Abstract
Six 1-month-old piglets were intravenously injected with deoxynivalenol (DON) at the concentration of 1 mg/kg body weight, with three pigs each necropsied at 6 and 24 h post-injection (PI) for investigation of hepatotoxicity and immunotoxicity with special attention to apoptotic changes and cytokine mRNA expression. Histopathological examination of the DON-injected pigs revealed systemic apoptosis of lymphocytes in lymphoid tissues and hepatocytes. Apoptosis of lymphocytes and hepatocytes was confirmed by the TdT-mediated dUTP-biotin nick end-labeling (TUNEL) method and immunohistochemical staining against single-stranded DNA and cleaved caspase-3. The number of TUNEL-positive cells in the thymus and Peyer's patches of the ileum was increased at 24 h PI compared to 6 h PI, but the peak was at 6 h PI in the liver. The mRNA expression of interleukin (IL)-1β, IL-6, IL-18, and tumor necrosis factor (TNF)-α in the spleen, thymus and mesenteric lymph nodes were determined by semi-quantitative RT-PCR, and elevated expression of IL-1β mRNA at 6 h PI and a decrease of IL-18 mRNA at 24 h PI were observed in the spleen. IL-1β and IL-6 mRNA expressions increased significantly at 6 h PI in the thymus, but TNF-α decreased at 6 h PI in the mesenteric lymph nodes. These results show the apoptosis of hepatocytes suggesting the hepatotoxic potential of DON, in addition to an immunotoxic effect on the modulation of proinflammatory cytokine genes in lymphoid organs with extensive apoptosis of lymphocytes induced by acute exposure to DON in pigs.
Deoxynivalenol (DON) is a mycotoxin produced by Fusarium spp. and a major contaminant of wheat and other grains in many countries around the world. Pigs are the animal most sensitive to DON, followed by rodents, dogs, cats, poultry, and ruminants [24,30,35]. Animals fed low to moderate doses of DON show a reduction in feed consumption and lower weight gain; higher doses induce vomiting, increased salivation, and malaise [4,24,27,30,35,36].
DON cytotoxicity is mainly caused by its inhibition of protein synthesis via binding to the ribosome [24,30]. Leukocytes are central targets of DON, and high-dose DON exposure promotes leukocyte apoptosis [20], which were confirmed in mouse and in vitrostudies [22,33,37]. The hepatotoxic effect of DON was reported in cell culture experiments with primary hepatocytes of rats [5], pigs [18] and humans [15], but apoptosis of hepatocytes induced by DON has not been reported in animal experiments. In addition, in vivo pig studies on the hepatotoxic potential of DON using naturally contaminated feedstuffs have produced inconsistent results [1,6,9,11,13,31,34]. Therefore, pure DON administration experiments are needed for direct demonstration of the hepatotoxic effect of DON, as naturally contaminated feedstuffs can contain several kinds of mycotoxins other than DON [1,9,13,34]. It was also reported that the effect of a pure DON-spiked diet on pigs was milder than naturally contaminated feed containing the same level of DON, suggesting the presence of additional known and/or unidentified toxic compounds in naturally contaminated grain [27].
Acute trichothecene poisoning in humans and animals has been characterized as a multisystem shock-like syndrome, with clinical signs that include dermal irritation, nausea, vomiting, diarrhea, hemorrhage, and hematological lesions such as leukopenia and anemia [30], some of which could be mediated by cytokines. Trichothecene exposure affects the immune function by initiating a rapid and transient upregulation of proinflammatory cytokines including interleukin (IL)-1β, IL-6, tumor necrosis factor (TNF)-α, interferon (IFN)-γ and IL-2 in mice [3,38,39]. Cytokines play a critical role in the regulation of immune responses; however, information about cytokine modulation by DON in pigs is very limited and has only been reported after prolonged exposure to DON [25].
The aims of this study were: 1. To demonstrate in vivo hepatotoxicity of DON in pigs, compared to immunotoxicity, with special attention to apoptosis. 2. To evaluate the effect of DON on the modulation of cytokine genes in lymphoid organs of acutely exposed pigs.
