Abstract
Spectral waveform analysis of blood flow velocity in the major arteries of six healthy, conscious immature micropigs was determined using Doppler ultrasonography. Doppler spectral tracings were recorded from the external iliac artery, femoral artery, and renal arcuate artery. Tracings were also taken from three parts of the common carotid artery and two parts of the abdominal aorta. Spectral Doppler parameters included peak systolic velocity, early diastolic velocity, peak systolic velocity-to-end diastolic velocity ratio, resistive index, and pulsatility index. In addition, the diameter of major arteries and indirect blood pressure were measured. These results from spectral Doppler analysis in major arteries may be useful as reference ranges in the future studies of vascular hemodynamics in immature micropigs.
Micropigs are considered as the optimal transplant donor animals because their organs have a similar anatomy and physiology to human organs [5,12]. Therefore, the selection of the normal healthy micropigs and the survey of vessels supplying the organs for transplantation are important. However, there are no baseline studies on the Doppler flow velocity parameters and waveform pattern in the major arteries of the micropigs.
Spectral waveform analysis of blood flow by Doppler ultrasonography is a simple and noninvasive method to investigate blood hemodynamics [1,3,8]. The evaluation of blood flow velocity with spectral waveform analysis by Doppler ultrasonography becomes the most routine and useful technique in many vascular diseases in humans and animals [2-4,6-9,13-15].
The purpose of this study is to determine the baseline blood flow values of the major arteries in immature micropigs using spectral analysis of Doppler ultrasound. For this, we determined the peak systolic velocity (PSV), early diastolic velocity (EDV), peak systolic velocity-to-end diastolic velocity (S:D) ratio, resistive index (RI), and pulsatility index (PI), and evaluated blood flow waveform patterns in the major arteries of normal conscious immature micropigs.
Six healthy micropigs (4-month-old male; PWG Genetics Korea, Korea) were used in this study. Before Doppler ultrasonography, all micropigs were fasted for 24 h to reduce abdominal pressure and ultrasound artifacts from ingesta and feces in their gastrointestinal tracts. Water was supplied ad libitum.
Blood pressure was recorded indirectly using Vet-Dop Doppler (Vmed Technology, USA). Recordings in the left dorsal pedal artery were taken as an average of three time measurements at five-minute intervals under stable and conscious state just prior to the Doppler examination.
Doppler ultrasonography was performed using a 5-12 multifrequency linear-array transducer (SonoAce 9900; Medison, Korea). Each micropig was placed in dorsal or right oblique lateral recumbency under conscious state without anesthesia. The probe was smoothly positioned without compression on the clipped skin over the neck and abdomen to prevent the introduction of artificial errors in vascular resistance. With initial two-dimensional gray-scale images and color Doppler mapping, arteries of interest were identified. Pulsed-wave Doppler examinations were performed on the longitudinal sections of imaged arteries. The size of the sample volume was set at 2 mm and positioned in the center of the vessel by the maximum velocity method using a small sample volume (approximately half of the diameter of the vessel) [6]. The angle of insonation was set at the lowest possible angle under 60 degrees to gain the closest estimation of velocity [1,8,10]. Three distinct pulsed wave spectral tracings containing three consecutive cardiac cycles were recorded.
Spectral Doppler tracings were recorded from the representative waveform in the common carotid artery, abdominal aorta, external iliac artery, femoral artery, and renal arcuate artery. The common carotid artery was imaged along the long axis of the mildly extended neck in dorsal recumbency, and three parts, cranial, middle, and caudal, were evaluated. The cranial part of the common carotid artery was measured caudal to the bifurcation of the internal carotid artery in the cranial deep aspect of the jugular groove. As the caudal part of the common carotid artery, the sample volume was located cranial to the thoracic inlet. The middle part of the common carotid artery was evaluated between the cranial and caudal part of the common carotid artery. The abdominal aorta was evaluated in two parts approximately 2 cm cranial and caudal to the left renal artery in the right oblique lateral recumbency. The left renal arcuate artery, external iliac artery and femoral artery were selected and traced in the dorsal recumbency with the mild right oblique rotation because the left side arteries are easier to approach than the right side for the right handed operator.
