Abstract
The canine transmissible venereal tumor (CTVT) is found mainly in dogs' sexual organs. Currently, it is widely accepted that all samples of CTVT show similar histopathological characteristics and share common genetic alterations. Despite the common genetic origin of CTVT, mutations in the P53 gene have been reported. In this study, we proposed that tumor samples can be genetically grouped using this gene. The presence of different subgroups of CTVT was determined in Mexican dogs using the TP53 gene sequence in CTVT samples. Four new polymorphisms were found and therefore, the CTVT samples were classified in five subgroups.
The canine transmissible venereal tumor (CTVT) is characterized by the vertical propagation of tumoral cells from an affected individual to a healthy one. Although CTVT is mainly found in the sexual organs of female and male dogs, it can become highly invasive to other tissues [7]. Metastasis occurs in low frequency and the tumors generally respond to antineoplasic treatment [1].
Several findings have highlighted that CTVT arose from an ancestral neoplasic cell, coming from a single host, through successions of selection and clonal expansion [3,8]. Accordingly, worldwide samples of CTVT show closely related histopathological characteristics and share common genetic alterations such as aneuploid karyotype with the presence of 58 to 59 chromosomes and a particular long interspersed nuclear element (LINE-1) insertion near c-myc oncogene [4].
Despite the common origin of CTVT, tumor samples from different countries can be genetically subdivided, depending on their geographic origin. For instance, the DQA 1 locus has been found to be diploid in some CTVT samples and haploid in others. In addition, two genetic subgroups based on mitochondrial DNA analysis have been characterized [5]. Moreover, a mutation found in position 963 of the TP53 gene in CTVT cells from Asian dogs [2], was absent in tumor samples from Mexican dogs [10]. Taken together, these data suggest the existence of several CTVT subgroups, probably because of the acquisition of new genetic alterations after the development of the original CTVT clone.
The TP53 gene is one of the most important suppressor genes associated with cancer development [11]. This gene represents a mutation hot spot in the mammalian genome and could be used as a marker of genetic diversity. To determine the presence of different subgroups of CTVT in Mexican dogs, 9 out of the 11 exons in the TP53 gene were sequenced in 5 CTVT samples. Four new polymorphisms were found. Considering the clonal origin of CTVT and the presence of various mutations in the TP53 gene, our data suggested that CTVT samples from different countries could be subgrouped based on TP53 polymorphisms.
All sample tumors were diagnosed by means of histopathology, using a hematoxylin and eosin stain and molecular diagnosis by PCR-identification of LINE insertion in c-myc oncogene, according to Liao et al. [4].
RNA was obtained from both 50 mg of fresh frozen tumors and 500 µL of total blood using trizol reagent (Invitrogen, USA) according to the manufacturer's instructions. Blood and tumor biopsies were always obtained from the same dogs. The amplification of exons 1-9 of TP53 gene was carried out by the one step RT-PCR system. Briefly, 1 ng of RNA was amplified using 25 µL of buffer 5×, 250 ng/µL of each primer previously designed in our laboratory JSTVT1F 5′CAGTCAGAGCTCAATATC GACCCCC3′ and JSTVT10R 5′AGGCAGTGCTCGCT TGGTAC3′, 1.0 µL/ 5 U of RT, and 5 µL of RNA.
The PCR purified products were cloned into the pGEM-T vector system (Promega, USA). Briefly, 2 mg of recombinant plasmids were used for the sequencing reaction with Big Dye Terminator v.3.1 cycle sequencing kit (Applied Biosystems, USA); sequencing reaction was performed in an ABI PRISM 3100 Sequence Detection System (Applied Biosystems, USA). The sequences obtained from CTVT samples were aligned with the sequences from blood samples and the reported sequence of the wild type TP53 gene in the Gen Bank (U62133), using Clustal W (DDBJ, Japan). The amino acid translation was performed using the Translate tool software found at the expasy tools site and the posterior alignment using Clustal W.
After analyzing the five CTVT samples, 4 different mutations were found. Only one of the studied samples matched 100% with the wild type sequence. A G to C transversion was identified in position 147 in two different samples, which did not affect the coded amino acid; as well as an A to G transition at nucleotide 625 in only one sample, which produced a change from glutamic acid to lysine at the 209 residue. In a fourth sample, the same kind of mutation was found, a G to A transition in two different positions, 83 and 680, respectively. The two last mutations produced an amino acid change from serine to asparagine (Table 1 and Fig. 1).
In the present study we found three different alleles in the TP53 gene in 5 samples of CTVT with the same histopathological characteristics and the Line/c-myc insertion. All of these mutations were different from the TP53 gene variant previously determined by Choi and Kim [2]. Our findings suggest that after the ancestral origin of the CTVT, the tumors have diverged into several different clones. Considering the TP53 mutations that were found, the CTVT tumor population could be further classified in at least five subgroups. However, in order to identify new alleles, determine their frequency and analyze the correlation between mutations and geographic regions, it is important to increase the number of samples for study and to obtain tumor samples from different countries around the world.
Given the important role played by TP53 in the maintenance of genome stability, cell cycle regulation, apoptosis and senescence, functional polymorphisms in this gene could deeply affect cancer development [6]. At present, more than 10 mutations in TP53 have been found in different canine neoplasias [9]. Although no polymorphisms of TP53 gene have been associated with CTVT tumorigenesis. This work shows that analyzed samples of CTVT present genetic diversity, probably as a result of the proliferation of CTVT cells. In future studies it would be important to establish if the polymorphisms originating in CTVT play an important role in the pathogenesis and response to chemotherapy of the disease.
Figures and Tables
Fig. 1
(A) Electropherograms of the sequence of the TP53 from canine transmissible venereal tumor (CTVT) samples, which show changes. Black arrows mark the change in nucleotides. (B) In the sequence alignment of the TP53 from CTVT samples, the nucleotide changes are marked in bold type. In the 5 samples of CTVT analyzed are observed four different mutations, producing three different alleles in the TP53 gene.
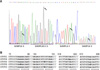
Acknowledgments
The authors would like to thank the Universidad Autónoma del Estado de México for their financial support.
References
1. Calvert CA, Leifer CE, MacEwen EG. Vincristine for treatment of transmissible venereal tumor in the dog. J Am Vet Med Assoc. 1982. 181:163–164.
2. Choi YK, Kim CJ. Sequence analysis of canine LINE-1 elements and p53 gene in canine transmissible venereal tumor. J Vet Sci. 2002. 3:285–292.


