Abstract
Purpose
This study was designed to investigate the role of taurine-upregulated gene 1 (TUG1) in MCF-7 breast cancer cells and the molecular mechanism involved in the regulation of microRNA-9 (miR-9).
Methods
The expression of TUG1 in breast cancer tissues and cells was evaluated using quantitative reverse transcription polymerase chain reaction. Cell viability was examined using a 3-(4,5-dimethyl-2-thiazolyl)-2,5-diphenyl-2-H-tetrazolium bromide (MTT) assay; cell cycle progression and apoptosis were analyzed using flow cytometry. A dual luciferase reporter assay was used to detect the relationship between TUG1 and miR-9. The expression of methylenetetrahydrofolate dehydrogenase 2 (MTHFD2) was measured by western blot.
Results
Higher expression of TUG1 was observed in breast cancer tissues and cell lines than in the corresponding controls. TUG1 knockdown reduced proliferation, suppressed cell cycle progression, and promoted apoptosis of MCF-7 cells. The dual luciferase reporter assay showed that TUG1 could negatively regulate the expression of miR-9. MiR-9 inhibition abrogated the effect of TUG1 knockdown on the proliferation, cell cycle progression, and apoptosis of MCF-7 cells. TUG1 positively regulated the expression of MTHFD2 in breast cancer cells.
Breast cancer is the most common malignant tumor in women, and its incidence has been increasing year on year [1]. In addition, the age of breast cancer onset is decreasing [2]. Approximately 500,000 women die of breast cancer world-wide annually. With the development of improved diagnostic and clinical therapeutic methods, the efficiency of breast cancer treatment has improved greatly. At the same time, gene therapy against tumors is a growing area of research [3]. Therefore, there is an urgent need to identify a target gene for the treatment for breast cancer.
Noncoding RNAs (ncRNAs), which constitute the majority of transcripts encoded by the human genome, have recently gained considerable attention for their role in a variety of cellular functions and disease processes such as cancer cell proliferation, metastasis, and apoptosis [4]. Long noncoding RNAs (lncRNAs) and microRNAs (miRNAs) constitute the main ncRNAs. LncRNAs are defined as transcripts longer than 200 nucleotides with no protein-coding potential. To date, several lncRNAs have been found to be dysregulated in breast cancer and to be closely associated with the diagnosis and prognosis of breast cancer, such as metastasis-associated lung adenocarcinoma transcript 1 (MALAT1), nuclear enriched abundant transcript 1 (NEAT1), and breast cancer antiestrogen resistance 4 (BCAR4) [567]. LncRNA taurine upregulated gene 1 (TUG1) was initially identified as an essential gene for retinal development in the developing mouse eye [8]. Subsequent research revealed that TUG1 was abnormally regulated in tumorigenesis, either as a potential tumor suppressor or as an oncogene [910]. However, the role of TUG1 in breast cancer is still poorly understood.
As an another important type of ncRNA, miRNAs can regulate gene expression by binding to 3’-untranslated regions (3’-UTRs) of target mRNAs, causing gene silencing or translational repression [11]. MicroRNA-9 (miR-9) was originally found to contribute to brain development [12]. Recently, several studies have demonstrated the carcinogenic or carcino-static role of miR-9 [1314]. For example, miR-9 is upregulated in bladder cancer tissues and promotes cell proliferation by targeting longevity assurance homolog 2 of yeast LAG1 (LASS2) [14], whereas in nasopharyngeal carcinoma, miR-9 functions as a potential tumor suppressor by regulating the expression of C-X-C chemokine receptor type 4 (CXCR4) [13]. In breast cancer, miR-9 was shown to be downregulated and associated with epithelial-mesenchymal transition, breast cancer stem cell phenotype, and tumor progression [15].
In this study, we aimed to investigate the role of TUG1 in MCF-7 breast cancer cells and the molecular mechanism involved in the regulation of miR-9. Our findings will provide new insights into the molecular functions of TUG1 as well as its regulatory mechanisms in breast cancer.
A total of 24 breast cancer tissues and adjacent tissues were obtained from patients at The First Affiliated Hospital of Chongqing Medical University. Tissues were stored at -80℃ until use. The study was approved by the Ethics Committee of The First Affiliated Hospital of Chongqing Medical University (approval number: 2015-05-008). Informed consent was obtained from all participants.
Human breast cancer cell lines BT474, MCF-7, MDA-MB-231, and T47D, and the normal mammary epithelial cell line 76N were obtained from the American Type Culture Collection (Manassas, USA). Cells were maintained in Roswell Park Memorial Institute (RPMI)-1640 medium (Sigma, Shanghai, China) supplemented with 10% fetal bovine serum (Invitrogen, Carlsbad, USA) and 100 units/mL of penicillin-streptomycin (Invitrogen), and were kept in a humidified incubator at 37℃ with 5% CO2.
