Abstract
Effect of sodium nitroprusside (SNP), a nitric oxide (NO) donor, on in vitro survival, growth, steroidogenesis, and apoptosis of buffalo preantral follicles (PFs) was investigated. PFs (200~250 µm) were isolated by micro-dissection and cultured in 0 (control), 10-3, 10-5, 10-7, and 10-9 M SNP. To examine the reversible effect of SNP, PFs were cultured with 10-5 M SNP + 1 mM Nω-nitro-L-arginine methyl ester (L-NAME) or 1.0 µg hemoglobin (Hb). The results showed that greater concentrations of SNP (10-3, 10-5, 10-7 M) inhibited (p < 0.05) FSH-induced survival, growth, antrum formation, estradiol production, and oocyte apoptosis in a dose-dependent manner. However, a lower dose of SNP (10-9 M) significantly stimulated (p < 0.05) the survival, growth, antrum formation, follicular oocyte maturation, and stimulated progesterone secretion compared to the control. A combination of SNP + L-NAME promoted the inhibitor effect of SNP while a SNP + Hb combination reversed this effect. Nitrate and nitrite concentrations in the culture medium increased (p < 0.05) in a dose-dependent manner according to SNP concentration in the culture medium. At higher concentrations, SNP had a cytotoxic effect leading to follicular oocyte apoptosis whereas lower concentrations have stimulatory effects. In conclusion, NO exerts a dual effect on its development of buffalo PFs depending on the concentration in the culture medium.
Nitric oxide (NO) is a ubiquitous free radical molecule that plays a significant role in different physiological systems. It is synthesized from L-arginine by NO synthase (NOS), an enzyme that exists in three isoforms [24]. Two of these, neuronal NOS (nNOS) and endothelial NOS (eNOS), are synthesized at a constant rate and seem to be responsible for the continuous basal release of NO regardless of physiological demand. The third isoform, inducible NOS (iNOS), and is expressed in response to inflammatory cytokines and lipopolysaccharides [25]. In mammals, several studies have demonstrated the presence of these isoforms in the ovary by immunohistochemistry and Western blotting. NO is involved in several aspects of female reproduction including ovarian follicular development [14], ovulation [26], steroidogenesis [15], and oocyte meiotic maturation [31]. The enzymes responsible for NO production have been detected in mouse [9], rat [16], pig [38], and cattle [27,28]. Taken together, these reports support the hypothesis that NO plays an important role during follicle and oocyte maturation.
NO synthesis is important for oocyte maturation because eNOS-knockout mice have a reduced number of oocytes in metaphase II along with a high percentage of oocytes that are in metaphase I [14]. Furthermore, sodium nitroprusside (SNP) has been demonstrated to stimulate meiotic maturation in mice [32]. Studies using Nω-nitro-L-arginine methyl ester (L-NAME) to inhibit endogenous and exogenous (via SNP supplementation) NO production found that NO is essential for optimal meiotic maturation both in vitro and in vivo [16]. It has been reported that early follicles produce more NO than those in later stages of development [13], showing that NOS activity changes during follicular development. Bilodeau-Goeseels [2] reported that excess NO inhibits germinal vesicle breakdown (GVBD) whereas NO reduction during the initial hours of in vitro maturation stimulates GVBD. Therefore, the NOS/NO system may have important roles during different developmental phases of mammalian ovarian follicles but the role of this system in buffalo follicle development is not clear.
Antrum formation is one of the important events of mammalian follicle development. It has been found that FSH and IGF-I promotes antrum formation in pig [21] and buffalo [33] preantral follicles (PFs). Studies have shown that NO is a negative regulator of steroid synthesis and exerts its effects by binding to the prosthetic heme group of P450 aromatase enzyme [12,39]. NO/cyclic guanosine monophosphate pathway has been suggested as one of the mechanisms used by NO to inhibit granulosa cells steroidogenesis in pig [10]. NO also acts as a pro- or anti-apoptotic agent depending on the amount produced and cell type [19]. Follicular atresia is associated with apoptosis and the level of NO [13]. Chun et al. [6] reported that NO suppresses follicular apoptosis, suggesting that NO acts as a follicle survival factor.
