Abstract
This study was designed to investigate the potential estrogenic effects of perinatal dietary phytoestrogens on the rat uterus. Pregnant rats were divided to three groups provided the following diets: (1) rat chow, (2) rat chow with 7.5% Trifolium (T.) pratense, or (3) rat chow supplemented with 17β-estradiol (0.5 mg/kg). The dams in each group were kept on the same diet during pregnancy and lactation. Female offspring were euthanized on day 21 at which time body and organ weights were recorded and tissue samples were taken for histology. Immunohistochemistry was performed to detect estrogen receptor alpha (ERα) and progesterone receptor (PR) levels. Our results revealed estrogen-like biological effects of perinatal T. pratense exposure. Relative uterus and ovary weights in the experimental groups were increased compared to control. The number of uterine glands and luminal epithelium heights were also increased. However, there were no statistically significant changes detected in the immunostaining intensity of ERα and PR between the groups.
Trifolium (T.) pratense, a fodder plant, contains phytoestrogens that possess putative weak estrogenic or anti-estrogenic activity. T. pratense contains four important estrogenic isoflavones (biochanin A, formononetin, genistein, and daidzein) and coumestans [20]. Early studies demonstrated that isoflavone intake was associated with impaired fertility in sheep and silage-fed cows exposed to isoflavone-rich T. pratense [1,12]. Reportedly, even low concentrations of phytoestrogens may have substantial deleterious effects on the reproductive rate of sheep, and possibly cattle, or may manifest as subclinical infertility in the absence of any visible clinical signs [1].
T. pratense is important not only as animal feed but also as an alternative to conventional hormone replacement therapy due to its high isoflavone content [3]. Although phytoestrogens were shown to be responsible for fertility-related disorders in animals, they have also been found to be beneficial for certain diseases. Extracts obtained from T. pratense have been shown to inhibit aromatase activity, angiogenesis, and inflammation, and have been tested for treatment of breast and prostate cancers [11,15,19,24]. A number of clinical trials have been performed to address the effects of T. pratense on menopause, cognitive function, and bone and cardiovascular health with no agreement on their effectiveness [5,8,16]. Further studies are necessary to satisfy this controversy and evaluate the risks of long-term use of phytoestrogens. Phytoestrogens have generated considerable interest because of their potential cancer preventive, cardioprotective, and anti-osteoporotic affects, as well as their ability to relieve menopausal symptoms. There have been contradictory results published on the effects of isoflavones on a variety of estrogen-regulating organs postulating both beneficial and adverse effects on human health [25].
Animal studies have indicated that neonatal exposure to phytoestrogens and environmental estrogens may affect male and female reproductive development [2]. A soy isoflavone, genistein, was reported to modulate sex steroid receptor expression in prepubertal rat uteri when given at considerably high pharmacologic doses [9]. Moreover, extracts of T. pratense tested in an adult ovariectomized rat model were suggested to be weakly estrogenic [7] or to have no effect on rat uterus [18].
Compounds with estrogenic activity, which naturally occur in food products or fodder plants, may alter the reproductive performance of animals and may also have an impact on animal and/or human health. Therefore, experimental studies based on physiologic dose levels evaluating exposure at different periods of life are needed. In this study, we investigated whether perinatal exposure to T. pratense through dietary intake would alter the uterine health of prepubertal rats.
Adult, female Wistar albino rats (180~250 g; n = 15) and their female littermates (n = 40) were used for this study. The experimental protocol was approved by Istanbul University's Animal Care and Use Committee (Approval number: 18/13072001) consistent with the NIH Guidelines for the Care and Use of Laboratory Animals. The rats were maintained under standard laboratory conditions with a 12:12 light/dark cycle and free access to pellet feed and tap water.
