Abstract
Angiotensin-I converting enzyme (ACE) is a key regulator of blood pressure, electrolytes and fluid homeostasis through conversion of angiotensin I into angiotensin II. Recently, a genetic polymorphism of the ACE gene, which accounts for 47% of the variation of ACE activity in blood, has been advocated as a biomarker of athletic aptitude. Different methods of analysis and determination of ACE activity in plasma have been used in human and equine research without a consensus of a "gold standard" method. Different methods have often been used interchangeably or cited as being comparable in the existing literature; however, the actual agreement between assays has not been investigated. Therefore, in this study, we evaluated the level of agreement between three different assays using equine plasma obtained from 29 horses. Two spectrophotometric assays using Furylacryloyl-phenylalanyl-glycyl-glycine as substrate and one fluorimetric assay utilizing o-aminobenzoic acid-FRK-(Dnp)P-OH were employed. The results revealed that the measurements from the different assays were not in agreement, indicating that the methods should not be used interchangeably for measurement of equine ACE activity. Rather, a single method of analysis should be adopted to achieve comparable results and critical appraisal of the literature is needed when attempting to compare results obtained from different assays.
Angiotensin I-converting enzyme (ACE) is responsible for the conversion of angiotensin I into angiotensin II and therefore plays a major role in blood pressure regulation and fluid and electrolyte homeostasis. ACE activity measurement in blood samples is a useful tool in human medicine for the detection and treatment monitoring of diseases such as sarcoidosis and hypertension. In small animal veterinary medicine, blood ACE is used to monitor hypertension and heart failure [4]. In horses, elevated blood ACE levels have been found to be associated with endometrial disease [15], gestational stage [23] and laminitis [16]. ACE levels in blood obtained from human athletes have been correlated with a genetic polymorphism and aptitude for endurance sports [19,21]. This polymorphism accounts for almost 50% of the variation in ACE activity in plasma [25,30]. Similar genetic polymorphisms have been identified in horses [12], and research results have demonstrated an inverse correlation between ACE activity in the blood and the distance successfully raced by Thoroughbred racehorses [10]. These recent developments warranted further investigation to test whether ACE activity in equine blood could be used as a marker of performance and fitness, and therefore an assessment of the agreement between existing ACE assays for use in horses was relevant. Several methods for measuring ACE in plasma and serum samples have been described for human beings. Some of these, which employ substrates such as hippuryl-histidyl-leucine (HHL) [14,20] and 3H-hippuryl-glycyl-glycine [29] and use a change in ultraviolet absorbance to measure the kinetics [16], have previously been described for use in equine serum or plasma. Recently, Alves et al. [1] validated the use of the fluorescence resonance energy transfer (FRET) substrate o-aminobenzoic acid-FRK(Dnp)P-OH (referred to as ABZ) [2] for assaying human ACE levels and established that there is a good correlation between a spectrophotometric method employing HHL as the substrate and the fluorimetric method using the ABZ substrate in humans [1,27]. Other methods and their applications can be found in the literature [1,3,11,14,17,20,22,24,28,29]. The objective of this study was not to validate the methods used for equine samples, since that has been done previously. Rather, this study was conducted to determine whether the results obtained using different methods were comparable and to assess the level of agreement between methods for measuring ACE activity in equine plasma. To accomplish this, we compared three different assays previously utilized in human research.
The population studied comprised 29 horses, of which 26 (90%) were Thoroughbreds, two (7%) were mixed breeds and one (3%) was a Standardbred. The ages of the horses ranged from 3 to 16 years and there were 16 males (13 geldings and 3 colts) and 13 females. Blood samples (10 mL per horse) were collected between 7 AM and 10 AM by simple venipuncture from one of the jugular veins into heparinized vacuum tubes. The blood was immediately placed and then kept on ice and promptly centrifuged at 3,400 × g for 10 min in a refrigerated centrifuge (GS15R; Beckman, Australia) at 4℃. The resulting plasma was harvested and transferred with sterile disposable plastic pipettes in aliquots into labeled microtubes (Eppendorf, Australia). The sample aliquots were snap-frozen in liquid nitrogen and stored in a -70℃ freezer until analysis. Each aliquot was used once, after a single cycle of thawing. Sample aliquots were not thawed and refrozen. From collection to freezing, the process took a maximum of 2 h.
