberg S, Hulthen L, Blum WF, Wikland KA. Reference values for insulin-like growth factor-binding protein-3 (IGFBP-3) and the ratio of insulin-like growth factor-I to IGFBP-3 throughout childhood and adolescence. J Clin Endocrinol Metab. 2005. 90:1420–1427.


24. Massa R, Bantar C, Lopardo H, Vay C, Gutkind G. Whole-cell protein profiles are useful for distinguishing enterococcal species recovered from clinical specimens. Rev Argent Microbiol. 2007. 39:199–203.
25. Maxwell A, Butterwick R, Batt RM, Camacho-Hübner C. Serum insulin-like growth factor (IGF)-I concentrations are reduced by short-term dietary restriction and restored by refeeding in domestic cats (Felis catus). J Nutr. 1999. 129:1879–1884.


26. McMillan DE. Increased levels of acute-phase serum proteins in diabetes. Metabolism. 1989. 38:1042–1046.


27. Myers MG Jr, Sun XJ, Cheatham B, Jachna BR, Glasheen EM, Backer JM, White MF. IRS-1 is a common element in insulin and insulin-like growth factor-I signaling to the phosphatidylinositol 3'-kinase. Endocrinology. 1993. 132:1421–1430.


28. O'Connell TX, Horita TJ, Kasravi B. Understanding and interpreting serum protein electrophoresis. Am Fam Physician. 2005. 71:105–112.
29. Okumuş Z. Diabetes mellitus in dogs and cats Part 1: Etyology, pathogenesis and treatment. Kafkas Univ Vet Fak Derg. 1999. 5:223–231.
30. Rand JS, Fleeman LM, Farrow HA, Appleton DJ, Lederer R. Canine and feline diabetes mellitus: nature or nurture? J Nutr. 2004. 134:8 Suppl. 2072S–2080S.


31. Rand JS, Marshall RD. Diabetes Mellitus in Cats. Vet Clin North Am Small Anim Pract. 2005. 35:211–224.


32. Raynaud-Simon A, Perin L, Meaume S, Lesourd B, Moulias R, Postel-Vinay MC, Le Bouc Y. IGF-I, IGF-I-binding proteins and GH-binding protein in malnourished elderly patients with inflammation receiving refeeding therapy. Eur J Endocrinol. 2002. 146:657–665.


33. Reusch CE, Kley S, Casella M, Nelson RW, Mol J, Zapf J. Measurements of growth hormone and insulin-like growth factor 1 in cats with diabetes mellitus. Vet Rec. 2006. 158:195–200.


34. Rinderknecht E, Humbel RE. The amino acid sequence of human insulin-like growth factor I and its structural homology with proinsulin. J Biol Chem. 1978. 253:2769–2776.


35. Rosenfeld RG, Lamson G, Pham H, Oh Y, Conover C, De Leon DD, Donovan SM, Ocrant I, Giudice L. Insulinlike growth factor-binding proteins. Recent Prog Horm Res. 1990. 46:99–159.


36. Russell-Jones DL, Umpleby AM, Hennessy TR, Bowes SB, Shojaee-Moradie F, Hopkins KD, Jackson NC, Kelly JM, Jones RH, Sönksen PH. Use of a leucine clamp to demonstrate that IGF-I actively stimulates protein synthesis in normal humans. Am J Physiol. 1994. 267(4 Pt 1):E591–E598.


37. Russell-Jones DL, Bates AT, Umpleby AM, Hennessy TR, Bowes SB, Hopkins KD, Jackson N, Kelly J, Shojaee-Moradie F, Jones RH, Sonksen PH. A comparison of the effects of IGF-I and insulin on glucose metabolism, fat metabolism and the cardiovascular system in normal human volunteers. Eur J Clin Invest. 1995. 25:403–411.


38. Scheiwiller E, Guler HP, Merryweather J, Scandella C, Maerki W, Zapf J, Froesch ER. Growth restoration of insulin-deficient diabetic rats by recombinant human insulin-like growth factor I. Nature. 1986. 323:169–171.


39. Simpson HL, Jackson NC, Shojaee-Moradie F, Jones RH, Russell-Jones DL, Sönksen PH, Dunger DB, Umpleby AM. Insulin-like growth factor I has a direct effect on glucose and protein metabolism, but no effect on lipid metabolism in type 1 diabetes. J Clin Endocrinol Metab. 2004. 89:425–432.


40. Sjögren K, Wallenius K, Liu JL, Bohlooly-Y M, Pacini G, Svensson L, Törnell J, Isaksson OG, Ahrén B, Jansson JO, Ohlsson C. Liver-derived IGF-I is of importance for normal carbohydrate and lipid metabolism. Diabetes. 2001. 50:1539–1545.


41. Sönksen PH, Russell-Jones D, Jones RH. Growth hormone and diabetes mellitus. A review of sixty-three years of medical research and a glimpse into the future? Horm Res. 1993. 40:68–79.
42. Starkey SR, Tan K, Church DB. Investigation of serum IGF-I levels amongst diabetic and non-diabetic cats. J Feline Med Surg. 2004. 6:149–155.


43. Struble AL, Nelson RW. Non insulin-dependent diabetes mellitus in cats and humans. Compend Contin Educ Pract Vet. 1997. 19:935–944.
44. Stogdale L. Definition of diabetes mellitus. Cornell Vet. 1986. 76:156–174.