3. Foster RA. McGavin MD, Zachary JF, editors. Female and male reproductive system. Pathologic Basis of Veterinary Disease. 2006. 4th ed. St. Louis: Elsevier;1263–1315.
4. Liao KW, Lin ZY, Pao HN, Kam SY, Wang FI, Chu RM. Identification of canine transmissible venereal tumor cells using in situ polymerase chain reaction and the stable sequence of the long interspersed nuclear element. J Vet Diagn Invest. 2003. 15:399–406.


5. Murgia C, Pritchard JK, Kim SY, Fassati A, Weiss RA. Clonal origin and evolution of a transmissible cancer. Cell. 2006. 126:477–487.


7. Oduye OO, Ikede BO, Esuruoso GO, Akpokodje JU. Metastatic transmissible venereal tumour in dogs. J Small Anim Pract. 1973. 14:625–637.


8. Rebbeck CA, Thomas R, Breen M, Leroi AM, Burt A. Origins and evolution of a transmissible cancer. Evolution. 2009. 63:2340–2349.


9. Setoguchi A, Sakai T, Okuda M, Minehata K, Yazawa M, Ishizaka T, Watari T, Nishimura R, Sasaki N, Hasegawa A, Tsujimoto H. Aberrations of the p53 tumor suppressor gene in various tumors in dogs. Am J Vet Res. 2001. 62:433–439.