T. pratense was gathered from local fields in the Marmara region of Turkey. The plant was identified by Associate Professor Dr. Tuna Ekim (Faculty of Pharmacy, University of Istanbul, Turkey) as T. pratense L. var. Pratense. A dried specimen was deposited in the Herbarium of Istanbul University, Faculty of Pharmacy, with registration number 39935. The areal part of T. pratense was dried in a non-humid room at 25℃ and then grinded with a blender. The pulverized plant was thoroughly mixed and stored in the dark until the time of use. The concentrations of different isoflavones in T. pratense were analyzed by Krenn et al. [14]. The extract was prepared by maceration with water and extraction of the free genins was accomplished with methanol under reflux. The concentrations of the isoflavones in our material were as follows: biochanin 0.3%, formononetin 0.27%, daidzein 0.01%, and genistein 0.01%.
The study consisted of three groups receiving different diets as follows: the control group received standard rat diet (Eris Yem, Turkey); the T. pratense group included pregnant rats fed the control diet mixed with pulverized T. pratense (75 g/kg diet); and the estradiol group had 0.5 mg estradiol [(17β)-estra-1,3,5(10)-triene-3,17-diol; Sigma, USA] dissolved in corn oil (Sigma, USA) added to 1 kg of the control diet (12.5 µg/rat/day). The dams were exposed to different diets from the seventh day of gestation throughout lactation until postpartum day 21 at which time the female littermates were euthanized. Daily diet consumption was recorded as ~25 g. Only female littermates were used in the experiment.
The uteri samples were fixed in Bouin's solution for 18~20 h. After fixation, the samples were dehydrated in ethanol, cleared in xylene, and embedded in paraffin. Tissue sections were cut at a thickness of 5 µm and stained with hematoxylin and eosin. Images were taken with a Microphot FX light microscope (Nikon, Japan) and digitized with a CCD camera (F10; Panasonic, Japan). Body and uteri weights were determined in all groups. Relative weights were calculated by dividing organ weights by body weights and multiplying by 10,000.
Histometric methods were used to measure uterine layer areas and uteri luminal epithelium heights. Each uterine horn was cut into four equal pieces and the number of uterine glands was counted from these different segments. For measurement of uterine layer area, digitalized cross sections of the uteri were transferred to the AutoCad 2002 program (Autodesk, USA) and a line was drawn around each uterine layer using the polyline tool. After transferring the same sections to the Corel Draw 12 program (Corel, USA), the freehand tool was used to measure the epithelial height at 4~6 different points in each section. Calibration was performed with a milimetric ruler (1 mm, 0.01 Div; Graticules Pyser-Sgi, UK), which was photographed with the same magnification as all other images. Measurements of luminal epithelial cell height were completed for 10 animals per group.
Progesterone receptor (PR) antibody (rabbit anti-human PR, recognizes both forms of PR; Dako, Denmark), and estrogen receptor alpha (ERα) antibody (monoclonal mouse anti-human ER; Dako, Denmark) were used for immunohistochemistry. Paraffin sections were dewaxed and rehydrated. After washing in water, the sections underwent antigen retrieval in a microwave oven in 10 mM sodium citrate buffer, pH 6.0, for 15 min. The sections were allowed to cool and then rinsed with phosphate buffered saline (PBS). Endogenic peroxidase activity was blocked with 1% H2O2 in PBS for 20 min. PR (1:400) and ERα (1:200) antibodies were diluted in PBS with 3% bovine serum albumin containing 0.05% Tween. The sections were incubated with primary antibodies overnight at +4℃, washed with PBS, and incubated with horseradish peroxidase conjugated Dako EnVisionTM systems (Dako, Denmark) for 30 min. The slides were rinsed twice with PBS and color was developed with diaminobenzidine substrate (Dako, Denmark). The sections were then counterstained with Mayer's hematoxylin, dehydrated, and mounted.
Slides were analyzed using light microscopy. The number and distribution of ERα and PR stainings were determined from uteri sections of five animals in each group at ×200 magnification. Uterine structures, namely luminal/glandular epithelium, circular/longitudinal muscle, and stroma, were analyzed. The proportional score of positively stained cells was evaluated and given a score of zero for no staining, 1 for weak staining, 2 for moderate staining, 3 for strong staining, and 4 for intense staining. The proportional score reflected the number of nuclear ERα and PR positive epithelial cells in the layers of the uteri.
