Abstract
This in vitro study evaluated the detrimental effect of acute gamma (γ)-irradiation on rat immature hippocampal neurons. Rat immature hippocampal neurons (0.5 day in vitro) were irradiated with 0~4 Gy γ-rays. Cytotoxicity was analyzed using a lactate dehydrogenase release assay at 24 h after γ-irradiation. Radiation-induced cytotoxicity in immature hippocampal neurons increased in a dose-dependent manner. Pre-treatments of pro-apoptotic caspase inhibitors and anti-oxidative substances significantly blocked γ-irradiation-induced cytotoxicity in immature hippocampal neurons. The results suggest that the caspase-dependent cytotoxicity of γ-rays in immature hippocampal cultured neurons may be caused by oxidative stress.
Ionizing radiation is a very useful tool in a wide variety of fields including medicine, agriculture, industry, research, and military operations. However, it has various effects on both the developing and adult brain, even though the adult brain displays less severe morphological changes to irradiation than other organs. A relatively low-dose of whole body exposure in experimental animals can produce transient damage to immature progenitor cells in specific tissues, including the brain [7]. Especially, under very low doses of radiation, the developing brain is one of the most radiosensitive organs [6,10].
In adults, although irradiation is one of the most effective therapies for treatment of head and neck tumors, this therapeutic application is limited due to potential damage of normal brain tissue [9]. Ionizing radiation induces apoptosis of neural immature progenitor cells in the subventricular zone of the lateral ventricles and in the subgranular zone of the hippocampal dentate gyrus in the adult brain [3,7]. The phenomenon in the adult mouse hippocampus may lead to hippocampal-dependent learning and memory impairment [7]. Even very low doses of irradiation to the brain of an infant before age 18 months can affect the patient's cognitive abilities in adulthood [5]. However, mechanistic studies of radiation-induced effects on cognitive function related to the hippocampus are very difficult because they rely on complicated in vivo models [2]. A previous in vitro study confirmed that the radiosensitivity of immature hippocampal cells is significantly higher than that of the mature cells, indicating that the susceptibility of such hippocampal cells depends on their differentiation [12]. Therefore, further study needs to address the specific mechanisms associated with radiation effects on immature hippocampal neurons by using in vitro approaches.
This study examined the cytotoxicity of acute gamma (γ)-irradiation in immature hippocampal neurons to clarify the precise mechanism(s) in the detrimental effect of ionizing radiation on immature hippocampal neurons in an in vitro system.
Pregnant Sprague-Dawley rats were obtained from a specific pathogen-free colony at Oriental Inc. (Korea). The method used to primary hippocampal neuron culture has been previously described in detail [12]. Briefly, hippocampi were dissected from postnatal day 0 rats. After dissection, tissues were chopped and digested with 10 units/mL papain (Worthington, USA) and 100 units/mL DNase I (Roche, Switzerland) in dissociation buffer at 37℃ for 30 min. The digestion was triturated with Neurobasal A medium (Invitrogen, USA). The cells were seeded at a density of 4 × 106 cells/well on poly-D-lysine hydrobromide (150 µg/mL; Sigma-Aldrich, USA) coated 4-well plates. Two hours after plating, Neurobasal A was replaced with growth medium including Neurobasal A, 1 × B27 supplement (Invitrogen, USA), 100 units/mL penicillin, 0.1 mg/mL streptomycin, and 0.5 mM glutamine.
Hippocampal cultured neurons [0.5 day in vitro (DIV)] on a cover slip were fixed with 4% paraformaldehyde (Sigma-Aldrich, USA) in phosphate-buffered saline (PBS, pH 7.4) for 20 min at room temperature. The cells were blocked with 2% normal goat serum and 1% bovine serum albumin, allowed to react with rabbit monoclonal anti-Ki-67 (DRM004, 1 : 200; Acris Antibodies GmbH, Germany) and rabbit polyclonal anti-doublecortin (DCX) antibody (1 : 400; Cell Signaling Technology, USA) overnight at 4℃, and incubated with tetramethylrhodamine isothiocyanate-conjugated goat anti-rabbit IgG (1 : 50; Sigma-Aldrich, USA) for 1 h at room temperature. The cells were incubated with either mouse monoclonal anti-nestin (1 : 200; Chemicon International, USA) or mouse monoclonal anti-glial fibrillary acidic protein (GFAP) antibody (1 : 500; Sigma-Aldrich, USA) for 1 h at room temperature, and then incubated with fluorescein isothiocyanate-conjugated goat anti-mouse IgG (1 : 50; Sigma-Aldrich, USA) for 1 h at room temperature. For counterstaining, 1 mg/mL 4',6-diamidino-2-phenylindole 2HCl (DAPI; Thermo Fisher Scientific, USA) in PBS was added and incubated at room temperature for 5 min. The immunofluorescence-stained specimens were examined using a ProgRes CFscan fluorescence digital camera (Jenoptik, Germany).
Cultured neurons at 0.5 DIV were irradiated with 0, 0.5, 2, or 4 Gy of 137Cs-generated γ-rays using a Gamma-cell Elan 3000 (Nordion International, Canada). The mean dose rate of the γ-rays was 3.1 Gy/min. Control samples were sham-irradiated with 0 Gy. The cells were assayed at 24 h post-irradiation. To evaluate the radioprotective effects of anti-oxidative substances, including amifostine (0~100 µM; a representative agent of radioprotactants; Sigma-Aldrich, USA) and epigallocatechin gallate (EGCG, 0~10 µM; the main ingredient of green tea polyphenols; Sigma-Aldrich, USA) on immature hippocampal neurons, both substances were pre-treated at 30 min before irradiation.
