Abstract
Interleukin (IL)-10 exerts potent anti-inflammatory effects by suppression of both T-help (Th) 1 and Th2 cells. Previous studies have reported that IL-10 can ameliorate various inflammatory disorders. The present study was performed to examine whether IL-10 plasmid DNA could suppress development of atopic dermatitis (AD)-like skin lesions in NC/Nga mice, as an initial step towards the development of an appliance for use in dogs with AD. Intradermal injection of IL-10 plasmid DNA markedly inhibited the development of AD-like skin lesions, as evidenced by a marked decrease in skin symptoms and reduced inflammation within the skin lesions. Efficacy was confirmed by significant decreases in eosinophil ratio and serum IgE concentration, and a reduction in the number of Staphylococcus aureus recovered from the ear. Moreover, relative mRNA expression levels of IL-4 and interferon-γ in the skin lesions of mice injected with IL-10 plasmid DNA were also decreased compared with those of control mice. Of note, higher serum IL-10 levels in mice injected with IL-10 plasmid DNA were maintained compared with those in control mice. Taken together, the results indicate that IL-10 plasmid DNA can suppress the development of AD-like skin lesions by suppressing both Th1 and Th2 cell responses. Beneficial effects of IL-10 plasmid DNA may be expected in dogs with AD.
Canine atopic dermatitis (AD) is one of the most common inflammatory skin diseases in small-animal practice [11, 18]. Severe pruritus and chronic or relapsing eczematous skin lesions are major clinical features of this condition [6, 19]. This condition is most commonly associated with immunoglobulin (Ig) E antibodies to environmental allergens [24]. Frequent characteristics include elevation of eosinophil levels in peripheral blood [9] and increase of staphylococcal colonization in mucosal and skin lesions [4]. Although the pathogenesis of canine AD is complex, involving genetic, environmental and immunological factors, interleukin (IL)-4 and IL-5 produced by T-helper (Th) 2 cells may have especially key roles in its onset and development [23,33]. IL-4 mediates an IgE isotype switch in B cells and IL-5 promotes IgE synthesis induced by IL-4. Mast cells stimulate the release of various chemical mediators in IgE-mediated allergic disorders. Subsequently, many types of inflammatory cells infiltrate into skin lesions [13]. Although Th2 cells are dominant in this acute phase, interferon (IFN)-γ and IL-2 produced by Th1 cells are highly expressed and contribute to the pathogenesis in the chronic phase [16,21]. The above knowledge of AD suggests that down-regulation of the responses of Th1 and Th2 cells may be effective strategies for the management of canine AD.
IL-10, previously known as cytokine synthesis inhibitor factor, has potent anti-inflammatory effects mediated by the inhibition of IFN-γ and IL-2 produced by Th1 cells [5]. It also inhibits IL-4 and IL-5 production by Th2 cells, by interfering with B7/CD28-dependent signals [20,30]. Moreover, eosinophil survival and IL-4-induced IgE synthesis are suppressed by this cytokine [27]. A number of studies have reported that IL-10 can ameliorate various inflammatory disorders including Crohn's disease [34], psoriasis [1] and hepatic ischemia/reperfusion injury [38].
Despite these interesting therapeutic implications for IL-10, the best approaches for delivery of IL-10 in vivo remain to be determined. Most studies have been performed using systemic delivery of the recombinant IL-10 protein. Unfortunately, high dosages of recombinant IL-10 are required for maximal therapeutic activity due to the short half-life (approximately 20 min in plasma) [3]. Such high dosages result in peak plasma IL-10 levels that have potentially undesirable systemic effects. To overcome this limitation, naked plasmid DNA encoding the IL-10 gene has been used in mice; this strategy has produced remarkable biological effects with long duration time (approximately 5 days in plasma) [14]. This long duration time may decrease the need for high systemic protein levels associated with bolus administration of cytokines, thereby reducing undesirable side effects [35].
The present study was performed to examine whether a plasmid DNA carrying the IL-10 gene (IL-10 plasmid DNA) could suppress development of AD-like skin lesions in NC/Nga mice, a suitable model for AD in human and canines [19,32], as an initial step towards the development of an appliance for use in dogs with AD.
