Abstract
This study was to investigate the anti-obesity effects of diglyceride (DG)-conjugated linoleic acid (CLA) containing 22% CLA as fatty acids in C57BL/6J ob/ob male mice. There were four experimental groups including vehicle control, DG, CLA, and DG-CLA. The test solutions of 750 mg/kg dose were orally administered to the mice everyday for 5 weeks. CLA treatments significantly decreased mean body weight in the obese mice throughout the experimental period compared to the control (p < 0.01). All test solutions significantly decreased the levels of triglyceride, glucose and free fatty acids in the serum compared with control (p < 0.05). The levels of total cholesterol were also significantly reduced in DG and DG-CLA groups compared with the control group (p < 0.05). CLA significantly decreased weights of renal and epididymal fats compared with the control (p < 0.05). DG and DG-CLA also significantly decreased the epididymal fat weights compared with the control (p < 0.05). A remarkable decrease in the number of lipid droplets and fat globules was observed in the livers of mice treated with DG, CLA, and DG-CLA compared to control. Treatments of DG and CLA actually increased the expression of peroxisome proliferator-activated receptor gamma. These results suggest that DG-CLA containing 22% CLA have a respectable anti-obesity effect by controlling serum lipids and fat metabolism.
Obesity is a major public health problem and main cause of most of geriatric diseases in Western countries. It is associated with many health risks, including heart disease, diabetes mellitus, stroke, high blood pressure, gallbladder disease, and some forms of cancer [17,26]. According to a recent report from the National Health and Nutrition Examination Survey in the United States, it was estimated that over 65% of adults were overweight or obese, and 16% of children were overweight [13,31]. Although diet, especially dietary fat, has been recognized as contributing to the development of obesity, differential effects have arisen with respect to individual fatty acids [15,17].
The physiological and anti-obesity effects of diglyceride (DG), which consists mainly of 1,3-DG, have been reported in numerous studies [27,38]. A single dose of DG emulsion lowers the extent of increase in postprandial serum triglyceride (TG) levels in rats [38]. Dietary DG, in contrast to TG, decreases both body weight and visceral fat mass as determined by computed tomography in healthy men [29]. In addition, dietary DG suppresses the accumulation of high-fat and high-sucrose diet-induced body fat in C57BL/6J mice [28].
Conjugated linoleic acid (CLA) refers to a group of isomers of linoleic acid (cis-9, cis-12 octadecadienoic acid). These isomers can either be positional (shifting of double bonds to 9~11 or 10~12 positions), geometric (cis/trans variations), or a combination of both. The major dietary source of CLA for humans is ruminant meats such as beef and lamb, and diary products including milk and cheese [10,22,34]. CLA has been reported to be anticarcinogenic [10,34], antiatherogenic [19], and immunomodulating agents [37]. More recently, a crude mixture of CLA isomers has been shown to reduce body fat and enhance fat-free mass in animals and humans [2,33]. In addition, the treatment of CLA during adipocyte differentiation reduces lipid accumulation and inhibits the expression of peroxisome proliferator-activated receptor gamma (PPAR-γ), which is a nuclear receptor that activates genes involved in lipid storage and metabolism [4,14].
PPAR-γ is expressed at the highest level in adipose tissue [9], colon epithelium [21], and macrophages [25]. In contrast to these tissues or cells, the expression of PPAR-γ in the liver is very low [12] and the function of PPAR-γ in the liver is unclear. However, it is noteworthy that PPAR-γ is expressed at elevated levels in the liver of a number of murine models of diabetes or obesity, including acid binding protein (aP2)/DTA [7], A-ZIP/F1 [8], ob/ob [23], db/db [23], and KKA [3], mutant mice. Levels of hepatic PPAR-γ are elevated by seven- to nine-fold in ob/ob and db/db mice compared with wild-type mice [23].
DG and CLA have been reported to have anti-obesity effects in humans and animals [19,28,29,33]. In the present study, DG-CLA may have a synergistic effect on anti-obesity compared to DG or CLA alone. Here, we investigated the effects of DG, CLA, and DG-CLA on anti-obesity in an animal model of C57BL/6J ob/ob mice as determined with body weight, serum lipid levels, abdominal fat weights, lipid droplets in liver, and PPAR-γ expression.