Previous studies in the literature have not elucidated the role of NO in regulating the growth, survival, apoptosis, and antrum formation of buffalo follicles. Involvement of NO during preantral follicle development may be partly responsible for low reproductive efficiency, low primordial/preantral follicle populations, and high incidence of follicular atresia in economically important livestock buffalo species, especially in India. Therefore, present study was designed to investigate whether SNP induces or inhibits the survival, growth, steroidogenesis, and apoptosis of buffalo PFs.
All chemicals used in this study were purchased from Sigma-Aldrich (USA) unless otherwise indicated.
Ovaries in different stages of the estrous cycle were randomly collected from a abattoir located at Mohanpur-theria, Bareilly, India and transported at 25~30℃ in 0.9% saline to the reproductive physiology laboratory of Indian Veterinary Research Institute, Bareilly within 2 h. The ovaries were rinsed in pre-warmed phosphate buffer saline (PBS) supplemented with antibiotics (75 mg/L penicillin-G and 50 mg/L streptomycin sulphate). In a laminar flow hood, fine cortical sections (approximately 0.5~1.0 mm thick) were cut from the ovarian surface using a surgical blade and placed in tissue culture medium-199 (TCM-199) supplemented with sodium pyruvate (2 mM), glutamine (2 mM), bovine serum albumin (BSA, 3 mg/mL) and antibiotics. PFs (200~250 µM) were isolated using a microdissection method described by Sharma et al. [34]. The isolated follicles were washed twice in TCM-199 containing 10% fetal bovine serum (FBS). Healthy PFs (compact granulosa cell layers and a visible centrally-located oocyte) were selected for culturing.
The isolated PFs were maintained in HEPES-buffered (5 mM) TCM-199 supplemented with sodium bicarbonate (26 mM). To determine the optimal effective dose of NO, the isolated PFs were divided into five treatment groups. The control medium was supplemented with 10% FBS, 1% insulin, transferrin, and selenium solution (ITS), and 0.5 µg/mL follicle stimulating hormone (FSH). The addition of SNP at 10-3, 10-5, 10-7, and 10-9 M to the control medium were the different treatment media. To determine whether the growth inhibitory effect of SNP were reversible or not, PFs (4~5 PFs/droplet of culture medium) were cultured with 10-5 M SNP and 1.0 mM L-NAME, an NOS inhibitor, or 1.0 µg of hemoglobin (Hb), an NO scavenger. Control PFs were cultured without SNP. The highest concentration of SNP (10-3 M) was excluded from this experiment because it almost abolished PF growth and survival; 10-7 SNP had an inhibitory effect and 10-9 SNP had a stimulatory effect. PFs selected for in vitro culturing were placed in 50 µL (4~5 PFs/droplet of culture medium) of the respective culture medium in 35 mm plastic tissue culture dishes (Nunc, Denmark) and overlaid with 2 mL of embryo-tested lightweight mineral oil. The culture dishes were placed in a humidified incubation chamber (Thermo Forma, USA) and incubated at 38 ± 1℃ in a maximum humidified atmosphere of 5% CO2 in air. A two-third volume of the medium was replenished every third day with an equal volume of fresh medium.
In vitro cultured PFs from all the treatment groups were evaluated according to two factors: folliculogenesis (follicle survival, growth, and antrum formation) and oogenesis (follicular oocyte maturation and apoptosis). The morphology of each follicle was evaluated every other day using a phase contrast inverted microscope (CKX 41; Olympus, Japan). The follicle diameter was measured in duplicate (basement membrane to basement membrane) using Image J 1.33U software (National Institutes of Health, USA) based on a calibrated ocular micrometer. The number of PFs exhibiting antrum formation and extruded oocytes was determined. Eccentric displacement of the oocyte within the follicles was considered to be an indicator of in vitro follicular development. PFs were stained with 0.5% (w/v) Trypan blue (Sigma-Aldrich, USA) for 5~10 min at the end of culture period to evaluate their viability. Degenerated PFs having irregular basement membrane and loose follicular cells were discarded.