Total RNA was extracted from mouse spleen using a RNeasy Mini Kit (Qiagen, USA) and cDNA was synthesized from the extracted RNA using Superscript II reverse transcriptase enzyme (Invitrogen, USA) according to the manufacturer's instructions. Mouse IL-10 gene was amplified from the synthesized cDNA using polymerase chain reaction (PCR) with the following primers: forward primer, 5'-TCC ATC ATG CCT GGC TCA-3' and reverse primer, 5'-GGT GTT TTA GCT TTT CAT TTT-3' (Genebank accession number: NM_010548). The underlined nucleotides imply the start and stop codon, respectively. The amplified 549 bp PCR products were separated by 0.7% (w/v) agarose gel electrophoresis and purified using a QIAquick gel extraction kit (Qiagen, USA) according to the manufacturer's instructions. Purified mouse IL-10 gene was cloned into pGEM-T Easy vector (Promega, USA), and this gene was then sub-cloned into the NotI site of the mammalian expression vector pcDNA3.1 (Invitrogen, USA) to generate pcDNA3.1-IL-10 (named IL-10 plasmid DNA). The construct was transformed into chemically competent Escherichia coli DH5α cells (Invitrogen, USA). The transformants were selected on Luria-Bertani media (BD Biosciences, USA) supplemented with 100 µg/mL ampicillin and the plasmid DNA was extracted using a QIAprep Spin Miniprep Kit (Qiagen, USA) according to the manufacturer's instructions. Restriction analysis (NotI) and DNA sequencing confirmed the presence and orientation of the mouse IL-10 gene in the extracted plasmid DNA. The DNA sequencing reactions were performed using an ABI PRISM 3100 automated DNA sequencer (Applied Biosystems, USA).
To confirm that the constructed IL-10 plasmid DNA was functional and to determine the efficiency of protein expression in mammalian cells, Vero cells (African green monkey kidney cells) were transfected with the IL-10 plasmid DNA using the Nanofect transfection reagent (Qiagen, USA) according to the manufacturer's instructions. Briefly, Vero cells were maintained in wells of a six-well plate in minimum essential medium alpha (α-MEM; Welgene, Korea) supplemented with 1% (v/v) antibiotics (Gibco, USA) and 5% (v/v) fetal bovine serum (Gibco, USA). When 60-70% confluence was reached, the cells were transfected with 2 µg of the IL-10 plasmid DNA pre-treated with Nanofect transfection reagent in α-MEM without serum and antibiotics. Forty-eight hours after transfection, the cells were passaged into maintenance medium with 2% (v/v) geneticin (Gibco, USA). After colonies appeared, surviving cells were selected and passaged into fresh maintenance medium. Forty-eight hours after passage, the cell culture fluids were collected and analyzed for expression of mouse IL-10 protein by the mouse IL-10 Quantikine ELISA Kit (R&D Systems, USA) according to the manufacturer's instructions. Non-transfected vero cell culture fluids were used as negative control, and each sample was tested in triplicate.
Specific pathogen-free (SPF) female 8-week-old NC/Nga mice (SLC, Japan) were randomly divided into two groups consisting of five mice each. All mice were housed in an air-controlled room. Feed and tap water were provided ad libitum for all mice. All animal procedures were conducted in accordance with the guidelines of the local ethical committee (Chonnam National University, Approving number: CNU IACUC-YB-2009-15).
1-chloro-2,4-dinitrobenzene (DNCB; Sigma-Aldrich, USA) was used to induce dermatitis in NC/Nga mice through repeated cutaneous application as previously described [26] with some modification. Briefly, DNCB in an acetone/olive oil mixture (3 : 1) was used. Dorsal skin regions were shaved with an electric razor on day 0 of experiment; this site as well as the glabrous ears were sensitized epicutaneously with 200 µL of a 1% (w/v) DNCB solution on day 4 of the experiment. Three days after sensitization, the dorsal skin and ears were challenged with 150 µL of 0.2% (w/v) DNCB solution every 3 days. In vivo delivery of the IL-10 plasmid DNA was performed as previously described [35]. Briefly, NC/Nga mice were intradermally injected with 100 µg of the IL-10 plasmid DNA in 100 µL of phosphate-buffered saline (PBS) or PBS alone (control) at the base of the tail using a 30-gauge needle and a 1 mL syringe on days 1 and 8 of the experiment. Peripheral blood and skin samples were collected post mortem for analysis 3 days after the third application of 0.2% (w/v) DNCB solution. The experiment schedule is shown in Fig. 1.
The severity of dermatitis was evaluated by scoring clinical signs on the face, ears, nose, neck, and dorsal skin every 3 days starting from day 4 of the experiment. The total clinical skin severity score was defined as the sum of the individual scores (none = 0, mild = 1, moderate = 2, and severe = 3). Skin severity was characterized according to the presence of erythema/hemorrhage, edema, excoriation/erosion, and dryness/scaling as previously described [15].
All mice were sacrificed at the end of the experiment. Sampling was performed from dorsal skin lesions and ears, and the samples were fixed in 10% phosphate-buffered formalin (pH 7.2). Serial paraffin sections 5 µm in thickness were cut and stained with hematoxylin and eosin for evaluating general skin condition and toluidine blue (pH 4.0) for counting mast cells. General skin condition (epidermal hyperplasia, hyperkeratosis, and inflammatory cell infiltration and dermal infiltration of inflammatory cells) was compared between groups as previously described [29]. Mast cell number between the epithelium and panniculus carnosus was counted under a microscope at a magnification of ×400 at five sites chosen at random as previously described [12]. All histological evaluations were performed in blinded fashion.