This method utilized kinetic spectrophotometry and a commercially available assay kit, ACE Infinity (Thermo-Fisher, USA), developed for ACE measurement in both plasma and serum. This assay is based on the chemical reaction between ACE and the substrate furylacryloyl-phenylalanyl-glycyl-glycine (FAPGG), which causes a decrease in absorbance at 340 nm. The method employs a ready-to-use calibrator and substrate reagents and uses 30 µL of plasma and 300 µL of substrate for each sample analyzed. The linearity of the assay is between 1 and 120 U/L and the sensitivity is described by the manufacturer as 0.084ΔmA/min per U/L. Calibration methodology for the spectrophotometer is provided by the kit manufacturer.
This method is also a kinetic spectrophotometric method based on the chemical reaction between ACE and FAPGG (F7131; Sigma-Aldrich, Australia). The method utilizes raw chemicals instead of a pre-assembled assay kit. Samples must be run with both blanks and substrate reagent. The method also requires preparation of an HEPES buffer. The analytical range for ACE activity varies from 5~200 U/L. Hydrolysis of the substrate causes a decrease in absorbance at 340 nm. The complete description of the method has been published elsewhere [5,26]. Methods 1 and 2 require a 5 min incubation period at 37℃ and the results are given in U/L (based on the fact that 1 unit of ACE converts 1 µmol of FAPGG/min at 37℃).
This method uses the FRET substrate Abz-FRK(Dnp)P-OH (A4980; Sigma-Aldrich, Australia) and requires calibration of the fluorimeter with respect to the concentration of Abz-FRK(Dnp)P-OH to guarantee 10 µM of substrate in each sample analysed. We used a fluorimeter (Cary Varian, Australia) with chamber temperature control and constant magnetic stirring. This method provides real time kinetic readings of enzyme activity utilizing wavelengths of 320 nm (excitation) and 420 nm (emission). The results are given in arbitrary fluorescent units and have to be converted into U/L by finding the conversion constant of the equipment through complete hydrolysis of the substrate by a known concentration of commercially available enzyme in pure form, rabbit lung enzyme (A6778; Sigma-Aldrich, Australia). The complete method has been described elsewhere [1,8,27]. We used the average of readings taken for 310 sec for comparison with the ACEIn and FAPGG method. To evaluate the precision of the methods, repeatability studies were conducted in 33 samples obtained specifically for this purpose. Collection and processing were similar to that for the samples used in the agreement study. The analytical coefficient of variation (CV), within-run (CVw), and between-run (CVb) coefficients of variation were calculated using ANOVA and the "between/within" capability of the statistical software (Minitab 15.1.30.0; Minitab, USA). The sensitivity of each substrate was established based on the Kcat/Km values found in the literature [7,8]. The results from the different methods were compared using the Bland and Altman method [6,18] to determine the limits of agreement and confidence intervals as per the MedCalc v11.3.0 software (MedCalc, Belgium) and WinPepi v10.5 software (Brixton Health, UK). Acceptable agreement was considered to have occurred when two ACE measurements derived from two methods were within 5 to 10 U/L of each other in 95% of the occasions. This was based on the mean value obtained from each method, and represents a difference of 10% of the mean. Coefficients of determination (r2) were calculated to investigate linearity and association. Statistical significance of a quadratic coefficient was also assessed.
The mean ± SD of 29 samples was 69.0 ± 16.5 U/L for the ACEIn method, 89.8 ± 19.5 U/L for FAPGG method and 85.1 ± 24.8 U/L for the ABZ method. The CV for each method was 4.3% for the ACEIn method, 2.8% for the FAPGG method and 13.6% for the ABZ method. The respective CVw and CVb values were 2.7% and 10.1% for the ACEIn method, 2.5% and 14.5% for the FAPGG method and 9.5% and 14.8% for the ABZ method. Complete ACE activity inhibition was achieved in the ABZ method by adding captopril to the samples before analysis. Ninety eight percent of the ACE activity was inhibited by EDTA in the ACEIn method, while 97.7% was inhibited in the FAPGG method. The results (Figs. 1, 2, 3) showed that measurements of ACE acquired by different methods had poor agreement. For the FAPGG method and ACEIn method, the lower limit of agreement was -8.4 (95%CI -18.2 to 1.4 U/L) and the upper limit of agreement was 49.9 (95%CI 40.2 to 59.7 U/L). For the FAPGG method and ABZ method, the lower limit of agreement was -28.8 (95%CI -40.1 to -17.6 U/L) and the