To evaluate DNA fragmentation in spontaneously extruded or mechanically isolated oocytes from the different treatment groups, a terminal deoxynucleotidyl transferase-mediated dUTP biotin nick end labeling (TUNEL) in situ detection kit (R&D Systems, USA) was used. The TUNEL assay was performed as previously described by Chaube et al. [5]. To prevent the loss of oocytes during assay, poly-L-lysin-coated glass slides were used. The control and different treatment groups (4 to 6 oocytes per group) were immediately transferred to the slide and fixed with 3.7% formaldehyde in PBS for 15 min at 18~20℃. The slides were washed twice with PBS and air dried at room temperature; all the procedures were carried out at 18~20℃ unless stated otherwise. The apoptotic signal was recorded as positive when the nucleus contained either diffuse or granular dark brown staining. Oocytes with positive TUNEL staining showing more than 50% of the dark brown staining of nucleoplasm were considered to be apoptotic.
To determine the meiotic stages of the oocytes, spontaneously extruded or mechanically isolated oocytes from different treatment groups of cultured PFs were examined. The cumulus cells were removed by treatment with 1.0% pronase and then placed onto L-poly-lysine-coated glass slides. To fix the oocyte, the slides were immersed in a methanol : acetic acid solution (3 : 1) for at least 24 h prior to staining, the fixative solution was removed by washing two to three times with 100% methanol. The oocytes were stained with a 1% (w/v) aceto-orcein solution and examined under a light microscope. The oocytes were classified as germinal vesicle stage (GV, immature oocytes), metaphase I (MI), or metaphase II (MII, mature oocytes) as described by Sharma et al. [35].
To assess the ability of in vitro cultured PFs to secrete steroids in the presence or absence of SNPs, estradiol and progesterone were measured in the culture medium collected on culture days 3, 6, and 9 using commercially-available radio-immunoassay kits (Immunotech, Czech Republic). To measure steroid concentrations, medium from each treatment groups was assayed in duplicate. The assays were carried out according to the manufacturer's protocol. The analytical sensitivity of the progesterone and estradiol kits was 0.02 ng and 4.5 pg, respectively. Inter- and intra-assay variation coefficients were 8.5% and 4.5% for progesterone, and 11.2% and 12.1% for estradiol, respectively.
The concentrations of NO metabolites (nitrate and nitrite) were measured in the PF culture medium as described by Sastry et al. [30]. Briefly, 100 µL of the sample or standard (potassium nitrate, 100 µm/L) was mixed with 400 µL of 0.55 M carbonate buffer (pH 9.0). Approximately 100 mg of activated copper-cadmium alloy filings were added to the samples and the mixture was incubated at 30℃ for 1 h with gentle shaking. Next, 100 µL of 0.35 M sodium hydroxide and 400 µL of 0.12 M zinc sulfate were added and the solution was incubated for 10 min at room temperature. The tubes were centrifuged at 1,600 × g for 10 min and the supernatant (150 µL) was transferred to the wells of microtiter plates in duplicate. Next, 75 µL of 1% (w/v) sulfanilamide and 75 µL of 0.1% (w/v) N-naphthalenediamine were gently added and the plates were incubated for 10 min. Absorbance was measured at 545 nm in a microplate reader (Molecular Device, USA).
Data on the survival of PFs and follicle diameter were collected from 11 replicate samples and expressed as the percentage between the value at the beginning and at the end of the culturing period. Statistical significance was determined using SPSS software for Windows (version 7.5; SPSS, USA) by an ANOVA followed by Duncan's post-hoc multiple comparison test for proportion. The data are presented as the mean ± SE. A p value < 0.05 was considered to be statistically significant. Progesterone and estradiol levels were subjected to statistical analysis as the total hormone accumulation over the culture period for 3, 6, and 9 days.