Nine healthy, 1-month-old LWD (triple crossbreed of Landrace, Large White and Duroc) piglets (8~10 kg) from an SPF farm (Chiba Prefecture, Japan) were used for the experiments. All pigs were fed the same commercial swine feed. Six pigs were intravenously injected with DON (Wako, Japan) into the jugular vein at the concentration of 1 mg/kg body weight in 0.9% physiological saline (total volume 10 mL), which corresponds to the concentration that previously induced apoptosis of hepatocytes in vitro [18]. Three control pigs received an equivalent amount of saline. Three DON pigs were necropsied at 6 h post-injection (PI) and the remaining 3 DON pigs and 3 control pigs at 24 h PI after euthanization (exsanguinated under anesthesia). A complete necropsy was performed, and collected tissue samples were fixed in 10% phosphate-buffered formalin. The tissues were routinely embedded in paraffin, and 3~4 µm thick sections stained with hematoxylin and eosin (H&E) were prepared for histopathological examination.
All animal experiments were carried out under the approval of the Animal Ethics Committee of the National Institute of Animal Health.
TdT-mediated dUTP-biotin nick end-labeling (TUNEL) method was performed on the liver, spleen, mesenteric lymph node, thymus, and ileum using a commercial kit for TUNEL (ApopTag Kit; Chemicon, USA) according to the manufacturer's instructions.
For immunohistochemical examination, sections of the liver, spleen, mesenteric lymph node, thymus, and ileum were deparaffinized, and endogenous peroxidase activity was blocked by 3% H2O2 in methanol at room temperature for 30 min. Proteolytic digestion for single-stranded DNA (ssDNA) detection was performed by 0.1% actinase E (Kaken Pharmaceutical, Japan)-PBS at 37℃ for 10 min. For detection of cleaved caspase-3, sections were autoclaved at 120℃ for 10 min in 1 mM EDTA solution (pH 8.0) [2]. Sections were incubated with anti-ssDNA antibody (Dako Japan, Japan) diluted 1 : 200 or with anti-cleaved caspase-3 antibody (Cell Signaling Technology, USA) diluted 1 : 100 at 4℃ overnight. Next, all sections were incubated with Histofine Simple Stain MAX-PO(R) (Nichirei, Japan) at room temperature for 30 min, using diaminobenzidine as the chromogen for 5 min, then counterstained with hematoxylin.
For the morphometrical analysis, the total number of TUNEL-positive cells in the thymus (cortex), ileum (Peyer's patches), and liver were counted in 5 fields each section displayed randomly on the monitor at high-power view. The mean values and standard deviations were calculated from three pigs each in the DON-6 h, DON-24 h and control groups, and assessed for statistical difference by Dunnett's method.
Small pieces of tissue samples were collected from the spleen, thymus and mesenteric lymph nodes at the necropsy and stored in RNAlater (Ambion, USA) at -20℃. Total RNA from the samples was isolated using TRIzol Reagent (Invitrogen, USA) according to the manufacturer's manual. Extracted RNA was resuspended in 20 µL ultra-pure water. The purity and concentration were checked by a spectrophotometer at 260 and 280 nm. Expressions of IL-1β, IL-6, IL-18 and TNF-α mRNA were determined by semi-quantitative RT-PCR as described previously [17]. Briefly, 1 µg of RNA isolated from each organ was reverse transcribed using oligo (dT)15 primers (Roche Diagnostics, Germany) and an RNA PCR Kit (AMV) Ver.2.1 (Takara, Japan), essentially according to the manufacturer's protocol. The cDNA equivalent to 25 ng total RNA was subjected to PCR for IL-1β, IL-6, IL-18, TNF-α and β-actin. Electrophoresis band intensities of the PCR products were quantified using Light Capture type AE-6962 and a CS Analyzer (Atto, Japan). Mean IL-1β, IL-6, IL-18 and TNF-α mRNA expression levels normalized against β-actin levels from each tissue were presented in arbitrary units. Mean values and standard deviations were calculated from three pigs each in the DON- 6h PI, DON-24h PI and control groups, and analyzed for statistical difference by Dunnett's method.