Experimental materials including DG, CLA, and DG-CLA were obtained from the Illshinwells (Korea). CLA typically produced for experimental purposes was composed of the cis-9,trans-11 and trans-10,cis-12 isomers (approximately a 50 : 50 ratio). The composition of fatty acids of DG, CLA, and DG-CLA was analyzed by gas-liquid chromatography (Table 1). DG-CLA was produced according to patent No. 10-0540875 (Illshinwells, Korea).
Five week-old C57BL/6J ob/ob male mice were obtained from Japan SLC (Japan). The animal room was maintained as follows; a 12-h light/dark cycle, 10-times room air changes per h, 21~24℃ temperature and 35~65% relative humidity. Animal experiments were performed in accordance with Standard Operation Procedures of Laboratory Animals that were approved by Institutional Animal Care and Use Committee of Laboratory Animal Research Center at Chungbuk National University.
Mice weighing an average of about 47 g were divided into 4 groups, including vehicle control, DG, CLA, and DG-CLA. Animals were treated orally with 10 mL/kg of 0.5% methyl cellulose for the control and 750 mg/kg for the three test solutions for the treated groups daily for 5 weeks. The body weight was measured twice a week and the feed and water intake were measured every week. After 5 weeks, animals were anesthetized with ether and blood samples were collected by a syringe from the abdominal aorta and immediately transferred into serum separator tubes. The liver was sampled for microscopical examination.
Serum was separated by centrifuging whole blood at 3,000 rpm for 20 min. Serum lipids were analyzed using a blood chemistry analyzer (Hitachi, Japan). The levels of serum TG, total cholesterol (T-CHO), low-density lipoproteins (LDL), high-density lipoproteins (HDL), glucose (Glu), glutamic oxaloacetic transaminase (GOT), γ-glutamatepyruvate transaminase (γ-GPT), blood urea nitrogen (BUN), and creatinine (CRE) were determined.
Abdominal fats including mesentery, renal, and epididymal fats were carefully separated and weighed. The relative fat weight (%) was calculated based on final body weight.
Mice livers were fixed in 10% neutral buffer formalin and embedded in paraffin. Sections of 4-µm thickness were stained with hematoxylin and eosin according to the general procedures. In addition, the livers frozen in a deep freezer (Revco, USA) were embedded in OCT compounds, and 10-µm thick sections were stained with oil red O. Morphology of the livers was examined under a light-microscope (Olympus, Japan).
The livers were homogenized in a lysis buffer containing 20 mM Hepes (pH 7.5), 150 mM NaCl, 1% Triton X-100, 1 mM EDTA, 1 mM EGTA, 100 mM NaF, 10 mM sodium pyrophophate, and 1 mM Na3VO3. The soluble materials were removed by centrifugation at 12,000 rpm for 20 min and protein level was determined using the Bradford protein assay. The lysates (50 µg of protein) were resolved on a sodium dodacyl sulfate-10% polyacrylamide gel and transferred onto PVSF membrane (Hoefer, USA). The blots were blocked with 5% skim milk in TNT (20 mM Tris-HCl, pH 7.5, 150 mM NaCl and 0.1% Tween 20) solution and then incubated at 4℃ overnight with either PPAR-γ antibody (1 : 1,000; Santa Cruz Biotechnology, USA) or GAPDH (1 : 1,000; Cell Signaling Technology, USA) diluted with 3% skim milk. The blots were then incubated with anti-mouse horseradish peroxidase-conjugated antibody (1 : 1,000; Cell Signaling Technology, USA) or anti-rabbit horseradish peroxidase-conjugated antibody (1 : 1,000; Cell Signaling Technology, USA). Signals were detected by using the enhanced chemiluminescence method using WEST-ONE (iNtRON Biotechnology, Korea).
Data were expressed as the mean ± SE. Statistical significance between control group and treatment groups were determined by one-way ANOVA, followed by the LSD using the SPSS 10.0 statistic computer program. A difference at the level of p < 0.05 or p < 0.01 was considered to be statistically significant.
CLA treatment significantly decreased the mean body weights in the obese mice throughout the experimental periods compared with the control (Fig. 1) (p < 0.01). Meanwhile, treatments of DG or DG-CLA did not significantly decrease the mean body weight compared to control (Fig. 1). The decrease in body weight by CLA was also significantly different from the DG or DG-CLA groups (p < 0.05). The daily feed intake for the experimental period in the CLA group was significantly lower than the control and DG groups (Fig. 2) (p < 0.01). The reduction of body weight was strongly associated with the decrease in food consumption of the obese mice.