The addition of higher doses of SNP (10-7, 10-5, and 10-3 M) to the control medium significantly (p < 0.05) inhibited the survival, growth, and antrum formation rates of PFs in a dose-dependent manner compared to the control. However, the lower dose of SNP (10-9 M) stimulated (p < 0.05) the survival, growth, and antrum formation rates of PFs compared to the control and other treatment groups. In the 10-3 M SNP-treated group, only 13% of the PFs survived with a 123% increase in follicular diameter on day 12 of culturing (Tables 1 and 2). Supplementation with 10-7, 10-5, and 10-3 M SNP in the culture medium significantly (p < 0.05) inhibited the transition from MI to MII, and increased the number of TUNEL-positive oocytes in a dose-dependent manner (Table 3). However, all extruded oocytes from PFs in the control and 10-9 M SNP groups reached metaphase II stage (MII, 100%) and showed no sign of apoptosis (Table 3). Co-administration of L-NAME (1.0 mM) with SNP (10-5 M) could not overcome the inhibitory effect of SNP. However, L-NAME reduced the inhibitory effect of SNP on PF survival and growth. Similarly, co-administration of Hb (1.0 µg/mL) with SNP (10-5 M) markedly reduced the SNP-induced inhibitory effect, resulting in an increased PF survival rate up to 53% and growth rate up to 179% similar to the control group on day 12 of culturing (Tables 1 and 2). Administration of Hb also induced the transition of oocytes from MI into MII (83.44%) and reduced SNP (10-5 M)-induced oocyte apoptosis (25%) compared to L-NAME (Table 3).
PFs cultured with higher doses of SNP (10-7, 10-5, and 10-3 M) showed significant inhibition (p < 0.05) of FSH-induced estradiol and progesterone secretion compared to the control group and PFs exposed to 10-9 M SNP on days 3, 6, and 9 of culturing. However, a combination of SNP (10-5 M) with Hb (1.0 µg/mL) reversed these inhibitory effects and induced estradiol and progesterone synthesis similar to those associated with L-NAME treatment (Figs. 1 and 2). Accumulation of NO metabolites (NO-3/NO-2) in culture medium increased significantly (p < 0.05) in a SNP dose-dependent manner. However, L-NAME supplementation with SNP 10-5 M or Hb decreased the level of NO-3/NO-2 production in culture medium (Fig. 3). Overall follicular viability, growth, and antrum formation rates were stimulated by 10-9 M SNP (Figs. 4 and 5) while higher doses of SNP (10-3 and 10-5 M) induced follicular oocyte apoptosis (Fig. 6).
The present study was conducted to assess the consequences of increased NO bioavailability during in vitro PF development, and to determine the influence of this cellular messenger molecule on survival, growth, steroidogenesis and apoptosis. NO has emerged as a potential regulator of follicular development and is implicated in several ovarian processes, including steroidogenesis and ovulation [37]. Several other studies in mammals have provided evidence that NO affects follicular development and apoptosis, and participates in the regulation of corpus luteum function [17,23]. However, the physiological functions of NO/NOS during PF development in buffalo have not been clarified. To our knowledge, the present study provides the first demonstration that NO exerts a dual effect (inhibiting or stimulating) in a dose-dependent manner on buffalo follicular oocyte maturation. On one hand, high concentrations of SNP (10-3 and 10-5 M) inhibited in vitro PF survival, growth, and steroidogenesis, and induced follicular oocytes apoptosis. On the other hand, an intermediate concentration of SNP (10-7 M) had a mild inhibitory effect while a low concentration of SNP (10-9 M) had a stimulatory effect on the survival, growth, and steroidogenesis of PFs compare to the other treatment groups and control. These results indicate that NO has opposing actions on follicular development and oocyte maturation in vitro depending in a concentration-dependent manner.
The use of SNP as an NO donor slows the transition from MI to MII in a dose-dependent manner by inhibiting first polar body extrusion in rodents [4], pigs [38], and bovine [40]. On the other hand, low NO concentrations stimulate meiosis, suggesting that there is a specific physiological concentration of NO that can promote the follicle maturation process [2,32]. In this study, the higher doses of SNP (10-3 and 10-5 M) inhibited the transition from MI to MII while a lower dose of SNP (10-9 M) stimulated this transition and enhanced the growth, survival, and steroidogenesis of buffalo PFs. These result suggested that excess NO could have