All of the DON-injected pigs showed hypersalivation from approximately 5 min PI, vomiting from 10 min PI, diarrhea from around 1 h PI, and the pigs were depressed and anorectic. The salivation, vomiting, and diarrhea disappeared at around 3 h PI, but depression and anorexia continued. The pigs appeared to have recovered from clinical symptoms of the disease at 24 h PI. No clinical signs were observed in control pigs injected with saline. The common necropsy findings in DON-injected pigs (6 and 24 h PI) were mucosal hemorrhage from the fundus to the pylorus of the stomach. No significant lesions were seen in control pigs.
Upon histopathological examination, common findings in DON-injected pigs were systemic apoptosis of lymphocytes in lymphoid tissues including the spleen (data not shown), tonsil (data not shown), lymph nodes (data not shown), thymus, and Peyer's patches of the ileum. Fig. 1 shows the microscopic lesions of thymus and Peyer's patches. Apoptotic lymphocytes had chromatin-condensed nuclei, often with chromatin margination to the nuclear membrane. Apoptotic changes of lymphocytes were observed at both 6 and 24 h PI, but were more severe at 24 h PI (Fig. 1). Increased apoptosis was also seen in the bone marrow and infiltrated/resident lymphocytes in the epithelia, lamina propria, and submucosa of the trachea and gastrointestinal tract (data not shown).
In the liver, activation of Kupffer cells and dilation of sinusoids were observed, together with apoptotic hepatocytes exfoliated from hepatic cords, apoptotic bodies of hepatocytes (acidophilic bodies), and acidophilic material in the sinusoids in DON-injected pigs (Fig. 1). These lesions were conspicuous at 6 h PI, but were mild at 24 h PI. In addition to these findings, periacinar degeneration to necrosis was occasionally observed in the liver at 6 h (1/3) and 24 h PI (2/3) in DON-injected pigs. The most commonly observed stomach lesions in DON-injected pigs were hemorrhage and necrosis of the mucous membrane, principally in the fundic to pyloric area. There were no additional findings related to DON administration.
The TUNEL method and immunohistochemical staining against ssDNA and caspase-3 revealed apoptosis of lymphocytes in the spleen, mesenteric lymph nodes, thymus and ileum, and hepatocytes in the liver. A positive reaction was seen in the nuclei of apoptotic cells with the TUNEL method and anti-ssDNA immunostaining, but in the cytoplasm with anti-caspase-3 immunostaining (Fig. 2). There were significantly greater numbers of TUNEL-positive cells in the lymphoid tissues and the liver of DON-injected pigs compared to the controls (Fig. 3). The number of TUNEL-positive cells in the thymus and Peyer's patches of the ileum increased further at 24 h PI compared to those at 6 h PI (Figs. 3A and B). On the other hand, the number of TUNEL-positive cells peaked in the liver at 6 h PI (Fig. 3C), consistent with histopathological observations.
A statistically significant increase of IL-1β mRNA at 6 h PI and decrease of IL-18 mRNA at 24 h PI were observed in the spleen by RT-PCR (Fig. 4A). Increased expression of IL-6 at 6 h PI was also observed without significant difference. The mRNA expression for IL-1β and IL-6 increased significantly at 6 h PI in the thymus. A decrease of TNF-α mRNA was observed at 6 h PI in the mesenteric lymph nodes. These changes were all transient.
Until now, DON-induced apoptosis of hepatocytes has only been reported in vitro [15,18]. Here, this phenomenon was confirmed to exist in vivo as well. Although distortion of hepatic cords with degenerative to necrotic changes of individual hepatocytes were observed at 3 and 24 h following intraperitoneal administration of DON (10 mg/kg body weight) in rats [31], those findings could be related to apoptosis. The number of TUNEL-positive cells in piglet livers peaked at 6 h PI and was reduced at 24 h PI. This is in agreement with the study of Ihara et al. [14], which showed that hepatocyte apoptosis in mice induced by T-2 toxin, a trichothecene mycotoxin, was observed at 2 h PI, but not at 12 h PI. These findings may reflect the fact that Kupffer cells in the liver swiftly and efficiently remove apoptotic cells [10].