Cytotoxicity was evaluated using a lactate dehydrogenase (LDH) release assay. A commercially available LDH-cytotoxicity assay kit from Biovision (USA) was used as recommended by the manufacturer. The optical density values were quantified by an Emax microplate reader (Molecular Devices, USA) for absorbance at a wavelength of 490 nm. To monitor intracellular accumulation of ROS, the fluorescent probe 2',7'-dichlorodihydrofluorescein diacetate (H2DCF-DA; Invitrogen, USA) was used. Intracellular ROS production was quantified by a fluorescent microplate reader with excitation at 485 nm and emission at 535 nm.
Medium was removed and sodium dodecyl sulfate (SDS) sample buffer (4×) was added to each culture. The cells were scraped and sonicated for 10 sec. The samples were heated to 100℃ for 10 min. The samples were then separated by 10% SDS-polyacrylamide gel electrophoresis (SDS-PAGE), and the resolved proteins were transferred to a nitrocellulose membrane and blocked with 1% normal goat serum and 0.5% bovine serum albumin in PBS-T (PBS, 0.1% Tween 20) for 30 min at room temperature. The membranes were then incubated with a 1 : 1,000 dilution of either rabbit anti-cleaved (active) caspase-3 antibody (Cell Signaling Technology, USA) or rabbit anti-poly (ADP-ribose) polymerase (PARP) antibody (Cell Signaling Technology, USA) in PBS-T overnight at 4℃. After extensive washing and incubation with a 1 : 10,000 dilution of horseradish peroxidase-conjugated goat anti-rabbit IgG (Vector Laboratories, USA) for 1.5 h at room temperature, the signals were visualized using chemiluminescence methods (SuperSignal West Pico; Thermo Fisher Scientific, USA). For normalization purposes, the membranes were reprobed with a 1 : 20,000 dilution of β-actin antibody (Sigma-Aldrich, USA). Several exposure times were used to obtain the signals in the linear range. The bands were quantified using Scion Image Beta 4.0.2 for Windows XP software (Scion, USA).
To confirm the cell phenotypes of 0.5 DIV hippocampal cultured cells, double-immunofluorescent staining was performed. We first detected Ki-67, nestin, DCX and GFAP, which are immunohistochemical markers for proliferating cells, neural stem cells, immature progenitor neurons and astrocytes, respectively, in 0.5 DIV hippocampal cells. The double-immunofluorescent results showed that Ki-67-positive cells were co-localized to nestin immunoreactivity (approximately 15% of total cells; Figs. 1A~D), but DCX-positive cells (approximately 80% of total cells) were rarely matched with GFAP immunoreactivity (< 5% of total cells; Figs. 1E~H) in 0.5 DIV hippocampal cells.
The cytotoxicity of γ-irradiation in immature hippocampal cultured neurons was estimated 24 h after irradiation by 0~4 Gy by the LDH release assay (Fig. 2). The cytotoxicity increased in a dose-dependent pattern within the range of irradiation applied.
To determine whether radiation-induced cell death in immature hippocampal neurons was associated with the caspase-dependent pathway, caspase-3 activation and caspase-specific PARP cleavage were assessed by Western blot and the effects of caspase inhibitors on radiation-induced cytotoxicity were examined by LDH release assay (Fig. 3). Active caspase-3 and cleaved PARP markedly increased in immature hippocampal neurons at 24 h after 2 Gy γ-irradiation (Fig. 3A). However, both the caspase-family inhibitor Z-VAD-FMK and the caspase-3 specific inhibitor Z-DEVD-FMK significantly blocked LDH release from γ-irradiated immature hippocampal neurons (Fig. 3B).
We further examined whether anti-oxidative substances, including amifostine (well-known as a representative radioprotective agent [8]) and EGCG could inhibit the cytotoxicity of γ-rays in immature hippocampal neurons. Pre-treatment of amifostine and EGCG significantly blocked increase of LDH release (Figs. 4A and B) and intracellular ROS levels (Fig. 4C) caused by 2 Gy-irradiation.
In this study, we demonstrate the cytotoxicity of γ-rays in immature hippocampal neurons via the caspase-dependent pathway. Further, we suggest that the caspase-dependent cytotoxicity of γ-rays in immature hippocampal cultured neurons may be caused by oxidative stress.
An irradiation study using developing rat brains suggested that the radiosensitive population consists primarily of cells nearing cell division or cells that have recently completed mitosis and are beginning to differentiate [1]. Viability of immature hippocampal cells, but not mature cells, can significantly decline upon exposure to γ-rays, indicating that neuronal resistance to γ-irradiation is associated with neuronal differentiation [11,12]. Furthermore, cultured hippocampal cells form functional synapses and a network after 3~5 DIV [13]. Therefore, we chose to apply γ-irradiation to 0.5 DIV-cultured hippocampal neurons, which are comparable to neural immature progenitor neurons before cell connection and synapse formation.