Treatment of rats from gestation day 7 to postnatal day 21 with T. pratense or with estradiol, slightly decreased body weights, but this decrease was not statistically significant (Table 1). Relative ovaria weights were significantly increased only in the T. pratense group. T. pratense caused a statistically significant increase in relative uterine weights with a similar increase also found in the estradiol group (Table 1).
The number of uterine glands was significantly increased in animals treated with T. pratense or estradiol (Table 2). Luminal epithelial cell height was significantly increased in both T. pratense and esteradiol treated animals compared to control animals (Table 2) and endometrium volumes were significantly increased in both T. pratense and estradiol groups as well (Table 3).
ERα and PR positive nuclei were localized in the epithelial, stromal, and muscular cells of the uteri (Fig. 1). Uterine sections not receiving primary antibody showed no staining. Strong PR immunoactivity was observed in the luminal epithelium of uterine tubes but only faint staining was observed in T. pratense and estradiol treated groups (Fig. 1). The staining intensity of glandular, luminal, stromal, and muscular compartments for ERα in the uteri of rats was reduced in both T. pratense and estradiol treated animals compared to controls (Fig. 2A); however, this decrease was not statistically significant. PR staining intensity decreased in luminal and glandular compartments in T. pratense and estradiol treatment groups. No changes were observed in the staining intensity of muscular and stromal layers between the control and estradiol groups. Decreased stromal staining intensity was observed in the T. pratense group compared to the control group. No PR staining was observed in the muscular layer of T. pratense treated animals (Fig. 2B).
In the present study, the effects of dietary perinatal exposure to T. pratense were investigated in female rats. Dietary estradiol was used as a reference compound for estrogenicity. In addition to isoflavones, T. pratense contains other potentially important components including phenolic acids and flavonoids that may affect uterine health in combination with isoflavones. We administered T. pratense by adding it to the diet rather than giving it as an extract to simulate the consumption of isoflavones through diet.
In agreement with earlier data [14], HPLC analysis showed that biochanin A and formononetin were the most abundant isoflavones detected in T. pratense, followed by genistein and daidzein. Genistein is the major isoflavone found in soy. T. pratense differs from soy not only in its lower genistein content but also in its higher phytoestrogen content. The total amount of isoflavones found in T. pratense extract used in the present study (0.61%) was lower than the amount reported by Saloniemi et al. [21] (1~2.5%). The low isoflavone content of T. pratense used in the present study may be due to the extraction method, seasonal changes, temperature (e.g. cool weather during the growing season), phosphorus content of the soil, changes in ozone exposure, or differences among T. pratense varieties [4,21-23].
Both estradiol and T. pratense treatments slowed the growth of pups. Exposure to either estradiol or T. pratense throughout pregnancy until day 21 increased relative uterine weights, suggesting estrogenic activity. In vitro studies demonstrated that T. pratense extracts exhibit estrogenic activity in an estrogen-dependent MCF-7 cell proliferation assay and had preferential agonist activity towards ERβ [6]. In accordance with our results, 2~3 g dry matter per day was found to increase the weight of immature rat uteri [18]. In an ovariectomized uterotrophic adult rat model, T. pratense extract was reported to be weakly estrogenic; however, this effect was based mainly on the semi-quantitative results of the Allen-Doisy vaginal cell cornification assay. Although, the highest dose (750 mg/kg per day) of T. pratense extract slightly increased uterine weight, it is not clear whether this difference was statistically significant compared to the control group [7]. In another study, ovariectomized adult rats were treated for 21 days with 4, 40, or 400 mg/kg per day of T. pratense extract. Neither uterine weight nor vaginal cornification assay results were significantly altered [18]. It is possible that rats at this perinatal period are more sensitive to estrogenic stimuli than adults. Thus, the timing and/or route of administration of T. pratense may account for differences in results.