Immunohistochemical staining using anti-cleaved caspase-3 antibody is an easy and reliable method for the detection and qualification of apoptotic cells [28]. DON-induced apoptosis in porcine hepatocytes was demonstrated to occur through the caspase-3 activation pathway [18]. Our immunohistochemical results support that finding in the liver of the DON-injected pig as well. It is speculated that p38 and ERK act as molecular rheostats in DON-induced apoptosis, that activation of both ERK and p38 leads to the expression of immune genes and antiapoptotic pathways, and that only p38 activation initiates the apoptotic response involving caspase-3 activation [20].
It was reported that DON concentrations in liver tissue after intravenous administration (1 mg/kg body weight) in pigs was 1,114.3 ± 153.9 ng/g tissue at 0.33 h PI [26]. Although we did not determine the concentration of DON in the organs, these observation suggested the DON concentration of the liver may have reached the same level as in our previous in vitro study, in which we observed apoptosis of hepatocytes macroscopically at the concentration of 1 µg/mL [18].
Induction of lymphocytic apoptosis by DON has been previously reported in mice [22,37], but has not been clearly demonstrated in pigs. In this study, we confirmed that DON induced apoptosis of lymphocytes in pigs. Coppock et al. [7] reported mild lympholysis of the mesenteric lymph nodes in intravenously DON-injected (0.5 mg/kg) pigs, and lymphocytic depletion and atrophy of lymphoid organs were reported in long-term swine feeding experiments [6-8]. These findings are considered to be the consequence of lymphocyte apoptosis. It was suggested that DON can suppress host resistance to Listeria [21], Salmonella [32] and enteric reovirus [16] in mice. The same predisposition may be also a possible outcome in pigs exposed to a high level of DON because extensive apoptosis of lymphocytes is considered to be a manifestation of immunosuppression [20,23,24]. More detailed study is needed to elucidate the impact of DON on the porcine immune system, as DON can be either immunostimulatory or immunosuppressive in mice, depending on the dose, exposure frequency, and timing of functional immune assays [20,23,24].
We also found hemorrhagic lesions in the fundic area of the stomach in DON-injected pigs. "Hemorrhagic gastritis" is often seen in pigs with acute septicemia, and the severe congestion of mucous membranes in this condition is attributed to venous infarction from endotoxemia [12]. This type of gastric change is also observed with certain intoxicants. Our findings could be associated with this condition, although venous thrombi were not conspicuous in H&E stain section of DON-injected pigs.
The transient elevation of IL-1β and IL-6 mRNA at 6 h PI was in accordance with the previous mice studies [3,39]. It was reported that mRNA levels for IL-1β, IL-6, TNF-α, IFN-γ and IL-2 increased rapidly in spleens of mice exposed orally to 5 or 25 mg/kg body weight DON. No elevation of TNF-α mRNA expression was observed in the spleen of DON-inoculated pigs in our study. This result probably depends on the sampling time, as Zhou et al. [39] detected TNF-α mRNA elevation at 3 h PI in mice. Since TNF-α is the most acutely responsive cytokine, the peak of this cytokine concentration in the serum was at 1 h PI in lipopolysaccharide (LPS)-inoculated pigs [19]. Our preliminary data indicates the elevation of TNF-α mRNA up to 2 times greater than control at 3 h PI (data not shown). Elevated cytokine mRNA returned to the normal levels after 24 h PI in our study, in agreement with the results from DON-exposed mice, showing that the maximum duration for the elevation of cytokine mRNA was 4 to 8 h [39]. In another experiment, mice were orally exposed to 12.5 mg/kg body weight DON and splenic TNF-α, IL-1β and IL-6 mRNA were found to peak at 3 h PI and remained significantly elevated at 6 h PI, but not at 9 h PI [38]. The mRNA expression of IFN-γ, IL-4, IL-6 and transforming growth factor-β were not affected in the spleen and ileum of pigs fed a naturally DON-contaminated diet (2.2-2.5 mg/kg) for 9 weeks [25]. The differences from our results are considered to be due to the exposure dose and duration. A high dose of DON induce "shock-like death" in mice; our results suggest that the "cytokine storm" which was observed in LPS-inoculated pigs [19] may be induced in acutely DON-exposed pigs as well.