Blood was collected in tubes coated with ethylene diaminetetraacetic acid. Blood leukocyte and differential counts were determined using an automated veterinary hematology analyzer (Oxford Science, USA) configured for mouse blood.
S. aureus was enumerated as previously described [15] with some modifications. Briefly, an area of ear skin of about 1 cm2 was collected and suspended in 1 mL of sterile PBS. One hundred microliters of 10-fold serial dilutions of the suspensions were inoculated on mannitol salt medium (BD Biosciences, USA) and cultured for 48 h at 37℃. Yellow colonies were counted and expressed as log colony-forming units (cfu)/cm2 (one ear). In addition, representative colonies were identified through gram staining and coagulase test [7].
Blood was collected from the retro-orbital plexus of each mouse at the end of the experiment. Serum was obtained by centrifugation and stored at -20℃ until used. Total serum IgE levels were measured using an OptEIA Mouse IgE ELISA kit (BD Biosciences, USA) and serum IL-10 levels were measured using the Mouse IL-10 Quantikine ELISA Kit (R&D Systems, USA) according to the manufacturer's instructions.
Skin tissues were collected from dorsal lesions of mice. Total RNA extraction was performed using a TRI reagent (MRC, USA) and cDNA was synthesized from the extracted RNA using Superscript II reverse transcriptase enzyme (Invitrogen, USA) according to the manufacturer's instructions. To minimize variations in reverse transcriptase efficiency, all samples were transcribed simultaneously. Primers and probes for murine IL-4, IFN-γ, and β-actin were designed as previously described [25] (Table 1). The probes were dual-labelled with the reporter dye 6-carboxyfluorescein (FAM) at the 5' end and the quencher dye 6-carboxytetramethyrhodamine (TAMRA) at the 3' end. IL-4 and IFN-γ mRNA levels were determined by real-time PCR using a Rotor-gene 6,000 (Corbett Research, Australia) with 0.5 µg of cDNA. The threshold cycle (Ct; the cycle number at which the amount of amplified gene of interest reaches a fixed threshold) was determined subsequently. Relative quantitation of IL-4 and IFN-γ mRNA expression was calculated by a comparative Ct method as previously described [17]. The relative quantitation value of target (IL-4 or IFN-γ) was normalized to an endogenous control β-actin gene and relative to a calibrator. It was expressed as 2-ΔΔCt (fold), where ΔCt = Ct of target gene -Ct of endogenous control gene and ΔΔCt = ΔCt of samples for target gene -ΔCt of the calibrator for the target gene.
The data were expressed as mean ± SD. A paired Student's t-test was performed for statistical analysis of the data. A Mann-Whitney U test (nonparametric test) was used for statistical analysis of the clinical skin severity scores. All statistical analysis of data performed using SPSS version 17.0 software (SPSS, USA). p<0.05 was considered as the level of significance.
The sequence of the mouse IL-10 gene in the constructed IL-10 plasmid DNA was identical with the original mouse IL-10 gene sequence (data not shown). The expression of mouse IL-10 in mammalian cells transfected with the IL-10 plasmid DNA was confirmed by ELISA. The results clearly revealed high expression levels of mouse IL-10 in culture fluid of cells transfected with the IL-10 plasmid DNA (489.9 ± 9.1 pg/mL).
However, the expression levels of mouse IL-10 in non-transfected cells was clearly negative (-0.1 ± 0.1 pg/mL).
Repeated cutaneous application of DNCB accelerates development of dermatitis in NC/Nga mice. Consistent with this finding, clinical skin severity in the control mice injected with PBS alone increased gradually with the number of cutaneous applications of DNCB and reached a peak at the end of experiment (clinical severity score of 8.6 ± 0.6 points). A statistical difference in the clinical skin severity scores was observed between mice injected with the IL-10 plasmid DNA and control mice after the third application of DNCB (Fig. 2). Most control mice exhibited severe symptoms of dermatitis including erythema/hemorrhage, edema, excoriation/erosion, and dryness/scaling, while mice injected with the IL-10 plasmid DNA presented with mild or moderate clinical skin severity (Fig. 3).
Severe epidermal hyperplasia and hyperkeratosis and dense infiltration of inflammatory cells into both the epidermis and dermis were observed in both the dorsal and ear skin of control mice. However, the IL-10 plasmid DNA inhibited these inflammatory changes (Figs. 4A and B). Mice injected with the IL-10 plasmid DNA showed a significant decrease in the epidermal thickness of dorsal skin (p<0.05) and ear skin (p<0.01) compared with control mice (Table 2). Repeated cutaneous application of DNCB increased dermal mast cell number and degranulation in control mice. However, these features were significantly suppressed by the IL-10 plasmid DNA compared with control mice (Figs. 4C and D, Table 2).