cytotoxic effects and may react with other free radicals to generate peroxynitrates (ONOO-), which are more potent toxic molecules, and might be responsible for inhibiting the meiotic maturation of follicular oocytes. The mechanism by which NO interferes in follicular oocytes maturation probably involves the generation of cyclic guanosine 3',5'-monophosphate (cGMP), the major mediator of NO [3]. cGMP has been implicated in the control of meiosis [41] and granulosa cell function [10]. In the present study, higher NO concentrations (10-3 and 10-5 M) blocked the maturation of follicular oocyte whereas lower concentrations (10-9 M) induced meiosis in follicular oocytes. These findings suggested that a physiological level of NO may be necessary to stimulate GVBD by reducing cGMP levels.
It has been reported that FSH is essential for the in vitro growth, estradiol secretion, and antrum development of bovine and buffalo preantral follicles [11,33]. In this study, we examined the effects of NO/NOS on FSH-induced steroidogenesis by incubation with the spent PF culture medium. Our results showed that higher concentrations of SNP (10-3, 10-5 and 10-7 M) significantly suppressed FSH-stimulated estradiol release. These results imply that NO might hinder follicular development via suppression of P450 aromatase activity in granulosa cells. The site of NO action and the detailed mechanisms by which NO inhibits ovarian steroidogenesis are not completely understood. Previous studies reported that NO donors inhibit FSH-induced estradiol production in human granulosa-luteal cells as well as porcine and bovine granulosa cells [8,22,36]. Snyder et al. [36] proposed that NO directly inhibits aromatase activity by binding to a sulfhydryl group near the active site of the enzyme. These findings suggest that the NO/NOS system may have an important role in the local regulation of ovarian hormonal function, follicle growth, and granulosa cell differentiation by directly affecting the granulosa cells. These findings also support the hypothesis that NO is an autocrine/paracrine regulator of granulosa cell functions and concur with the results of a previous study [1].
L-NAME is a competitive inhibitor of NOS which selectively inhibits NO production by both eNOS and iNOS, but is more effective against eNOS [18,24]. In this study, supplementation of L-NAME with 10-5 M SNP enhanced the basal secretion of estradiol and progesterone. Thus, NOS activity is closely related to the regulation of steroidogenesis. These data strongly suggest that the growth of PFs and granulosa cell differentiation stimulated by FSH is enhanced by NOS inhibitors and suppressed by NO donors. Supplementation of Hb with 10-5 M SNP reversed the inhibitory effect of SNP, suggesting that Hb scavenges excess NO and maintains the physiological level of NO necessary for PF growth and development.
NO controls programmed cell death (apoptosis) by upregulating the tumor suppressor p53 gene, changing the expression of pro- and anti-apoptotic Bcl-2 family members, activating caspases, and promoting chromatin condensation and DNA fragmentation. A TUNEL assay was performed to demonstrate that the NO donor, SNP (10-3 and 10-5 M), induced apoptosis in follicular oocytes. Higher levels of NO in follicular oocytes might generate free radicals which interfere with DNA replication and lead to increased DNA fragmentation. Moreover, NO is known to affect the expression of genes related to cell cycle regulation and apoptosis [33]. The role of NO in apoptosis is controversial; this may be due to the fact that NO can be either toxic or protective depending on the cell type and NO concentrations. It is difficult to measure unstable free NO radical. Therefore, the end products of NO metabolism, nitrite and nitrates, are widely used as indicators of NO production and NOS activity. At higher concentrations, NO induces apoptosis and/or necrosis in rat ovaries [7], human epithelial-endometrial cells [20], and cattle embryos [27]. NO in sufficient concentrations competes with oxygen and binds to the heme groups in the respiratory chain enzymes as well as cytochrome oxidase [29]. Results of the present study showed that the higher levels of nitrite and nitrate in the PF culture medium (from the 10-3 and 10-5 M SNP-treated groups), indicating that greater NO concentrations did not protect PFs from cellular and mitochondrial damage. Thus, the present data suggest that mitochondrial damage caused by the toxic effects of higher NO concentrations could be interlinked with apoptosis in follicular cells as well as oocytes.