Spectral Doppler parameters include PSV, EDV, S:D ratio, RI (peak systolic frequency shift - lowest diastolic frequency shift / peak systolic frequency shift), PI (peak systolic velocity - minimum diastolic velocity / mean velocity), and Doppler waveform pattern. The diameters of the major arteries except the renal arcuate artery were also determined.
The spectral Doppler analysis and the diameter measurements were repeated three times in the same recording sites of each artery under the same scan conditions.
PSVs of the cranial, middle, and caudal parts of the common carotid artery, cranial and caudal parts of the abdominal aorta, external iliac artery, femoral artery, and renal arcuate artery were 97.97 ± 14.04, 107.56 ± 11.97, 97.87 ± 13.58, 95.65 ± 17.62, 107.55 ± 19.45, 85.52 ± 10.01, 93.84 ± 14.35, and 53.92 ± 7.25 cm/sec, respectively (Table 1). The PSV of renal arcuate artery was significantly lower than the other arteries (p < 0.05). The PSV of external iliac artery showed a significantly lower value than the common carotid artery or abdominal aorta (p < 0.05). There were no significant differences observed among the three parts of common carotid artery and the two abdominal aorta parts.
EDVs of the cranial, middle, and caudal parts of the common carotid artery, cranial and caudal parts of the abdominal aorta, external iliac artery, femoral artery, and renal arcuate artery were 25.25 ± 6.97, 28.28 ± 7.43, 21.25 ± 5.36, 20.04 ± 5.66, 22.62 ± 7.53, 27.79 ± 5.72, 18.85 ± 4.03, and 22.57 ± 4.15 cm/sec, respectively (Table 1). The lowest EDV was observed in the femoral artery, which was significantly lower than the external iliac artery (p < 0.05). There were no significant differences observed among the three parts of common carotid artery and the two parts of abdominal aorta.
The S:D ratios of the cranial, middle, and caudal parts of the common carotid artery, cranial and caudal parts of the abdominal aorta, external iliac artery, femoral artery, and renal arcuate artery were 4.14 ± 1.30, 3.99 ± 0.85, 4.92 ± 1.56, 5.61 ± 1.22, 4.50 ± 1.03, 5.79 ± 2.25, 4.77 ± 1.39, and 2.43 ± 0.31, respectively (Table 1). The S:D ratio of renal arcuate artery was significantly lower than the other arteries (p < 0.05). There were no significant differences observed among other measured arteries.
The RIs of the cranial, middle, and caudal parts of the common carotid artery, cranial and caudal parts of the abdominal aorta, external iliac artery, femoral artery, and renal arcuate artery were 0.74 ± 0.06, 0.74 ± 0.05, 0.76 ± 0.07, 0.78 ± 0.06, 0.80 ± 0.05, 0.81 ± 0.03, 0.79 ± 0.03, and 0.58 ± 0.05, respectively (Table 1). The RI of renal arcuate artery was significantly lower than the other arteries (p < 0.05). There were no significant differences observed among other measured arteries.
The PIs of the cranial, middle, and caudal parts of the common carotid artery, cranial and caudal parts of the abdominal aorta, external iliac artery, femoral artery, and renal arcuate artery were 1.47 ± 0.26, 1.51 ± 0.27, 1.71 ± 0.35, 1.95 ± 0.28, 1.77 ± 0.36, 2.06 ± 0.24, 1.82 ± 0.24, and 0.88 ± 0.11, respectively (Table 1). The PI of renal arcuate artery had the lowest result compared to the other measured arteries (p < 0.05). There were no significant differences observed among other measured arteries.
All arteries presented a plug velocity profile and high resistance flow patterns except the renal arcuate artery, which had a parabolic velocity profile and low resistance flow pattern (Fig. 1).
The diameters of the major arteries are shown in Table 2. The size order from largest to smallest was the two parts of the aorta, caudal part of common carotid artery, external iliac artery, cranial and middle parts of common carotid artery, and femoral artery. The renal arcuate artery is so tiny that ultrasound could not measure the diameter.
Systolic blood pressure, diastolic blood pressure, and mean arterial pressure by an indirect blood pressure monitor were 107 ± 9, 62 ± 20, and 74 ± 17 mmHg, respectively.
Doppler ultrasonography and spectral waveform analysis has previously been used to assess both anatomic and dynamic information of blood flow in various vascular diseases [2-4,6,9,13-15].
This is due to each vessel having its specific Doppler signature, and the physiological and pathological changes of the vessels can be recognized by the evaluation of the Doppler spectrum [3,10,14]. Pulsed wave Doppler is used to identify vessel and assess the direction, velocity and pattern of blood flow. The common indices include PSV, EDV, S:D ratio, RI, and PI. In particular, the knowledge of PSV threshold is the most useful parameter for vascular stenotic diseases [2,4,11,14,15].