The ratio of eosinophils in peripheral leukocytes of mice injected with the IL-10 plasmid DNA was significantly lower than in control mice (p<0.05) (Fig. 5A).
The number of S. aureus (log cfu/cm2) on the ear in mice injected with the IL-10 plasmid DNA showed a significant decrease compared with control mice (p<0.01) (Fig. 5B).
Relative mRNA expression levels of IL-4 and IFN-γ significantly decreased in mice injected with the IL-10 plasmid DNA compared with those in control mice (p<0.05) (Figs. 5E and F).
Direct in vivo transfer of naked plasmid DNA was first described in 1990 as a novel strategy of gene therapy [37]. Initial studies showed muscle to be a suitable target tissue for gene delivery [36,37], but skin was also shown to be suitable as an alternative site for injection [10,35]. In addition, it has been shown that naked plasmid DNA induces local or systemic biological effects in vivo, including recruitment of neutrophils into the site of IL-8 plasmid DNA injection [10] and systemic activation of natural killer cells by IL-12 plasmid DNA injection [35]. In the present study, to confirm that the constructed IL-10 plasmid DNA was functional and to determine the efficiency of protein expression in mammalian cells, mouse IL-10 expression levels were evaluated in both in vitro culture fluids of cells transfected with the IL-10 plasmid DNA and in vivo NC/Nga mice injected with the IL-10 plasmid DNA. Mouse IL-10 levels in culture fluids of transfected cells were much higher than those in non-transfected cells. Moreover, serum IL-10 levels in mice injected with the IL-10 plasmid DNA (51.2 pg/mL) were maintained over twice that in control mice (24.8 pg/mL) at 8 days after two intradermal injections. These results imply that the IL-10 plasmid DNA is functional in mammalian (mouse) cells and has a long duration time in mice. These observations are consistent with a previous report demonstrating that IL-10 plasmid DNA injection induces high and long-lasting levels of serum IL-10 in mice [14].
AD-like skin lesions in NC/Nga mice highly resemble those of human and canine AD [33] and show various grades of clinical features such as erythema and hemorrhage, followed by edematous superficial erosion, deep excoriation, scaling, and dryness of the skin [15]. However, signs of dermatitis vary considerably under conventional conditions and clinical skin disorders do not occur in this strain under SPF conditions [22]. Therefore, to reduce experimental variation and induce stable clinical dermatitis over a short period, investigators have adopted the use of hapten-induced dermatitis using repeated applications of 1-chloro-2,4,6-trinitrobenzene (TNCB); successful development of dermatitis in NC/Nga mice under SPF conditions has been reported [15,22]. Unfortunately for investigations conducted in the Republic of Korea, the importation of TNCB is banned. DNCB was used as a rational alternative in view of its structural similarity and on the basis of previous evidence of its efficacy in creating AD-like skin lesions and IgE hyperproduction in NC/Nga mice [26]. In the present study the control mice also developed other typical AD signs including: erythema, oedema, excoriation, and dryness of the skin lesions; hyperplasia and hyperkeratosis of the epidermis; dense infiltration of inflammatory cells in the dermis; elevation of peripheral blood eosinophil ratio; and increased serum IgE concentration. The observation of increased colonization of the ear skin by S. aureus in the control mice is consistent with the view that massive colonization of S. aureus on the skin coincides with the severity of dermatitis in NC/Nga mice [12,22].
The present study has demonstrated that IL-10 plasmid DNA inhibits development of AD-like skin lesions in NC/Nga mice. Macroscopic and microscopic examinations revealed markedly decreased clinical skin severity and relatively mild epidermal and dermal inflammatory changes in mice injected with the IL-10 plasmid DNA compared with control mice. Moreover, significant decreases of the eosinophil ratio and serum IgE concentration, and a marked reduction in the number of S. aureus colonies recovered from the ear, confirmed the inhibitory effects of the IL-10 plasmid DNA on the development of AD-like skin lesions. Of note, relative mRNA expression levels of IL-4 and IFN-γ in the skin lesions of mice injected with the IL-10 plasmid DNA were decreased compared with those of control mice. These results are consistent with previous reports that IL-10 inhibits IL-4 and IL-5 production by Th2 cells [20,30], and IFN-γ and IL-2 production by Th1 cells [5], as well as eosinophil survival and IL-4-induced IgE synthesis [27].