upper limit of agreement was 38.3 (95%CI 27.0 to 49.6 U/L). Finally, for the ABZ method and the ACEIn method, the lower limit of agreement was -26.3 (95%CI -40.6 to -12.1 U/L) and the upper limit of agreement was 58.4 (95%CI 44.2 to 72.6 U/L). The correlation between the difference and average was 0.23 (p = 0.24) for the FAPGG and ACEIn methods, -0.33 (p = 0.08) for the FAPGG and ABZ methods, and 0.44 (p = 0.02) for the ABZ and ACEIn methods. Removal of the observation with the greatest difference between the ABZ method and ACEIn method (difference of 67.6) resulted in the correlation between the difference and average being 0.34 (p = 0.08). Coefficients of determination were 0.45 between the ACEIn method and FAPGG method, 0.26 between the ABZ method and ACEIn method and 0.53 between the ABZ method and FAPGG method. None of the non-linear quadratic coefficients were significant (p values > 0.75).
In the past, measurements obtained from different methods in horses were compared [9,20,29] with conclusions being drawn partially based on these results. Normality ranges obtained by one method [29] were assumed to be valid for other methods, and established as reference values. However, in humans, it has been demonstrated that the high intra-individual variation of ACE activity in blood precludes the use of a single reference interval for determination of normality [13]. Furthermore, as is the case with biological products such as enzymes, each method produces its own reference ranges within a wider populational normality range. Therefore, it seems inappropriate to cross-utilize values obtained by one methodology as references for all methods. This study demonstrated that there is poor agreement between different methods of analysis of equine ACE activity in plasma; therefore, these methods should not be used interchangeably. Variation in the mean value of ACE observed with different methods is likely to come from the fact that different protocols (spectrophotometric and fluorimetric) and different substrates (ACEIn and FAPGG vs. ABZ) were used. Nevertheless, a degree of comparability between methods was expected. Judicious analysis of literature utilizing ACE results obtained from one method but utilizing ACE reference values obtained from a second method is necessary. Previous studies on how to determine method agreement for clinical measures have been published and we have followed those guidelines [6,18]. We propose that comparison between results for equine ACE activity obtained from different methods should not be attempted, since this may be misleading. In conclusion, the results of this study demonstrate that different assays have poor agreement and should not be used interchangeably. Accordingly, linear regression should not be used to establish a transformation equation to compare results from different methods, even for clinical purposes. In terms of the precision of each method, the FAPGG method and ACEIn method showed the lowest analytical CV and within-run variation, while the ACEIn method had the lowest between-run coefficient of variation. Overall, the results presented here indicate that it is critical to adhere to a single method of analysis to achieve comparability of samples.
Figures and Tables
Fig. 1
Bland and Altman plot showing the limits of agreement (two outer lines), 95% confidence interval of the mean difference (two lines on either side of the mean) and the mean difference between the furylacryloyl-phenylalanyl-glycyl-glycine (FAPGG) method and the angiotensin I-converting enzyme Infinity (ACEIn) method. n = 29.
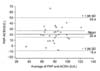
Fig. 2
Bland and Altman plot showing the limits of agreement (two outer lines), 95% confidence interval of the mean difference (two lines on either side of the mean) and the mean difference between the furylacryloyl-phenylalanyl-glycyl-glycine (FAPGG) method and the o-aminobenzoic acid-FRK(Dnp)P-OH (ABZ) method. n = 29.
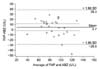
Fig. 3
Bland and Altman plot showing the limits of agreement (two outer lines), 95% confidence interval of the mean difference (two lines on either side of the mean) and the mean difference between the o-aminobenzoic acid-FRK(Dnp)P-OH (ABZ) method and angiotensin I-converting enzyme Infinity (ACEIn) method. n = 29.
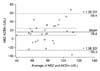
Acknowledgments
This research was funded by a Joint Collaborative Grant from The University of Melbourne (Australia) and UNIFESP (Brazil), and by The Pathology Section of The Faculty of Veterinary Science at The University of Melbourne.
References
1. Alves MF, Araujo MC, Juliano MA, Oliveira EM, Krieger JE, Casarini DE, Juliano L, Carmona AK. A continuous fluorescent assay for the determination of plasma and tissue angiotensin I-converting enzyme activity. Braz J Med Biol Res. 2005. 38:861–868.