Cytokine mRNA expression varied according to the lymphoid organs [17]. The decrease of IL-18 mRNA at 24 h PI and the decrease of TNF-α at 6 h PI were observed only in the spleen and mesenteric lymph nodes, respectively. We did not determine serum cytokine levels in this study, but mRNA expression in the spleen may reflect a systemic reaction, as the expression of cytokine mRNA in the spleen has been reported to correlate well with the cytokine concentrations in the serum [39].
The use of pigs for immunotoxicological studies is a chance to extend the knowledge gained from rodent animal models [29]. One problem in our experiment was the rather high inter-individual variation of mRNA expression of cytokines as observed by RT-PCR. An experimental design using line-bred miniature pigs may eliminate the influence of inter-individual variation.
In this study, we showed the apoptosis of hepatocytes in vivo, indicating the hepatotoxic potential of DON in pigs, as well as its immunotoxic effect on the modulation of proinflammatory cytokine genes in lymphoid organs with extensive apoptosis of lymphocytes induced by acute exposure to DON.
Figures and Tables
Fig. 1
Microscopic lesions of thymus, Peyer's patches of ileum and liver. Increased numbers of apoptotic lymphocytes (arrows) are observed in the thymus and Peyer's patches of deoxynivalenol (DON) -injected pigs at 24 h PI. Apoptotic changes of hepatocytes (arrow heads) and activation of Kupffer cells are found in the liver of DON-injected pigs, especially at 6 h PI. (Inset: high-power view of apoptotic hepatocyte, Scale bar = 10 µm.) Thy: thymus, PP: Peyer's patches of ileum, Li: liver. H&E stain, Scale bars = 20 µm.
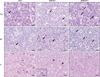
Fig. 2
TdT-mediated dUTP-biotin nick end-labeling (TUNEL) (A) and immunohistochemical staining against single stranded DNA (ssDNA) (B) and cleaved caspase-3 (C) for thymus and liver of DON-injected (6 h PI) and control pigs. Increased number of positive reactions are observed in DON-injected pigs. Positive reactions are in the nuclei of apoptotic cells in TUNEL and anti-ssDNA staining, but in the cytoplasm in anti-cleaved caspase-3 staining. C: control, D: DON-injected pig. Scale bars = 20 µm.
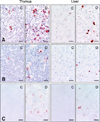
Fig. 3
Number of TUNEL-positive cells in thymus (A), Peyer's patches (B), and liver (C) of DON-injected and control pigs. TUNEL-positive cells in the thymus and Peyer's patches reach a maximum at 24 h PI (A, B), while the peak in the liver is at 6 h PI (C). Data are shown as mean ± SD from three pigs. *Significant difference compared with controls (p ≤ 0.05).
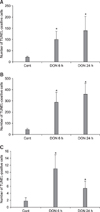
Fig. 4
Expression of proinflammatory cytokine mRNA in the lymphoid organs from DON-injected and control pigs. (A) spleen; (B) thymus; (C) mesenteric lymph nodes. Bars show the mean expression of mRNA of three pigs from each group as the ratio of the band intensity of each PCR product to the corresponding β-actin PCR product. Error bars show standard deviation. *Significant difference compared with control (p ≤ 0.05).
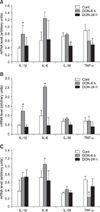
Acknowledgments
The authors would like to thank Mr. M. Kobayashi, Ms. M. Shimada, and Mr. T. Ohashi for their technical assistance. This work was supported by a grant from the Ministry of Agriculture, Forestry and Fisheries of Japan (Integrated Research Program for Functionality and Safety of Food toward an Establishment of Healthy Diet).
References
1. Accensi F, Pinton P, Callu P, Abella-Bourges N, Guelfi JF, Grosjean F, Oswald IP. Ingestion of low doses of deoxynivalenol does not affect hematological, biochemical, or immune responses of piglets. J Anim Sci. 2006. 84:1935–1942.