In the current study, morphometric analysis showed that the number of uterine glands found in the endometrium was significantly increased in both the T. pratense and estradiol groups. In accordance with our study, Foth and Cline [10] reported that diet containing soy protein isolates (SPI) given together with estradiol increased endometrial thickness, gland area, and epithelial proliferation in macaque monkeys. Interestingly, in their study, the effect of estradiol on proliferation was partially antagonized by SPI. In our study, the area of endometrium showed an increase in treated groups, but the total area (epithelium plus myometrium) of the uterus was not significantly different than that of the control group. Furthermore, the luminal epithelial cell height increased in both T. pratense and estradiol treated groups. In a study by Overk et al. [18], no data on luminal epithelial cells were presented in rats treated with T. pratense. Increased epithelial height indicates hypertrophy of the cells. Water imbibition, mainly in the endometrium, may be responsible for uterine weight increase even though histological observation indicated no evidence of edema in the uterine wall. Although we did not measure the proliferation index, it is also possible that increased cell proliferation in epithelial as well as stromal cells may be responsible for the increased thickness of the endometrium.
The expression of PR is under the control of estrogen and progesterone [13]. PR is a classic gene marker for estrogen action containing estrogen response elements in the 5' region. Estrogen increases PR expression while progesterone decreases its expression in most target tissues. Progesterone treatment can oppose the estrogen induction of PR and progesterone has been shown to decrease total PR expression in the uterus [17]. In the current study ERα and PR expression was detected by immunohistochemistry. Our results revealed that neither T. pratense nor estradiol significantly altered the expression of ERα or PR in the luminal and glandular epithelium, which could be due to the fact that only five animals from each group were analyzed. In a study by Cotroneo et al. [9], no significant alteration was detected in ERα expression by Western blot analysis or immunohistochemistry after dietary administration of genistein and estradiol to Sprague Dawley rats. However, a decrease in staining intensity was evident when pharmacological doses were given by injection. In line with our results, they demonstrated that PR expression was not altered by dietary genistein administration, but the injection of pharmacological doses of both genistein and estradiol increased PR expression as shown by Western blot analysis. Our results revealed that consumption of T. pratense via the oral maternal route caused estrogen-like biological effects on the genital organs of rats, namely increased uterine weight and luminal epithelial cell height. However, no significant changes were detected during immunohistochemical evaluation of ERα and PR levels.
Figures and Tables
Fig. 1
Immunohistochemical expression of estrogen receptor alpha (ERα) in the uterus and progesterone receptor (PR) in the uterus and uterine tube. M: myometrium, GE: glandular epithelium, E: endometrium, LE: luminal epithelium, L: lumen. Arrows: nuclear staining of ERα and PR. Counterstain with Mayer's hematoxylin. Scale bars = 50 µm.
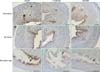
Fig. 2
ERα (A) and PR (B) immunostaining score in the uterus of 21-day-old rats. The proportion of positively stained cells was scored as follows: 0, none; 1, weak; 2, moderate; 3, strong; 4, intense staining.
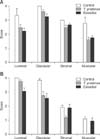
Acknowledgments
This work was supported by the Istanbul University Research Fund (Project No. T-1249/01112001). The authors thank Prof. L. Krenn for the HPLC analysis and Prof. H. Hakan Bozkurt and Dr. Basak Ulkay for their contributions to the study.
References
1. Adams NR. Detection of the effects of phytoestrogens on Sheep and Cattle. J Anim Sci. 1995. 73:1509–1515.