Other researchers, on the other hand, found that the suppression of IL-10 production by topical application of antisense oligonucleotides for IL-10 (AS6) alleviated the skin lesions of NC/Nga mice [28]. The discrepancy of the effects of IL-10 on AD could be ascribed to the differences in application period (after/before dermatitis-induction), application range (local/systemic effects) and target cell type [skin-infiltrating T cell / IL-10-producing regulatory T cell (Treg)]. As the precise mechanism as to how inhibition of IL-10 production by AS6 promotes skin lesion healing has yet to be clarified, we hypothesize the following mechanism. While IL-10 acts as an immunosuppressive cytokine on most cell types, we know that its effect on B cells is to enhance viability, proliferation and Ig secretion (the isotype switch) [2]. We also know that in dermatitis-induced skin lesions, AS6 suppresses IL-10 produced by skin-infiltrating T cells. Suppression of IL-10 production by AS6, therefore, leads to reduced IgE levels in dermatitis-induced skin lesions, with no effect on the IL-4 level [28]. Conversely, the present study confirmed that the strong induction of IL-10 production by systemic application of IL-10 plasmid DNA inhibits development of AD-like skin lesions in NC/Nga mice. This finding was similar to those of Sawada et al. [29], Segawa et al. [31] and Hayashi et al. [8], i.e. the strong induction of IL-10 in lymphoid organs and systemic levels delays the onset and suppresses the development of AD in NC/Nga mice. Sawada et al. [29] pointed out that the increased IL-10 level could be due to IL-10-producing Treg cells. In the present study, the increased IL-10 level might also be due to IL-10-producing Treg cells induced by IL-10 plasmid DNA. Finally, the mechanism of the beneficial effects of the increased IL-10 level might be the strong immunosuppressive effects of IL-10 on a systemic level, although IL-10 is a pleiotropic cytokine that can exert either immunosuppressive or immunostimulatory effects on a variety of cell types [2].
Taken together, these findings suggest that intradermal injection of IL-10 plasmid DNA can delay the onset and suppress the development of AD-like skin lesions in NC/Nga mice by suppressing the response of both Th1 and Th2 cells. Hence, IL-10 plasmid DNA may be a good candidate for control of AD in dogs. Confirmation of the prophylactic effect of IL-10 plasmid DNA is now required in dogs with AD.
Figures and Tables
Fig. 1
Induction of dermatitis and interleukin (IL)-10 plasmid DNA injection schedule. Dorsal regions of mice were shaved on day 0. The hairless dorsal regions and glabrous ears of mice were sensitized with 200 µL of 1% (w/v) DNCB solution on day 4. Three days after sensitization, the dorsal skin and ears were challenged with 150 µL of 0.2% (w/v) DNCB solution every 3 days. Following in vivo delivery of IL-10 plasmid DNA, mice were intradermally injected with 100 µg of IL-10 plasmid DNA in 100 µL of phosphate-buffered saline (PBS) or PBS alone (control) on days 1 and 8 of experiment.
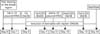
Fig. 2
Clinical skin severity score in the control mice increased gradually with the number of cutaneous applications of DNCB and reached a peak at the end of experiment. A significant improvement in mice injected with IL-10 plasmid DNA had occurred at day 13 (*p<0.05) and by day 16 was even more pronounced (**p<0.01) compared with the control mice (mean ± SD; n = 5).
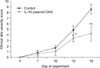
Fig. 3
The skin features demonstrated visually a marked reduction in severity of the dermatitis and more rapid hair re-growth with IL-10 plasmid DNA injection. (A) Dermatitis induced and PBS injected mice, (B) IL-10 plasmid DNA injected mice after dermatitis induction.
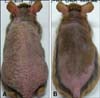
Fig. 4
Histological features of dorsal skin and dermal mast cell infiltration. (A) Hyperplastic epidermis and inflammatory cells were notable. (B) The inflammatory infiltrate was less severe in mice injected with plasmid DNA. H&E stain, Scale bar = 100 µm. (C) More severe infiltration of mast cells was evident in control mice. (D) The mast cell infiltrate was less severe in plasmid DNA injected mice. Toluidine blue stain, Scale bar = 50 µm.
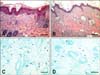
Fig. 5
The inhibitory effects of interleukin-10 plasmid DNA. The concentration of serum IL-10 in mice injected with IL-10 plasmid DNA was significantly higher than in control mice. *p < 0.05 vs. control, **p < 0.01 vs. control.
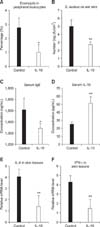
Acknowledgments
The authors acknowledge a graduate fellowship from the Korean Ministry of Education and Human Resources Development through the Brain Korea 21 project. This work was supported by the Regional Technology Innovation Program of the Ministry of Commerce, Industry and Energy (MOCIE), Korea (Grant No. RTI05-01-01) and the CNU Specialization Grant funded by Chonnam National University.
References
1. Asadullah K, Sterry W, Stephanek K, Jasulaitis D, Leupold M, Audring H, Volk HD, Döcke WD. IL-10 is a key cytokine in psoriasis. Proof of principle by IL-10 therapy: a new therapeutic approach. J Clin Invest. 1998. 101:783–794.