In addition, this study detected immunohistochemical markers for proliferating cells, neural stem cells, immature progenitor neurons, and astrocytes in 0.5 DIV hippocampal cells. This suggests that rat immature hippocampal cells in this in vitro system, which have > 95% immature progenitor neural cells, can be very useful for screening radioprotectants and studying various neuronal perturbation factors caused by radiation exposure.
Exposure of mammalian brains to ionizing radiation induces apoptosis of neural immature progenitor cells in vivo [3]. DNA-fragmentation and apoptotic appearance in immature hippocampal neuron are increased by γ-irradiation in a dose-dependent manner [12]. This study also showed that γ-ray-induced cytotoxicity in immature hippocampal neurons was increased in a dose-dependent pattern within the range of irradiation applied. Additionally, active caspase-3 and cleaved PARP were markedly increased in immature hippocampal neurons with 2 Gy γ-irradiation. Furthermore, both the caspase-family inhibitor Z-VAD-FMK and the caspase-3 specific inhibitor Z-DEVD-FMK significantly blocked radiation-induced cytotoxicity in immature hippocampal neurons. This suggests that γ-irradiation-induced cell death in immature hippocampal neurons depends on a pro-apoptotic caspase pathway, especially the caspase-3 related pathway.
Exposure of ionizing radiation to the central nervous system induces neuronal apoptosis via oxidative stress [4]. Appropriately, an experiment examined whether anti-oxidative substances could inhibit the γ-ray-induced cytotoxicity in immature hippocampal neurons. In this study, pre-treatment of amifostine and EGCG significantly blocked γ-ray-induced LDH release and intracellular ROS levels. These results support the suggestion that oxidative stress is associated with the cytotoxicity of γ-rays in immature hippocampal cultured neurons. Additionally, this experiment confirmed a beneficial in vitro system for screening radioprotective compounds.
Collectively, this in vitro study has confirmed that the caspase-dependent cytotoxicity of γ-rays in immature hippocampal cultured neurons may be caused by oxidative stress. In addition, this study provides a useful tool for the analysis of critical mechanistic parameters associated with radiation response of the brain and to better understand the molecular changes in radiation-induced cell death of immature neural cells.
Figures and Tables
Fig. 1
Double-immunofluorescent images of Ki-67 and nestin (A~D) and doublecortin (DCX) and glial fibrillary acidic protein (GFAP) (E~H) in 0.5 day in vitro-cultured hippocampal neurons. (A) Immunolabeling of Ki-67 (red). (B) Immuno-labeling of nestin (green). (C) 4',6-diamidino-2-phenylindole (DAPI) staining (blue). (D) Merged photograph. (E) Immunolabeling of DCX (red). (F) Immuno-labeling of GFAP (green). (G) DAPI staining (blue). (H) Merged photograph. Scale bars = 30 µm.
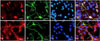
Fig. 2
Dose-dependent pattern of γ-ray-induced cytotoxicity in immature hippocampal neurons. (A) Lactate dehydrogenase (LDH) assay as an indicator of cytotoxicity of γ-rays (0~4 Gy) at 24 h after irradiation in hippocampal neurons of 0.5 DIV. (B and C) Differential interference contrast images revealed that cellular shrinkage and loss in irradiated cells (2 Gy) was increased compared with sham-irradiated controls. The data in (A) represent the mean ± SE, n = 3 cultures per condition. Scale bars = 30 µm.
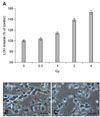
Fig. 3
Western blot analysis showing active caspase-3 and cleaved poly (ADP-ribose) polymerase (PARP) expression in immature hippocampal neurons with γ-irradiation (2 Gy). (A) Representative immunoblots for detection of active caspase-3 (17 kDa), PARP (118 kDa) and cleaved PARP (89 kDa) at 24 h after γ-irradiation (IR, 2 Gy). (B) 2 Gy γ-irradiated cells displayed significant increase of LDH in the medium. However, pre-treatment of the caspase-family inhibitor Z-VAD-FMK or the caspase-3 specific inhibitor Z-DEVD-FMK significantly inhibited release of LDH in the medium of 2 Gy-irradiated cells. The data represents the mean ± SE, n = 3 cultures per condition.
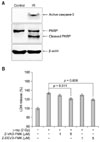
Fig. 4
The inhibitory effects of anti-oxidative substances on γ-ray-induced cytotoxicity and increase of intracellular reactive oxygen species (ROS) in immature hippocampal neurons. (A and B) Pre-treatments of anti-oxidative substances, amifostine (Ami) and epigallocatechin gallate (EGCG) at 30 min before irradiation significantly inhibited LDH release in immature hippocampal neurons with all of treated doses compared with that in 2 Gy-irradiated controls. (C) Pre-treatments of amifostine (100 µM) and EGCG (10 µM) significantly blocked cellular levels of ROS by 2 Gy-irradiation. The data represent the mean ± SE, n = 3 cultures per condition. *p < 0.05, **p < 0.01 vs. 2 Gy-irradiated controls.
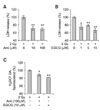
Acknowledgments
This study was financially supported by Special Research Program of Chonnam National University, 2009. This work was supported by the Grant of the Korean Ministry of Education, Science and Technology (The Regional Core Research Program/Biohousing Research Institute), Korea.
References
1. Bayer SA. Cellular aspects of brain development. Neurotoxicology. 1989. 10:307–320.
2. Fike JR, Rosi S, Limoli CL. Neural precursor cells and central nervous system radiation sensitivity. Semin Radiat Oncol. 2009. 19:122–132.