During ultrasonographic examination of the common carotid artery, it was necessary to extend the neck because of its short length and the large volume of fat in the micropig neck scanning region, although there would be mild artificial changes of blood flow spectrum. From the caudal to the cranial part, the common carotid artery of pigs separate into the right and left artery near the thoracic inlet, ascend along the medial aspect of internal jugular vein, and terminates into the internal carotid, lingual, and external carotid arteries in the cranial deep aspect of the jugular groove [12]. However, there were no significant differences observed among the three parts of common carotid artery in all blood flow velocity parameters and waveform pattern.
To scan the abdominal aorta, the transducer was placed on the caudodorsal part of the abdomen in the right oblique lateral recumbency. Micropigs had intestines filled with gas and ingesta in spite of fasting because of their unique, coiling intestinal structures [12]. Therefore, the renal artery was used as an only reference point to separate into the cranial and caudal parts of the abdominal aorta. The two parts of the abdominal aorta showed no significant differences in all blood flow velocity parameters and waveform pattern (p > 0.05).
The external iliac artery arises from the abdominal aorta, ventral to the last lumbar vertebra. It passes caudolaterally along the medial face of the iliopsoas muscle and courses along the deep face of the sartorius muscle ventrocaudally through the femoral ring [12]. This external iliac artery continues to the femoral artery, which crosses the medial surface of the femur [12]. The external iliac and femoral arteries were easy to trace on B-mode ultrasound. However, the Doppler angle needed to be maintained at almost 60 degrees because of the superficial location of both arteries. The femoral artery was evaluated in the region caudal to the femoral ring to minimize the Doppler angle. The lowest PSV was recorded in the external iliac artery and the lowest EDV was observed in the femoral artery compared to other arteries measured except the renal arcuate artery in this study.
All major arteries, including common carotid, abdominal aorta, external iliac, and femoral, showed plug flow velocity profiles with a narrow range of frequencies/velocities. These waveforms had high pulsatility and high resistance flow patterns with one sharp systolic peak, large and clear spectral windows, and a narrow velocity distribution. These results are similar to previous human and other animal studies [2,7,8,10] because all measured arteries are major large arteries.
The PSV, S:D ratio, RI, and PI of the left renal arcuate artery showed the lowest values compared to other arteries in this study (p < 0.05). And the renal arcuate artery showed a parabolic velocity profile with a wide range of frequencies/velocities, low pulsatility, low-resistance flow with broad and continuous systolic peaks, a gradually decreasing velocity, and continuous high velocity flow in diastole as previous study [1,3,8]. This waveform is found in arteries of the solid organs, in particular, the kidneys, testes and prostate. The low resistance waveform has a characteristic of continuing high flow during diastole, which is 20-50 percent of the peak systolic velocity [3]. The ratio of systolic to diastolic flow may be useful measurable parameter in this waveform. In this study, the diastolic velocity of renal arcuate artery was about 41 percent of the peak systolic velocity and showed the lowest S:D ratio.
This study investigated blood velocity parameters of major arteries in the normal conscious immature micropigs using Doppler ultrasonography. The number of micropigs is a study limitation and further study is necessary to compare these data with another age group or other species. However, the results of this study may be useful as a baseline data for future investigations, including vascular disease models and transplantation of organs.
Figures and Tables
Fig. 1
Representative pulsed wave Doppler spectral waveform pattern for the common carotid artery (A), abdominal aorta (B), external iliac artery (C), femoral artery (D), and renal arcuate artery (E). The scale to the right of the image is in increments of 10 cm/sec.
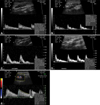
Acknowledgments
This work was supported by a grant (2007 0401034006) from the BioGreen 21 Program, Rural Development Administration, Korea.
References
1. Allan PL, Dubbins PA, Pozniak MA, McDicken WN. Clinical Doppler Ultrasound. 2006. 2nd ed. Philadelphia: Elsevier;27–39.
2. Blohmé L, Pagani M, Parra-Hoyos H, Olofsson P, Takolander R, Swedenborg J. Changes in middle cerebral artery flow velocity and pulsatility index after carotid endarterectomy. Eur J Vasc Surg. 1991. 5:659–663.