2. Barnes PJ, Chung KF, Page CP. Inflammatory mediators of asthma: an update. Pharmacol Rev. 1998. 50:515–596.
3. Chernoff AE, Granowitz EV, Shapiro L, Vannier E, Lonnemann G, Angel JB, Kennedy JS, Rabson AR, Wolff SM, Dinarello CA. A randomized, controlled trial of IL-10 in humans. Inhibition of inflammatory cytokine production and immune responses. J Immunol. 1995. 154:5492–5499.
4. Fazakerley J, Nuttall T, Sales D, Schmidt V, Carter SD, Hart CA, McEwan NA. Staphylococcal colonization of mucosal and lesional skin sites in atopic and healthy dogs. Vet Dermatol. 2009. 20:179–184.


5. Fiorentino DF, Bond MW, Mosmann TR. Two types of mouse T helper cell. IV. Th2 clones secrete a factor that inhibits cytokine production by Th1 clones. J Exp Med. 1989. 170:2081–2095.


6. Griffin CE, DeBoer DJ. The ACVD task force on canine atopic dermatitis (XIV): clinical manifestations of canine atopic dermatitis. Vet Immunol Immunopathol. 2001. 81:255–269.


7. Hashimoto Y, Kaneda Y, Akashi T, Arai I, Nakaike S. Persistence of Staphylococcus aureus colonization on the skin of NC/Nga mice. J Dermatol Sci. 2004. 35:143–150.