2. Gown AM, Willingham MC. Improved detection of apoptotic cells in archival paraffin sections: immunohistochemistry using antibodies to cleaved caspase 3. J Histochem Cytochem. 2002. 50:449–454.


3. Azcona-Olivera JI, Ouyang Y, Murtha J, Chu FS, Pestka JJ. Induction of cytokine mRNAs in mice after oral exposure to the trichothecene vomitoxin (deoxynivalenol): relationship to toxin distribution and protein synthesis inhibition. Toxicol Appl Pharmacol. 1995. 133:109–120.


4. Bergsjø B, Langseth W, Nafstad I, Jansen JH, Larsen HJ. The effects of naturally deoxynivalenol-contaminated oats on the clinical condition, blood parameters, performance and carcass composition of growing pigs. Vet Res Commun. 1993. 17:283–294.


5. Bradlaw JA, Swentzel KC, Alterman E, Hauswirth JW. Evaluation of purified 4-deoxynivalenol (vomitoxin) for unscheduled DNA synthesis in the primary rat hepatocyte-DNA repair assay. Food Chem Toxicol. 1985. 23:1063–1067.


6. Chen F, Ma Y, Xue C, Ma J, Xie Q, Wang G, Bi Y, Cao Y. The combination of deoxynivalenol and zearalenone at permitted feed concentrations causes serious physiological effects in young pigs. J Vet Sci. 2008. 9:39–44.


7. Coppock RW, Swanson SP, Gelberg HB, Koritz GD, Hoffman WE, Buck WB, Vesonder RF. Preliminary study of the pharmacokinetics and toxicopathy of deoxynivalenol (vomitoxin) in swine. Am J Vet Res. 1985. 46:169–174.
8. Cote LM, Beasley VR, Bratich PM, Swanson SP, Shivaprasad HL, Buck WB. Sex-related reduced weight gains in growing swine fed diets containing deoxynivalenol. J Anim Sci. 1985. 61:942–950.


9. Dänicke S, Valenta H, Klobasa F, Döll S, Ganter M, Flachowsky G. Effects of graded levels of Fusarium toxin contaminated wheat in diets for fattening pigs on growth performance, nutrient digestibility, deoxynivalenol balance and clinical serum characteristics. Arch Anim Nutr. 2004. 58:1–17.


10. Dini L, Pagliara P, Carlà EC. Phagocytosis of apoptotic cells by liver: a morphological study. Microsc Res Tech. 2002. 57:530–540.


11. Drochner W, Schollenberger M, Götz S, Lauber U, Tafaj M, Piepho HP. Subacute effects of moderate feed loads of isolated Fusarium toxin deoxynivalenol on selected parameters of metabolism in weaned growing piglets. J Anim Physiol Anim Nutr (Berl). 2006. 90:421–428.


12. Gelberg HB. McGavin MD, Zachary JF, editors. Alimentary system. Pathologic Basis of Veterinary Disease. 2007. 4th ed. Elsevier: St. Louis;301–391.
13. Goyarts T, Grove N, Dänicke S. Effects of the Fusarium toxin deoxynivalenol from naturally contaminated wheat given subchronically or as one single dose on the in vivo protein synthesis of peripheral blood lymphocytes and plasma proteins in the pig. Food Chem Toxicol. 2006. 44:1953–1965.


14. Ihara T, Sugamata M, Sekijima M, Okumura H, Yoshino N, Ueno Y. Apoptotic cellular damage in mice after T-2 toxin-induced acute toxicosis. Nat Toxins. 1997. 5:141–145.


15. Königs M, Schwerdt G, Gekle M, Humpf HU. Effects of the mycotoxin deoxynivalenol on human primary hepatocytes. Mol Nutr Food Res. 2008. 52:830–839.


16. Li M, Cuff CF, Pestka J. Modulation of murine host response to enteric reovirus infection by the trichothecene deoxynivalenol. Toxicol Sci. 2005. 87:134–145.


17. Mikami O, Muneta Y, Mori Y, Yokomizo Y, Nakajima Y. Expression of proinflammatory cytokine mRNA in the lymphatic organs of adult and neonatal pigs. Vet Immunol Immunopathol. 2002. 90:203–207.


18. Mikami O, Yamamoto S, Yamanaka N, Nakajima Y. Porcine hepatocyte apoptosis and reduction of albumin secretion induced by deoxynivalenol. Toxicology. 2004. 204:241–249.


19. Nakajima Y, Momotani E, Takahashi H, Ishikawa Y, Ito T, Kanesaki M, Madarame H. Endogenous tumor necrosis factor (TNF) production and modification of pathological lesions in experimental Escherichia coli endotoxemia of piglets. Vet Immunol Immunopathol. 1995. 45:45–54.


20. Pestka JJ. Mechanisms of deoxynivalenol-induced gene expression and apoptosis. Food Addit Contam Part A Chem Anal Control Expo Risk Assess. 2008. 25:1128–1140.