In conclusion, our results demonstrated that higher concentrations of SNP (10-3, 10-5, and 10-7 M) exert cytotoxic effects which can lead to cell death whereas 10-9 M SNP stimulated the survival, growth, and antrum formation of PFs. NO was found to have a dual effect on buffalo PFs depending on its concentration in the culture medium. These findings indicated that physiological levels of NO are involved in or required for the development of buffalo PFs.
Figures and Tables
![]() | Fig. 1Effect of SNP (0, 10-9, 10-7, 10-5, and 10-3 M) with or without L-NAME (1.0 mM) and Hb (1.0 µg) on the accumulation of estradiol in the buffalo PF culture medium during different culturing days. Lines with different superscript letters differ significantly (p < 0.05) when comparing different treatments on the same day. SNP: sodium nitroprusside, L-NAME: Nω-nitro-L-arginine methyl ester, Hb: hemoglobin. Each value represents the means ± SE of a total of three measurement from five independent experiments. |
![]() | Fig. 2Effect of SNP (0, 10-9, 10-7, 10-5, and 10-3 M) with or without L-NAME (1.0 mM) and Hb (1.0 µg) on the accumulation of progesterone in the PF culture media on different culturing days. Lines with different superscript letters differ significantly (p < 0.05) when comparing different treatments on the same day. Each value represents the means ± SE of a total of three measurements from five independent experiments. |
![]() | Fig. 3Effect of SNP (0, 10-9, 10-7, 10-5, and 10-3 M) with or without L-NAME (1.0 mM) and Hb (1.0 µg/mL) on NO-3/NO-2 concentrations in the PF culture medium on different culturing days. Values with different superscripts letters differ significantly (p < 0.05) when comparing different treatments on the same day. Each value represents the means ± SE of a total of three measurement from five independents experiments. |
![]() | Fig. 4
In vitro development of buffalo PFs cultured with SNP (10-9 M). (A) PFs showing centrally located oocyte surrounded by layers of granulosa cells on day 0. (B) In vitro growth of PFs after 6 days of culturing. (C) PFs showing signs of antrum formation (a) after 8 days of culturing. (D) Initiation of antrum formation after 10 days of culturing. (E) PFs showing antral cavity (a) after 12 days of culturing. (F) Extrusion of oocytes (o) after 15 days of culturing. Scale bars = 50 µm. |
![]() | Fig. 5Effect of different concentrations of SNP on in vitro antrum formation in cultured buffalo PFs. The percentages were subjected to arcsine transformation and analyzed by ANOVA followed by Duncan's multiple range test. Asterisks on the bars denote significant differences compared to the control group (p < 0.05). The sample size of each group is 39, 38, 46 and 47 from left to right, respectively. |
![]() | Fig. 6Detection of apoptosis in oocytes extruded from buffalo PFs cultured in vitro. (A) Control group oocyte showing TUNEL-negative staining. (B) SNP (10-3 M)-treated PFs oocyte showing TUNEL-positive staining (nuclear brown staining; arrow). Scale bars = 50 µm. |
Table 1
Effect of different SNP concentrations on in vitro survival of buffalo preantral follicles during different culture periods measured by trypan blue dye exclusion
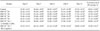
Acknowledgments
This study was financially supported by the Department of Biotechnology, Ministry of Science and Technology of the Government of India. Authors would like to thank the Director of the Indian Veterinary Research Institute for providing the necessary facilities to carry out this work. Authors are thankful to K.V.H. Sastry for providing the copper-cadmium alloy for the measurement of nitrate/nitrite. None of the authors of this paper have any financial or personal conflicts of interest that may have influenced this research.
References
1. Basini G, Baratta M, Ponderato N, Bussolati S, Tamanini C. Is nitric oxide an autocrine modulator of bovine granulosa cell function? Reprod Fertil Dev. 1998. 10:471–478.