3. Fukuda H, Fukuda A, Zhu C, Korhonen L, Swanpalmer J, Hertzman S, Leist M, Lannering B, Lindholm D, Björk-Eriksson T, Marky I, Blomgren K. Irradiation-induced progenitor cell death in the developing brain is resistant to erythropoietin treatment and caspase inhibition. Cell Death Differ. 2004. 11:1166–1178.


4. Gobbel GT, Bellinzona M, Vogt AR, Gupta N, Fike JR, Chan PH. Response of postmitotic neurons to X-irradiation: implications for the role of DNA damage in neuronal apoptosis. J Neurosci. 1998. 18:147–155.


5. Hall P, Adami HO, Trichopoulos D, Pedersen NL, Lagiou P, Ekbom A, Ingvar M, Lundell M, Granath F. Effect of low doses of ionising radiation in infancy on cognitive function in adulthood: Swedish population based cohort study. BMJ. 2004. 328:19.


6. Hays SR, Li X, Kimler BF. Is there an adaptive response to radiation in the developing brain of the fetal rat? Radiat Res. 1993. 136:293–296.


7. Kim JS, Lee HJ, Kim JC, Kang SS, Bae CS, Shin T, Jin JK, Kim SH, Wang H, Moon C. Transient impairment of hippocampus-dependent learning and memory in relatively low-dose of acute radiation syndrome is associated with inhibition of hippocampal neurogenesis. J Radiat Res (Tokyo). 2008. 49:517–526.


8. McDonough JH, Mele PC, Franz CG. Comparison of behavioral and radioprotective effects of WR-2721 and WR-3689. Pharmacol Biochem Behav. 1992. 42:233–243.


9. Moore AH, Olschowka JA, Williams JP, Paige SL, O'Banion MK. Radiation-induced edema is dependent on cyclooxygenase 2 activity in mouse brain. Radiat Res. 2004. 161:153–160.


10. Packer RJ, Sutton LN, Atkins TE, Radcliffe J, Bunin GR, D'Angio G, Siegel KR, Schut L. A prospective study of cognitive function in children receiving whole-brain radiotherapy and chemotherapy: 2-year results. J Neurosurg. 1989. 70:707–713.


11. Shirai K, Mizui T, Suzuki Y, Kobayashi Y, Nakano T, Shirao T. Differential effects of x-irradiation on immature and mature hippocampal neurons in vitro. Neurosci Lett. 2006. 399:57–60.