2. Araujo MC, Melo RL, Cesari MH, Juliano MA, Juliano L, Carmona AK. Peptidase specificity characterization of C- and N-terminal catalytic sites of angiotensin I-converting enzyme. Biochemistry. 2000. 39:8519–8525.


3. Ball BA, Gravance CG, Wessel MT, Sabeur K. Activity of angiotensin-converting enzyme (ACE) in reproductive tissues of the stallion and effects of angiotensin II on sperm motility. Theriogenology. 2003. 59:901–914.


4. BENCH (BENazepril in Canine Heart disease) Study Group. The effect of benazepril on survival times and clinical signs of dogs with congestive heart failure: Results of a multicenter, prospective, randomized, double-blinded, placebo-controlled, long-term clinical trial. J Vet Cardiol. 1999. 1:7–18.
5. Beneteau B, Baudin B, Morgant G, Giboudeau J, Baumann FC. Automated kinetic assay of angiotensin-converting enzyme in serum. Clin Chem. 1986. 32:884–886.


6. Bland JM, Altman DG. Measuring agreement in method comparison studies. Stat Methods Med Res. 1999. 8:135–160.


7. Bramucci M, Miano A, Quassinti L, Maccari E, Murri O, Amici D. Presence and comparison of Angiotensin converting enzyme in commercial cell culture sera. Biochem Mol Biol Int. 1999. 47:107–115.


8. Carmona AK, Schwager SL, Juliano MA, Juliano L, Sturrock ED. A continuous fluorescence resonance energy transfer angiotensin I-converting enzyme assay. Nat Protoc. 2006. 1:1971–1976.


9. Coomer RPC, Forhead AJ, Bathe AP, Head MJ. Plasma angiotensin-converting enzyme (ACE) concentration in Thoroughbred racehorses. Equine Vet J. 2003. 35:96–98.


10. Costa MFM. Angiotensin-converting enzyme (ACE) in horses and its relationship to performance and fitness. Ph.D dissertation. 2010. Melbourne: The University of Melbourne.
11. Cushman DW, Cheung HS. Spectrophotometric assay and properties of the angiotensin-converting enzyme of rabbit lung. Biochem Pharmacol. 1971. 20:1637–1648.


12. Ellis N. Molecular genetic characterization of the equine angiotensin-converting enzyme gene. Faculty of Veterinary Science. 2005. Sydney: The University of Sydney;338.
13. Fogarty Y, Fraser CG, Browning MCK. Intra- and inter-individual variation of serum angiotensin converting enzyme: clinical implications. Ann Clin Biochem. 1989. 26:201–202.


14. Forhead AJ, Gillespie CE, Fowden AL. Role of cortisol in the ontogenic control of pulmonary and renal angiotensin-converting enzyme in fetal sheep near term. J Physiol. 2000. 526:409–416.