2. Bilodeau-Goeseels S. Effects of manipulating the nitric oxide/cyclic GMP pathway on bovine oocyte meiotic resumption in vitro. Theriogenology. 2007. 68:693–701.


3. Blaise GA, Gauvin D, Gangal M, Authier S. Nitric oxide, cell signaling and cell death. Toxicology. 2005. 208:177–192.


4. Bu S, Xia G, Tao Y, Lei L, Zhou B. Dual effects of nitric oxide on meiotic maturation of mouse cumulus cell-enclosed oocytes in vitro. Mol Cell Endocrinol. 2003. 207:21–30.


5. Chaube SK, Dubey PK, Mishra SK, Shrivastav TG. Verapamil reversibly inhibits spontaneous parthenogenetic activation in aged rat eggs cultured in vitro. Cloning Stem Cells. 2007. 9:608–617.


6. Chun SY, Eisenhauer KM, Kubo M, Hsueh AJ. Interleukin-1 beta suppresses apoptosis in rat ovarian follicles by increasing nitric oxide production. Endocrinology. 1995. 136:3120–3127.


7. Ellman C, Corbett JA, Misko TP, McDaniel M, Beckerman KP. Nitric oxide mediates interleukin-1-induced cellular cytotoxicity in the rat ovary. A potential role for nitric oxide in the ovulatory process. J Clin Invest. 1993. 92:3053–3056.


8. Faes MR, Caldas-Bussiere MC, Viana KS, Dias BL, Costa FR, Escocard RM. Nitric oxide regulates steroid synthesis by bovine antral granulosa cells in a chemically defined medium. Anim Reprod Sci. 2009. 110:222–236.


9. Goud PT, Goud AP, Diamond MP, Gonik B, Abu-Soud HM. Nitric oxide extends the oocyte temporal window for optimal fertilization. Free Radic Biol Med. 2008. 45:453–459.


10. Grasselli F, Ponderato N, Basini G, Tamanini C. Nitric oxide synthase expression and nitric oxide/cyclic GMP pathway in swine granulosa cells. Domest Anim Endocrinol. 2001. 20:241–252.


11. Gutierrez CG, Ralph JH, Telfer EE, Wilmut I, Webb R. Growth and antrum formation of bovine preantral follicles in long-term culture in vitro. Biol Reprod. 2000. 62:1322–1328.


12. Hanke CJ, Drewett JG, Myers CR, Campbell WB. Nitric oxide inhibits aldosterone synthesis by a guanylyl cyclase-independent effect. Endocrinology. 1998. 139:4053–4060.


13. Hsueh AJW, Billig H, Tsafriri A. Ovarian follicle atresia: a hormonally controlled apoptotic process. Endocr Rev. 1994. 15:707–724.


14. Jablonka-Shariff A, Olson LM. Nitric oxide is essential for optimal meiotic maturation of murine cumulus-oocyte complexes in vitro. Mol Reprod Dev. 2000. 55:412–421.


15. Jablonka-Shariff A, Olson LM. The role of nitric oxide in oocyte meiotic maturation and ovulation: meiotic abnormalities of endothelial nitric oxide synthase knock-out mouse oocytes. Endocrinology. 1998. 139:2944–2954.


16. Jablonka-Shariff A, Basuray R, Olson LM. Inhibitors of nitric oxide synthase influence oocyte maturation in rats. J Soc Gynecol Investig. 1999. 6:95–101.


18. Lamas S, Marsden PA, Li GK, Tempst P, Michel T. Endothelial nitric oxide synthase: molecular cloning and characterization of a distinct constitutive enzyme isoform. Proc Natl Acad Sci USA. 1992. 89:6348–6352.


20. Li HY, Chang SP, Yuan CC, Chao HT, Ng HT, Sung YJ. Nitric oxide induces extensive apoptosis in endometrial epithelial cells in the presence of progesterone: involvement of mitogen-activated protein kinase pathways. Mol Hum Reprod. 2001. 7:755–763.


21. Mao J, Wu G, Smith MF, McCauley TC, Cantley TC, Prather RS, Didion BA, Day BN. Effects of culture medium, serum type, and various concentrations of follicle-stimulating hormone on porcine preantral follicular development and antrum formation in vitro. Biol Reprod. 2002. 67:1197–1203.


22. Masuda M, Kubota T, Kamada S, Aso T. Nitric oxide inhibits steroidogenesis in cultured porcine granulosa cells. Mol Hum Reprod. 1997. 3:285–292.