8. Hayashi A, Kimura M, Nakamura Y, Yasui H. Anti-atopic dermatitis effects and the mechanism of lactic acid bacteria isolated from Mongolian fermented milk. J Dairy Res. 2009. 76:158–164.


9. Hayashiya S, Tani K, Morimoto M, Hayashi T, Hayasaki M, Nomura T, Une S, Nakaichi M, Taura Y. Expression of T helper 1 and T helper 2 cytokine mRNAs in freshly isolated peripheral blood mononuclear cells from dogs with atopic dermatitis. J Vet Med A Physiol Pathol Clin Med. 2002. 49:27–31.


10. Hengge UR, Chan EF, Foster RA, Walker PS, Vogel JC. Cytokine gene expression in epidermis with biological effects following injection of naked DNA. Nat Genet. 1995. 10:161–166.


11. Hill PB, Lo A, Eden CA, Huntley S, Morey V, Ramsey S, Richardson C, Smith DJ, Sutton C, Taylor MD, Thorpe E, Tidmarsh R, Williams V. Survey of the prevalence, diagnosis and treatment of dermatological conditions in small animals in general practice. Vet Rec. 2006. 158:533–539.


12. Kang JS, Yoon WK, Han MH, Lee H, Lee CW, Lee KH, Han SB, Lee K, Yang KH, Park SK, Kim HM. Inhibition of atopic dermatitis by topical application of silymarin in NC/Nga mice. Int Immunopharmacol. 2008. 8:1475–1480.


13. Kishimoto T, Hirano T. Molecular regulation of B lymphocyte response. Annu Rev Immunol. 1988. 6:485–512.


14. Kokura S, Yoshida N, Ishikawa T, Higashihara H, Sakamoto N, Takagi T, Uchiyama K, Naito Y, Mazda O, Okanoue T, Yoshikawa T. Interleukin-10 plasmid DNA inhibits subcutaneous tumor growth of Colon 26 adenocarcinoma in mice. Cancer Lett. 2005. 218:171–179.


15. Lee HS, Kim SK, Han JB, Choi HM, Park JH, Kim EC, Choi MS, An HJ, Um JY, Kim HM, Min BI. Inhibitory effects of Rumex japonicus Houtt. on the development of atopic dermatitis-like skin lesions in NC/Nga mice. Br J Dermatol. 2006. 155:33–38.


17. Livak KJ, Schmittgen TD. Analysis of relative gene expression data using real-time quantitative PCR and the 2(-Delta Delta C(T)) Method. Methods. 2001. 25:402–408.


18. Lund EM, Armstrong PJ, Kirk CA, Kolar LM, Klausner JS. Health status and population characteristics of dogs and cats examined at private veterinary practices in the United States. J Am Vet Med Assoc. 1999. 214:1336–1341.
20. Moore KW, O'Garra A, de Waal Malefyt R, Vieira P, Mosmann TR. Interleukin-10. Annu Rev Immunol. 1993. 11:165–190.


21. Nuttall TJ, Knight PA, McAleese SM, Lamb JR, Hill PB. Expression of Th1, Th2 and immunosuppressive cytokine gene transcripts in canine atopic dermatitis. Clin Exp Allergy. 2002. 32:789–795.