The obese mice (C57BL/6J ob/ob) had greater levels of TG, T-CHO, HDL, LDL, Glu, FFA, GOT, and γ-GPT, compared with the respective lean mice (C57BL/6J) (data not shown). The DG, CLA, and DG-CLA treatments significantly decreased serum TG levels in the obese mice (Table 2, p < 0.05). DG and DG-CLA groups had a significant reduction T-CHO levels and a significant increase in serum HDL levels compared with the control group (Table 2, p < 0.05). The levels of Glu and FFA were also significantly decreased by DG, CLA, and DG-CLA treatments (Table 2). DG-CLA had the strongest reducing effect on serum Glu and FFA levels among the treatments. CLA significantly increased serum γ-GPT and CRE levels, while DG-CLA significantly decreased BUN levels compared with the control (p < 0.05).
Abdominal fat weights, including mesentery, renal, and epididymal fats were measured in relation to final body weight (Table 3). There were no significant differences in mesentery fat weight among experimental groups. CLA significantly decreased renal fat weight compared to control (p < 0.05), while DG significantly increased the renal fat weight (Table 3). DG, CLA, and DG-CLA treatments caused a significant decrease in epididymal fat weight compared with the control (p < 0.05). The abdominal fat weight, as the sum of the weights of mesentery, renal, and epididymal fats, was only significantly decreased by CLA treatments.
Livers of the control mice (C57BL/6J ob/ob) had numerous lipid droplets compared with those from lean normal mice (C57BL/6J) (data not shown). Large fat droplets were present diffusely in the liver of the control obese mice (Fig. 3). The treatment CLA and DG-CLA showed a reasonable decrease in the number of lipid droplets compared to the control (Fig. 3). In the liver stained with oil red O, a remarkable decrease in lipid droplets by CLA and DGCLA treatments were clearly shown, compared with the control (Fig. 4). Treatment of DG also weakly ameliorated the lipid accumulation in the liver cells compared with the control (Fig. 4).
CLA has been approved as a functional food for controlling obesity in humans [33]. The objective of the present study was to investigate the anti-obesity effects of DG, CLA and DG-CLA containing 22% CLA as fatty acids in C57BL/6J ob/ob mice. In the present study, CLA decreased body weight, serum lipids levels, and abdominal fat mass without hepatotoxicity and nephrotoxicity. DG and DG-CLA also decreased the serum levels of TG, T-CHO, Glu, and FFA, compared with the control. The effects on these anti-obesity biomarkers may be derived from the well-known functions of DG and CLA in previous studies [19,28,33,39].
Recently, dietary CLA has been shown to reduce body fat mass in various experimental animals, including lean/obese mice [11,24,32,35,36,41]. The C57BL/6J ob/ob mouse has been widely used in obesity research as a representative animal of obesity [21,28]. Obesity in these mice is result of a point mutation in the leptin gene, Lep. The ligand, leptin, has been shown to be a key weight control hormone that is mutated in the mouse obesity mutation [18].
To care and prevent obesity, decreases in body weight and body fat are important as the preferential target [11,13,41]. Several investigators have reported that CLA is an effective regulator of body fat accumulation and retention [35,36]. This five weeks trial using C57BL/6J ob/ob mice and treatment with CLA significantly decreased body weight in this study. The body weight of the animals was closely related to daily food consumption. The ob/ob or diet-induced obese mice receiving a recombinant form of ciliary neurotrophic factor exhibit preferential loss of fat mass and a decrease in feed consumption [18]. The treatment of CLA reduced the food consumption that may be associated with the significant weight loss in the obese mice in this study. The CLA inhibited abdominal fat weights which may be associated with their suppression of adipocyte differentiation and adipogenesis. Mesentery, renal, and epididymal fat mass in the abdomen of mice were significantly decreased by CLA treatment. The specific mechanism by which CLA exerts these effects remains unknown, but several hypotheses have been offered, including the inhibition of arachidonic acid formation and modulation of desaturase activity in the liver [20,37]. Obese mice have numerous metabolic abnormalities associated with lipid metabolism including increased lipid accretion and reduced desaturase activity [1,5].
The lipid accumulation of adipocytes is determined by a balance of lipogenesis and lipolysis. In the present study CLA and DG-CLA ameliorated the lipid accumulation in the liver of obese mice compared with the control. DG has also been reported to have anti-obesity effects in numerous studies showing decreased body weight, visceral fat, and serum TG levels with treatment [28,29]. In this study, DG and DG-CLA decreased total cholesterol levels, and DG, CLA, and DG-CLA decreased serum TG levels. These results show that CLA and/or DG can regulate lipogenesis and lipolysis to maintain lipid levels in the blood of the obese mice. The decreased levels of serum TG, Glu, and FFA by test solutions might be associated with the decrease of food intake in this study. However, the reducing effects on these serum values were stronger in the DG or DG-CLA group than the CLA group, indicating that their effects were not relevant to the daily feed intake.