2. Atanassova N, McKinnell C, Walker M, Turner KJ, Fisher JS, Morley M, Millar MR, Groome NP, Sharpe RM. Permanent effects of neonatal estrogen exposure in rats on reproductive hormone levels, Sertoli cell number, and the efficiency of spermatogenesis in adulthood. Endocrinology. 1999. 140:5364–5373.


3. Beck V, Rohr U, Jungbauer A. Phytoestrogens derived from red clover: an alternative to estrogen replacement therapy? J Steroid Biochem Mol Biol. 2005. 94:499–518.


4. Booth NL, Overk CR, Yao P, Totura S, Deng Y, Hedayat AS, Bolton JL, Pauli GF, Farnsworth NR. Seasonal variation of red clover (Trifolium pratense L., Fabaceae) isoflavones and estrogenic activity. J Agric Food Chem. 2006. 54:1277–1282.


5. Booth NL, Piersen CE, Banuvar S, Geller SE, Shulman LP, Farnsworth NR. Clinical studies of red clover (Trifolium pratense) dietary supplements in menopause: a literature review. Menopause. 2006. 13:251–264.


6. Boué SM, Wiese TE, Nehls S, Burow ME, Elliott S, Carter-Wientjes CH, Shih BY, McLachlan JA, Cleveland TE. Evaluation of the estrogenic effects of legume extracts containing phytoestrogens. J Agric Food Chem. 2003. 51:2193–2199.


7. Burdette JE, Liu J, Lantvit D, Lim E, Booth N, Bhat KP, Hedayat S, Van Breemen RB, Constantinou AI, Pezzuto JM, Farnsworth NR, Bolton JL. Trifolium pratense (red clover) exhibits estrogenic effects in vivo in ovariectomized Sprague-Dawley rats. J Nutr. 2002. 132:27–30.


8. Coon JT, Pittler MH, Ernst E. Trifolium pratense isoflavones in the treatment of menopausal hot flushes: a systematic review and meta-analysis. Phytomedicine. 2007. 14:153–159.


9. Cotroneo MS, Wang J, Eltoum IA, Lamartiniere CA. Sex steroid receptor regulation by genistein in the prepubertal rat uterus. Mol Cell Endocrinol. 2001. 173:135–145.


10. Foth D, Cline JM. Effects of mammalian and plant estrogens on mammary glands and uteri of macaques. Am J Clin Nutr. 1998. 68:6 Suppl. 1413S–1417S.


11. Jarred RA, Keikha M, Dowling C, McPherson SJ, Clare AM, Husband AJ, Pedersen JS, Frydenberg M, Risbridger GP. Induction of apoptosis in low to moderate-grade human prostate carcinoma by red clover-derived dietary isoflavones. Cancer Epidemiol Biomarkers Prev. 2002. 11:1689–1696.
12. Kallela K, Heinonen K, Saloniemi H. Plant estrogens; The cause of decreased fertility in cows. A case report. Nord Vet Med. 1984. 36:124–129.
13. Katzenellenbogen BS, Nardulli AM, Read LD. Estrogen regulation of proliferation and hormonal modulation of estrogen and progesterone receptor biosynthesis and degradation in target cells. Prog Clin Biol Res. 1990. 322:201–211.
14. Krenn L, Unterrieder I, Ruprechter R. Quantification of isoflavones in red clover by high-performance liquid chromatography. J Chromatogr B Analyt Technol Biomed Life Sci. 2002. 777:123–128.


15. Krenn L, Paper DH. Inhibition of angiogenesis and inflammation by an extract of red clover (Trifolium pratense L.). Phytomedicine. 2009. 16:1083–1088.


16. Lethaby AE, Brown J, Marjoribanks J, Kronenberg F, Roberts H, Eden J. Phytoestrogens for vasomotor menopausal symptoms. Cochrane Database Syst Rev. 2007. CD001395.


17. Milgrom E, Thi L, Atger M, Baulieu EE. Mechanisms regulating the concentration and the conformation of progesterone receptor(s) in the uterus. J Biol Chem. 1973. 248:6366–6374.


18. Overk CR, Guo J, Chadwick LR, Lantvit DD, Minassi A, Appendino G, Chen SN, Lankin DC, Farnsworth NR, Pauli GF, van Breemen RB, Bolton JL. In vivo estrogenic comparisons of Trifolium pratense (red clover) Humulus lupulus (hops), and the pure compounds isoxanthohumol and 8-prenylnaringenin. Chem Biol Interact. 2008. 176:30–39.


20. Sabudak T, Guler N. Trifolium L. - A review on its phytochemical and pharmacological profile. Phytother Res. 2009. 23:439–446.
21. Saloniemi H, Wähälä K, Nykänen-Kurki P, Kallela K, Saastamoinen I. Phytoestrogen content and estrogenic effect of legume fodder. Proc Soc Exp Biol Med. 1995. 208:13–17.


22. Saviranta NMM, Anttonen MJ, von Wright A, Karjalainen RO. Red clover (Trifolium pratense L.) isoflavones: determination of concentrations by plant stage, flower colour, plant part and cultivar. J Sci Food Agric. 2008. 88:125–132.


23. Sivesind E, Seguin P. Effects of the environment, cultivar, maturity, and preservation method on red clover isoflavone concentration. J Agric Food Chem. 2005. 53:6397–6402.