3. Cochlin DL, Dubbins PA, Goldberg BB, Alexander AA. Cochlin DL, Dubbins PA, Goldberg BB, Halpern FJ, editors. Basic principle of Doppler. Urogenital Ultrasound: A Text Atlas. 1994. 2nd ed. London: Informa Healthcare;1–22.
4. Cooperberg E. Ultrasound Doppler spectral analysis in the diagnosis of occlusive lesions of the carotid arteries. Ultrasound Med Biol. 1992. 18:421–425.


5. Dooldeniya MD, Warrens AN. Xenotransplantation: where are we today? J R Soc Med. 2003. 96:111–117.


6. Finn-Bodner ST, Hudson JA. Abdominal vascular sonography. Vet Clin North Am Small Anim Pract. 1998. 28:887–942.


7. Fraser KH, Meagher S, Blake JR, Easson WJ, Hoskins PR. Characterization of an abdominal aortic velocity waveform in patients with abdominal aortic aneurysm. Ultrasound Med Biol. 2008. 34:73–80.


8. Kremkau FW. Diagnostic Ultrasound: Principles and Instruments. 2006. 7th ed. St. Louis: Saunders;217–257.
9. Lee K, Choi M, Yoon J, Jung J. Spectral waveform analysis of major arteries in conscious dogs by doppler ultrasonography. Vet Radiol Ultrasound. 2004. 45:166–171.


10. Nelson TR, Pretorius DH. The Doppler signal: where does it come from and what does it mean? AJR Am J Roentgenol. 1988. 151:439–447.


11. Robinson ML, Sacks D, Perlmutter GS, Marinelli DL. Diagnostic criteria for carotid duplex sonography. AJR Am J Roentgenol. 1988. 151:1045–1049.


12. Sisson S, Grossman JD, Getty R. Sisson and Grossman's the Anatomy of the Domestic Animals. 1975. 5th ed. Philadelphia: Saunders;1306–1342.
13. Spaulding KA. A review of sonographic identification of abdominal blood vessels and juxtavascular organs. Vet Radiol Ultrasound. 1997. 38:4–23.


14. Taylor KJW, Burns PN, Woodcock JP, Wells PNT. Blood flow in deep abdominal and pelvic vessels: ultrasonic pulsed-Doppler analysis. Radiology. 1985. 154:487–493.


15. Zwiebel WJ, Fruechte D. Basics of abdominal and pelvic duplex: instrumentation, anatomy, and vascular Doppler signatures. Semin Ultrasound CT MR. 1992. 13:3–21.