15. Ganjam VK, Evans TJ. Equine endometrial fibrosis correlates with 11β-HSD2, TGF-β1 and ACE activities. Mol Cell Endocrinol. 2006. 248:104–108.


16. Gardner SY, Atkins CE, Sams RA, Schwabenton AB, Papich MG. Characterization of the pharmacokinetic and pharmacodynamic properties of the angiotensin-converting enzyme inhibitor, enalapril, in horses. J Vet Intern Med. 2004. 18:231–237.


17. Hurst PL, Lovell-Smith CJ. Optimized assay for serum angiotensin-converting enzyme activity. Clin Chem. 1981. 27:2048–2052.


18. Mantha S, Roizen MF, Fleisher LA, Thisted R, Foss J. Comparing methods of clinical measurement: reporting standards for Bland and Altman analysis. Anesth Analg. 2000. 90:593–602.


19. Montgomery H, Clarkson P, Barnard M, Bell J, Brynes A, Dollery C, Hajnal J, Hemingway H, Mercer D, Jarman P, Marshall R, Prasad K, Rayson M, Saeed N, Talmud P, Thomas L, Jubb M, World M, Humphries S. Angiotensin-converting-enzyme gene insertion/deletion polymorphism and response to physical training. Lancet. 1999. 353:541–545.


20. Muir WW III, Sams RA, Hubbell JAE, Hinchcliff KW, Gadawski J. Effects of enalaprilat on cardiorespiratory, hemodynamic, and hematologic variables in exercising horses. Am J Vet Res. 2001. 62:1008–1013.


21. Myerson S, Hemingway H, Budget R, Martin J, Humphries S, Montgomery H. Human angiotensin I-converting enzyme gene and endurance performance. J Appl Physiol. 1999. 87:1313–1316.
22. Nocenti MR, Simchon S, Cizek LJ. Analysis of the renin-angiotensin system during fasting in adult male rabbits. Proc Soc Exp Biol Med. 1975. 150:142–147.


23. O'Connor SJ, Fowden AL, Holdstock N, Giussani DA, Forhead AJ. Developmental changes in pulmonary and renal angiotensin-converting enzyme concentration in fetal and neonatal horses. Reprod Fertil Dev. 2002. 14:413–417.
24. Raimbach SJ, Thomas AL. Renin and angiotensin converting enzyme concentrations in the fetal and neonatal guinea-pig. J Physiol. 1990. 423:441–451.


25. Rigat B, Hubert C, Alhenc-Gelas F, Cambien F, Corvol P, Soubrier F. An insertion/deletion polymorphism in the angiotensin I-converting enzyme gene accounting for half the variance of serum enzyme levels. J Clin Invest. 1990. 86:1343–1346.


26. Ronca-Testoni S. Direct spectrophotometric assay for Angiotensin-converting enzyme in serum. Clin Chem. 1983. 29:1093–1096.


27. Sabatini RA, Bersanetti PA, Farias SL, Juliano L, Juliano MA, Casarini DE, Carmona AK, Paiva ACM, Pesquero JB. Determination of angiotensin I-converting enzyme activity in cell culture using fluorescence resonance energy transfer peptides. Anal Biochem. 2007. 363:255–262.


28. Schweisfurth H, Schiöberg-Schiegnitz S. Assay and biochemical characterization of angiotensin-I-converting enzyme in cerebrospinal fluid. Enzyme. 1984. 32:12–19.


29. Tillman LG, Moore JN. Serum angiotensin converting enzyme activity and response to angiotensin I in horses. Equine Vet J. 1989. 21:Suppl 7. s80–s83.


30. Tiret L, Rigat B, Visvikis S, Breda C, Corvol P, Cambien F, Soubrier F. Evidence, from combined segregation and linkage analysis, that a variant of the angiotensin I-converting enzyme (ACE) gene controls plasma ACE levels. Am J Hum Genet. 1992. 51:197–205.