The PPAR-γ, CC/AAT/enhancer binding protein α, and fatty aP2 are transcription factors known to be important in adipocyte differentiation and maturation [27]. The mechanism of how hepatic PPAR-γ is induced in the liver of ob/ob mouse remains elusive. It is known that the expression of hepatic PPAR-γ is increased in some obese and diabetic model mice [7]. In addition, the function of PPAR-γ in the liver is still unclear. A down-regulation of the genes might be correlated with the subsequent attenuation of lipid accumulation as described above. In this study, the treatment of DG and CLA remarkably increased PPAR-γ expression compared with the control. Some workers reported that t10, c12-CLA, but not c9 and t11-CLA activates PPAR-γ [16]. In adipose tissue, CLA t10 and c12 decreased adipogenesis by a mechanism that involves decreased expression of PPAR-γ [6]. In contrast, CLA increases both PPAR-γ mRNA expression and insulin sensitivity in rats [23]. In addition, prolonged treatment with CLA activated the expression of PPAR-γ in lepob/lepob mice [40]. Therefore, activation or depression of PPAR-γ may differ for various conditions. In our study, DG and CLA actually increased PPAR-γ expression in the liver of the C57BL/6J ob/ob mice, but its function in the liver is still elusive.
In conclusion, CLA decreased body and abdominal fat weights and serum lipid levels, most likely due to a loss of appetite and modulation of lipid metabolism in C57BL/6J ob/ob mice. DG and DG-CLA also decreased serum levels of TG, T-CHO, Glu, and FFA more strongly than CLA. Although DG and DG-CLA did not decrease body weight, they may play an important role in lipid metabolism, resulting in improving human health.
Figures and Tables
Fig. 1
Change in body weights of C57BL/6J ob/ob male mice for 5 weeks. DG: diglyceride, CLA: conjugated linoleic acid, DG-CLA: DG containing 22% CLA as fatty acid. Data is expressed as the means ± SE (n = 10). *Significantly different from the control at p < 0.01.
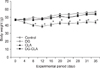
Fig. 2
Daily feed intake of C57BL/6J ob/ob mice for 5 weeks. Data represent the means ± SE (n = 10). *Significantly different from the control at p < 0.01.
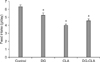
Fig. 3
Microphotographs of the liver of C57BL/6J ob/ob mice. Many fat droplets were diffusely present in the liver of the obese mice in control and treated with test solutions. (A) Control, (B) Diglyceride, (C) Conjugated linoleic acid, (D) Diglyceride containing 22% conjugated linoleic acid. H&E stain, ×200.
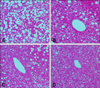
Fig. 4
Lipid accumulation in the liver of C57BL/6J ob/ob mice. Many fat droplets were diffusely present in the liver of the obese mice with control (A), while the treatments of diglyceride (B), conjugated linoleic acid (C), and diglyceride containing 22% conjugated linoleic acid (D) decreased the number of fat droplets. Oil red O staining, ×200.
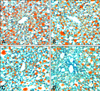
Fig. 5
Expression of PPAR-γ in the liver of C57BL/6J ob/ob mice. All samples in gels were equally loaded with 50 µg of total protein and GAPDH was used as an internal control for equal protein loading. As compared with the control (A), the expression of PPAR-γ increased with the treatment of diglyceride (B), conjugated linoleic acid (C), and diglyceride containing 22% conjugated linoleic acid as fatty acid (D).
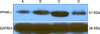
Table 2
Serum chemistry in C57BL/6J ob/ob mice
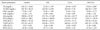
DG-CLA: DG containing 22% CLA as fatty acid, TG: triglyceride, T-CHO: total cholesterol, LDL: low-density lipoproteins, HDL: high-density lipoproteins, Glu: glucose, FFA: free fatty acid, GOT: glutamic oxaloacetic transaminase, γ-GPT: glutamate-pyruvate transaminase, BUN: blood urea nitrogen, CRE: creatinine. Data represent means ± SE (n = 6). *Significant different from the control group at the level of p < 0.05.
Acknowledgments
This study was supported by the Bio Organic Material & Food Center at Seowon University, a part of Regional Innovation Center Program of the Ministry of Commerce, Industry and Energy in Korea.
References
1. Azain MJ, Hausman DB, Kasser TR, Martin RJ. Effect of somatotropin and feed restriction on body composition and adipose metabolism in obese Zucker rats. Am J Physiol. 1995. 269:E137–E144.