22. Okada M, Hirasawa Y, Yoshijima K, Imaeda T, Fujimura T, Nagase T, Kimura H, Kyuki K. Effects of clobetasol propionate, a corticosteroid on hapten-induced dermatitis in SPF NC/Nga mice. Pharmacometrics. 2000. 59:135–139.
23. Olivry T, Dean GA, Tompkins MB, Dow JL, Moore PF. Toward a canine model of atopic dermatitis: amplification of cytokine-gene transcripts in the skin of atopic dogs. Exp Dermatol. 1999. 8:204–211.


24. Olivry T, DeBoer DJ, Griffin CE, Halliwell RE, Hill PB, Hillier A, Marsella R, Sousa CA. The ACVD task force on canine atopic dermatitis: forewords and lexicon. Vet Immunol Immunopathol. 2001. 81:143–146.


25. Overbergh L, Valckx D, Waer M, Mathieu C. Quantification of murine cytokine mRNAs using real time quantitative reverse transcriptase PCR. Cytokine. 1999. 11:305–312.


26. Pokharel YR, Lim SC, Kim SC, Heo TH, Choi HK, Kang KW. Sopungyangjae-Tang Inhibits Development of Dermatitis in Nc/Nga Mice. Evid Based Complement Alternat Med. 2008. 5:173–180.


27. Pretolani M, Goldman M. IL-10: a potential therapy for allergic inflammation? Immunol Today. 1997. 18:277–280.


28. Sakamoto T, Miyazaki E, Aramaki Y, Arima H, Takahashi M, Kato Y, Koga M, Tsuchiya S. Improvement of dermatitis by iontophoretically delivered antisense oligonucleotides for interleukin-10 in NC/Nga mice. Gene Ther. 2004. 11:317–324.


29. Sawada J, Morita H, Tanaka A, Salminen S, He F, Matsuda H. Ingestion of heat-treated Lactobacillus rhamnosus GG prevents development of atopic dermatitis in NC/Nga mice. Clin Exp Allergy. 2007. 37:296–303.


30. Schandené L, Alonso-Vega C, Willems F, Gérard C, Delvaux A, Velu T, Devos R, de Boer M, Goldman M. B7/CD28-dependent IL-5 production by human resting T cells is inhibited by IL-10. J Immunol. 1994. 152:4368–4374.
31. Segawa S, Hayashi A, Nakakita Y, Kaneda H, Watari J, Yasui H. Oral administration of heat-killed Lactobacillus brevis SBC8803 ameliorates the development of dermatitis and inhibits immunoglobulin E production in atopic dermatitis model NC/Nga mice. Biol Pharm Bull. 2008. 31:884–889.


32. Shiohara T, Hayakawa J, Mizukawa Y. Animal models for atopic dermatitis: are they relevant to human disease? J Dermatol Sci. 2004. 36:1–9.


33. Sinke JD, Rutten VP, Willemse T. Immune dysregulation in atopic dermatitis. Vet Immunol Immunopathol. 2002. 87:351–356.


34. van Deventer SJ, Elson CO, Fedorak RN. Crohn's Disease Study Group. Multiple doses of intravenous interleukin 10 in steroid-refractory Crohn's disease. Gastroenterology. 1997. 113:383–389.


35. Watanabe M, Fenton RG, Wigginton JM, McCormick KL, Volker KM, Fogler WE, Roessler PG, Wiltrout RH. Intradermal delivery of IL-12 naked DNA induces systemic NK cell activation and Th1 response in vivo that is independent of endogenous IL-12 production. J Immunol. 1999. 163:1943–1950.
36. Wolff JA, Ludtke JJ, Acsadi G, Williams P, Jani A. Long-term persistence of plasmid DNA and foreign gene expression in mouse muscle. Hum Mol Genet. 1992. 1:363–369.


37. Wolff JA, Malone RW, Williams P, Chong W, Acsadi G, Jani A, Felgner PL. Direct gene transfer into mouse muscle in vivo. Science. 1990. 247:1465–1468.