21. Pestka JJ, Tai JH, Witt MF, Dixon DE, Forsell JH. Suppression of immune response in the B6C3F1 mouse after dietary exposure to the Fusarium mycotoxins deoxynivalenol (vomitoxin) and zearalenone. Food Chem Toxicol. 1987. 25:297–304.


22. Pestka JJ, Yan D, King LE. Flow cytometric analysis of the effects of in vitro exposure to vomitoxin (deoxynivalenol) on apoptosis in murine T, B and IgA+ cells. Food Chem Toxicol. 1994. 32:1125–1136.


23. Pestka JJ, Zhou HR, Moon Y, Chung YJ. Cellular and molecular mechanisms for immune modulation by deoxynivalenol and other trichothecenes: unraveling a paradox. Toxicol Lett. 2004. 153:61–73.


24. Pestka JJ, Smolinski AT. Deoxynivalenol: toxicology and potential effects on humans. J Toxicol Environ Health B Crit Rev. 2005. 8:39–69.


25. Pinton P, Accensi F, Beauchamp E, Cossalter AM, Callu P, Grosjean F, Oswald IP. Ingestion of deoxynivalenol (DON) contaminated feed alters the pig vaccinal immune responses. Toxicol Lett. 2008. 177:215–222.


26. Prelusky DB, Trenholm HL. Tissue distribution of deoxynivalenol in swine dosed intravenously. J Agric Food Chem. 1991. 39:748–751.


27. Prelusky DB, Gerdes RG, Underhill KL, Rotter BA, Jui PY, Trenholm HL. Effects of low-level dietary deoxynivalenol on haematological and clinical parameters of the pig. Nat Toxins. 1994. 2:97–104.


28. Resendes AR, Majó N, Segalés J, Espadamala J, Mateu E, Chianini F, Nofrarías M, Domingo M. Apoptosis in normal lymphoid organs from healthy normal, conventional pigs at different ages detected by TUNEL and cleaved caspase-3 immunohistochemistry in paraffin-embedded tissues. Vet Immunol Immunopathol. 2004. 99:203–213.


29. Rothkötter HJ, Sowa E, Pabst R. The pig as a model of developmental immunology. Hum Exp Toxicol. 2002. 21:533–536.


30. Rotter BA, Prelusky DB, Pestka JJ. Toxicology of deoxynivalenol (vomitoxin). J Toxicol Environ Health. 1996. 48:1–34.
31. Sahu SC, Garthoff LH, Robl MG, Chirtel SJ, Ruggles DI, Flynn TJ, Sobotka TJ. Rat liver clone-9 cells in culture as a model for screening hepatotoxic potential of food-related products: hepatotoxicity of deoxynivalenol. J Appl Toxicol. 2008. 28:765–772.


32. Sugita-Konishi Y, Hara-Kudo Y, Kasuga F, Kumagai S. Effect of trichothecenes on host defense against infectious diseases. Mycotoxins. 1998. 47:19–23.
33. Sun XM, Zhang XH, Wang HY, Cao WJ, Yan X, Zuo LF, Wang JL, Wang FR. Effects of sterigmatocystin, deoxynivalenol and aflatoxin G1 on apoptosis of human peripheral blood lymphocytes in vitro. Biomed Environ Sci. 2002. 15:145–152.
34. Tiemann U, Brüssow KP, Küchenmeister U, Jonas L, Kohlschein P, Pöhland R, Dänicke S. Influence of diets with cereal grains contaminated by graded levels of two Fusarium toxins on selected enzymatic and histological parameters of liver in gilts. Food Chem Toxicol. 2006. 44:1228–1235.


35. Trenholm HL, Hamilton RM, Friend DW, Thompson BK, Hartin KE. Feeding trials with vomitoxin (deoxynivalenol)-contaminated wheat: effects on swine, poultry, and dairy cattle. J Am Vet Med Assoc. 1984. 185:527–531.
36. Young LG, McGirr L, Valli VE, Lumsden JH, Lun A. Vomitoxin in corn fed to young pigs. J Anim Sci. 1983. 57:655–664.


37. Zhou HR, Harkema JR, Hotchkiss JA, Yan D, Roth RA, Pestka JJ. Lipopolysaccharide and the trichothecene vomitoxin (deoxynivalenol) synergistically induce apoptosis in murine lymphoid organs. Toxicol Sci. 2000. 53:253–263.