2. Banni S, Carta G, Angioni E, Murru E, Scanu P, Melis MP, Bauman DE, Fischer SM, Ip C. Distribution of conjugated Linoleic acid and metabolites in different lipid fractions in the rat liver. J Lipid Res. 2001. 42:1056–1061.


3. Bedoucha M, Atzpodien E, Boelsterli UA. Diabetic KKAy mice exhibit increased hepatic PPARgamma1 gene expression and develop hepatic steatosis upon chronic treatment with antidiabetic thiazolidinediones. J Hepatol. 2001. 35:17–23.


4. Bocher V, Pineda-Torra I, Fruchart JC, Staels B. PPARs: transcription factors controlling lipid and lipoprotein metabolism. Ann N Y Acad Sci. 2002. 967:7–18.


5. Blond JP, Henchiri C, Bézard J. Delta 6 and delta 5 desaturase activities in liver from obese Zucker rats at different ages. Lipids. 1989. 24:389–395.


6. Brown JM, McIntosh MK. Conjugated linoleic acid in humans: regulation of adiposity and insulin sensitivity. J Nutr. 2003. 133:3041–3046.


7. Burant CF, Sreenan S, Hirano K, Tai TA, Lohmiller J, Lukens J, Davidson NO, Ross S, Graves RA. Troglitazone action is independent of adipose tissue. J Clin Invest. 1997. 100:2900–2908.


8. Chao L, Marcus-Samuels B, Mason MM, Moitra J, Vinson C, Arioglu E, Gavrilova O, Reitman ML. Adipose tissue is required for the antidiabetic, but not for the hypolipidemic, effect of thiazolidinediones. J Clin Invest. 2000. 106:1221–1228.


9. Chawla A, Schwarz EJ, Dimaculangan DD, Lazar MA. Peroxisome proliferator-activated receptor (PPAR) gamma: adipose-predominant expression and induction early in adipocyte differentiation. Endocrinology. 1994. 135:798–800.