23. Matta SGC, Caldas-Bussiere MC, Viana KS, Faes MR, Paes de Carvalho CS, Dias BL, Quirino CR. Effect of inhibition of synthesis of inducible nitric oxide synthase-derived nitric oxide by aminoguanidine on the in vitro maturation of oocyte-cumulus complexes of cattle. Anim Reprod Sci. 2009. 111:189–201.


24. Moncada S, Palmer RM, Higgs EA. Nitric oxide: physiology, pathophysiology, and pharmacology. Pharmacol Rev. 1991. 43:109–142.
25. Morris SM Jr, Billiar TR. New insights into the regulation of inducible nitric oxide synthesis. Am J Physiol. 1994. 266:E829–E839.


26. Olson LM, Jablonka-Shariff A, Beltsos AN. Adashi EY, editor. Ovarian nitric oxide: a modulator of ovulation and oocyte maturation. Ovulation: Evolving Scientific and Clinical Concepts. 2000. New York: Springer;243–254.


27. Orsi NM. Embryotoxicity of the nitric oxide donor sodium nitroprusside in preimplantation bovine embryons in vitro. Anim Reprod Sci. 2006. 91:225–236.


28. Pires PRL, Santos NP, Adona PR, Natori MM, Schwarz KRL, de Bem THC, Leal CLV. Endothelial and inducible nitric oxide synthases in oocytes of cattle. Anim Reprod Sci. 2009. 116:233–243.


29. Sarti P, Lendaro E, Ippoliti R, Bellelli A, Benedetti PA, Brunori M. Modulation of mitochondrial respiration by nitric oxide: investigation by single cell fluorescence microscopy. FASEB J. 1999. 13:191–197.


30. Sastry KVH, Moudgal RP, Mohan J, Tyagi JS, Rao GS. Spectrophotometric determination of serum nitrite and nitrate by copper-cadmium alloy. Anal Biochem. 2002. 306:79–82.


31. Schwarz KRL, Pires PRL, Adona PR, Câmara de Bem TH, Leal CLV. Influence of nitric oxide during maturation on bovine oocyte meiosis and embryo development in vitro. Reprod Fertil Dev. 2008. 20:529–536.


32. Sengoku K, Takuma N, Horikawa M, Tsuchiya K, Komori H, Sharifa D, Tamate K, Ishikawa M. Requirement of nitric oxide for murine oocyte maturation, embryo development, and trophoblast outgrowth in vitro. Mol Reprod Dev. 2001. 58:262–268.


33. Sharma GT, Dubey PK, Meur SK. Survival and developmental competence of buffalo preantral follicles using three dimensional collagen gel culture system. Anim Reprod Sci. 2009. 114:115–124.


34. Sharma GT, Dubey PK, Meur SK. Effect of different mechanical isolation techniques on developmental competence and survival of buffalo ovarian preantral follicles. Livest Sci. 2009. 123:300–305.


35. Sharma GT, Loganathasamy K. Effect of meiotic stages during in vitro maturation on the survival of vitrified-warmed buffalo oocytes. Vet Res Commun. 2007. 31:881–893.


36. Snyder GD, Holmes RW, Bates JN, Van Voorhis BJ. Nitric oxide inhibits aromatase activity: mechanisms of action. J Steroid Biochem Mol Biol. 1996. 58:63–69.


37. Tamanini C, Basini G, Grasselli F, Tirelli M. Nitric oxide and the ovary. J Anim Sci. 2003. 81:E Suppl 2. E1–E7.
38. Tao Y, Fu Z, Zhang MJ, Xia GL, Yang J, Xie HR. Immunohistochemical localization of inducible and endothelial nitric oxide synthase in porcine ovaries and effects of NO on antrum formation and oocyte meiotic maturation. Mol Cell Endocrinol. 2004. 222:93–103.


39. Van Voorhis BJ, Dunn MS, Snyder GD, Weiner CP. Nitric oxide: an autocrine regulator of human granulosa-luteal cell steroidogenesis. Endocrinology. 1994. 135:1799–1806.