10. Chin SF, Liu W, Storkson JM, Ha YL, Pariza MW. Dietary sources of conjugated dienoic isomers of linoleic acid, a newly recognized class of anticarcinogens. J Food Comp Anal. 1992. 5:185–197.


11. DeLany JP, Blohm F, Truett AA, Scimeca JA, West DB. Conjugated Linoleic acid rapidly reduces body fat content in mice without affecting energy intake. Am J Physiol. 1999. 276:R1172–R1179.
12. Fajas L, Auboeuf D, Raspé E, Schoonjans K, Lefebvre AM, Saladin R, Najib J, Laville M, Fruchart JC, Deeb S, Vidal-Puig A, Flier J, Briggs MR, Staels B, Vidal H, Auwerx J. The organization, promoter analysis, and expression of the human PPARgamma gene. J Biol Chem. 1997. 272:18779–18789.


13. Flegal KM. Epidemiologic aspects of overweight and obesity in the United States. Physiol Behav. 2005. 86:599–602.


14. Granlund L, Pedersen JI, Nebb HI. Impaired lipid accumulation by trans10, cis12 CLA during adipocyte differentiation is dependent on timing and length of treatment. Biochim Biophys Acta. 2005. 1687:11–22.


15. Hihi AK, Michalik L, Wahli W. PPARs: transcriptional effectors of fatty acids and their derivatives. Cell Mol Life Sci. 2002. 59:790–798.


16. Houseknecht KL, Vanden Heuvel JP, Moya-Camarena SY, Portocarrero CP, Peck LW, Nickel KP, Belury MA. Dietary conjugated linoleic acid normalizes impaired glucose tolerance in the Zucker diabetic fatty fa/fa rat. Biochem Biophys Res Commun. 1998. 244:678–682.


18. Lambert PD, Anderson KD, Sleeman MW, Wong V, Tan J, Hijarunguru A, Corcoran TL, Murray JD, Thabet KE, Yancopoulos GD, Wiegand SJ. Ciliary neurotrophic factor activates leptin-like pathways and reduces body fat, without cachexia or rebound weight gain, even in leptin-resistant obesity. Proc Natl Acad Sci USA. 2001. 98:4652–4657.


19. Lee KN, Kritchevsky D, Pariza MW. Conjugated linoleic acid and atherosclerosis in rabbits. Atherosclerosis. 1994. 108:19–25.


20. Lee KN, Pariza MW, Ntambi JM. Conjugated linoleic acid decreases hepatic stearoyl-CoA desaturase mRNA expression. Biochem Biophys Res Commun. 1998. 248:817–821.


21. Lefebvre AM, Chen I, Desreumaux P, Najib J, Fruchart JC, Geboes K, Briggs M, Heyman R, Auwerx J. Activation of the peroxisome proliferator-activated receptor gamma promotes the development of colon tumors in C57BL/6J-APCMin/+ mice. Nat Med. 1998. 4:1053–1057.


22. Lin H, Boylston TD, Chang MJ, Luedecke LO, Shultz TD. Survey of the conjugated linoleic acid contents of dairy products. J Dairy Sci. 1995. 78:2358–2365.


23. Memon RA, Tecott LH, Nonogaki K, Beigneux A, Moser AH, Grunfeld C, Feingold KR. Up-regulation of peroxisome proliferator-activated receptors (PPAR-alpha) and PPAR-gamma messenger ribonucleic acid expression in the liver in murine obesity: troglitazone induces expression of PPAR-gamma-responsive adipose tissue-specific genes in the liver of obese diabetic mice. Endocrinology. 2000. 141:4021–4031.


24. Miner JL, Cederberg CA, Nielsen MK, Chen X, Baile CA. Conjugated linoleic acid (CLA), body fat, and apoptosis. Obes Res. 2001. 9:129–134.


25. Moore KJ, Fitzgerald ML, Freeman MW. Peroxisome proliferator-activated receptors in macrophage biology: friend or foe? Curr Opin Lipidol. 2001. 12:519–527.


26. Must A, Spadano J, Coakley EH, Field AE, Colditz G, Dietz WH. The disease burden associated with overweight and obesity. JAMA. 1999. 282:1523–1529.


27. Murase T, Aoki M, Wakisaka T, Hase T, Tokimitsu I. Anti-obesity effect of dietary diacylglycerol in C57BL/6J mice: dietary diacylglycerol stimulates intestinal lipid metabolism. J Lipid Res. 2002. 43:1312–1319.


28. Murase T, Mizuno T, Omachi T, Onizawa K, Komine Y, Kondo H, Hase T, Tokimitsu I. Dietary diacylglycerol suppresses high fat and high sucrose diet-induced body fat accumulation in C57BL/6J mice. J Lipid Res. 2001. 42:372–378.


29. Nagao T, Watanabe H, Goto N, Onizawa K, Taguchi H, Matsuo N, Yasukawa T, Tsushima R, Shimasaki H, Itakura H. Dietary diacylglycerol suppresses accumulation of body fat compared to triacylglycerol in men in a double-blind controlled trial. J Nutr. 2000. 130:792–797.


30. Ntambi JM, Kim YC. Adipocyte differentiation and gene expression. J Nutr. 2000. 130:3122S–3126S.


31. Ogden CL, Carroll MD, Curtin LR, McDowell MA, Tabak CJ, Flegal KM. Prevalence of overweight and obesity in the United States, 1999-2004. JAMA. 2006. 295:1549–1555.


32. Ohnuki K, Haramizu S, Ishihara K, Fushiki T. Increased energy metabolism and suppressed body fat accumulation in mice by a low concentration of conjugated linoleic acid. Biosci Biotechnol Biochem. 2001. 65:2200–2204.


33. Pariza MW, Park Y, Cook M. The biologically active isomers of conjugated Linoleic acid. Prog Lipid Res. 2001. 40:283–298.


34. Parodi PW. Cows' milk fat components as potential anticarcinogenic agents. J Nutr. 1997. 127:1055–1060.


35. Park Y, Albright KJ, Storkson JM, Liu W, Cook ME, Pariza MW. Changes in body composition in mice during feeding and withdrawal of conjugated linoleic acid. Lipids. 1999. 34:243–248.


36. Park Y, Storkson JM, Albright KJ, Liu W, Pariza MW. Evidence that the trans-10, cis-12 isomer of conjugated linoleic acid induces body composition changes in mice. Lipids. 1999. 34:235–241.


37. Parker J, Daniel LW, Waite M. Evidence of protein kinase C involvement in phorbol diester-stimulated arachidonic acid release and prostaglandin synthesis. J Biol Chem. 1987. 262:5385–5393.


38. Taguchi H, Nagao T, Watanabe H, Onizawa K, Matsuo N, Tokimitsu I, Itakura H. Energy value and digestibility of dietary oil containing mainly 1,3-diacylglycerol are similar to those of triacylglycerol. Lipids. 2001. 36:379–382.


39. Terpstra AHM, Javadi M, Beynen AC, Kocsis S, Lankhorst AE, Lemmens AG, Mohede ICM. Dietary conjugated linoleic acids as free fatty acids and triacylglycerols similarly affect body composition and energy balance in mice. J Nutr. 2003. 133:3181–3186.


40. Wargent E, Sennitt MV, Stocker C, Mayes AE, Brown L, O'Dowd J, Wang S, Einerhand AW, Mohede I, Arch JR, Cawthorne MA. Prolonged treatment of genetically obese mice with conjugated linoleic acid improves glucose tolerance and lowers plasma insulin concentration: possible involvement of PPAR activation. Lipids Health Dis. 2005. 4:3.
41. West DB, Delany JP, Camet PM, Blohm F, Truett AA, Scimeca J. Effects of conjugated linoleic acid on body fat and energy metabolism in the mouse. Am J Physiol. 1998. 275:R667–R672.