An miR-9 mimic, an miR-9 inhibitor, and the corresponding controls were purchased from Shanghai GenePharma Co., Ltd. (Shanghai, China). Three small interfering RNAs (si-RNAs) specifically targeting TUG1 (si-TUG1, si-TUG1 2, and si-TUG1 3) and the control si-RNA (si-NC) were purchased from Ribobio (Guangzhou, China). For the in vivo experiments, the si-TUG1 sequence was cloned into plasmid vector PEV-Lv208, which was performed by Ribobio, yielding shRNA-TUG1. The vector pcDNA-TUG1 for the overexpression of TUG1 and the control pcDNA were constructed and purchased from Ribobio. Twenty-four hours before transfection, MCF-7 cells were seeded into six well plates. Cells were then transfected with the miR-9 mimic, miR-9 inhibitor, si-TUG1, pcDNA-TUG1, or the controls using Lipofectamine 2000 Reagent (Invitrogen), according to the manufacturer's instructions.
From the database starBase v2.0, we found that miR-9 potentially binds to TUG1. The binding sequence in the TUG1 gene was amplified by polymerase chain reaction (PCR) and cloned downstream of the luciferase gene in the pGL3 vector (Promega, Madison, USA), yielding the plasmid TUG1-WT. The plasmid TUG1-MUT containing the mutant seed region of TUG1 was obtained through site-specific mutagenesis on the base of the plasmid TUG1-WT. TUG1-WT, TUG1-MUT, miR-9 mimic, or miR-9 inhibitor were co-transfected into MCF-7 cells using Lipofectamine 2000. The dual luciferase reporter assay system (Promega) was used to detect luciferase activity 48 hours after transfection.
The total RNA was extracted from breast cancer tissues or cells with TRIzol reagent (Invitrogen) according to the manufacturer's protocol. RNA was reverse transcribed to complementary DNA (cDNA) using a Reverse Transcription Kit (Takara, Dalian, China). Quantitative PCR analyses were conducted with SYBR Premix Ex Taq (Takara). For the expression of miR-9, the Taqman MicroRNA Reverse Transcription Kit (Applied Biosystems Inc., Foster City, USA) and the Taqman Universal Master Mix II (Applied Biosystems Inc.) were used for the reverse transcription reaction and quantitative PCR, respectively. The results for TUG1 and methylenetetrahydrofolate dehydrogenase 2 (MTHFD2) were normalized to the expression of glyceraldehyde-3-phosphate dehydrogenase (GAPDH), and miR-9 to the expression of U6. The relative expression of each gene was calculated and normalized using the 2-ΔΔCt method.
A Western blot assay was performed to detect the protein level of MTHFD2 in cultured cells. Total protein was extracted using lysis buffer. Protein concentration was measured with the Bradford method. Equal amounts of protein were isolated using sodium dodecyl sulfate polyacrylamide gel electrophoresis (SDS-PAGE) and then transferred to a poly-vinylidene fluoride membrane (Millipore, Bedford, USA). After blocking in 5% nonfat skim milk/tris-buffered saline-Tween, the membrane was incubated with anti-MTHFD2 antibody (Cell Signaling Technology, Danvers, USA) at 4℃ overnight. Autoradiograms were quantified by densitometry, and a GAPDH antibody was used as the control.
Cell viability was monitored using a 3-(4,5-dimethyl-2-thiazolyl)-2,5-diphenyl-2-H-tetrazolium bromide assay (Sigma). The transfected cells were seeded in 96-well plates (5×103 cells/well). Cell viability was determined every 24 hours after the transfection. MTT (100 µL, 0.5 mg/mL; Sigma) was added to each well and the incubation, continued at 37℃ for 4 hours. The precipitate was dissolved using dimethyl sulfoxide (DMSO, 150 µL). The absorbance at 490 nm was measured using a microplate spectrophotometer (Thermo, Waltham, USA).
For cell cycle analysis, 48 hours after the transfection, cells were harvested and fixed with 75% ethanol at 4℃ overnight. The fixed cells were incubated with propidium iodide (50 µg/mL) for 30 minutes in the dark at 37℃. Then the cells were analyzed using a FACScan (Becton-Dickinson, Franklin Lakes, USA). The ModFit software (Becton-Dickinson) was used to quantify the cells in different phases of the cell cycle.
For apoptosis analysis, dual staining using the FITC Annexin V apoptosis Detection Kit (Sigma) was performed according to the manufacturer's instructions. Apoptosis was analyzed with FACS using CellQuest software (Becton-Dickinson).
All animal procedures were carried out according to the guidelines approved by the China Association of Laboratory Animal Care. BALB/c-nu/nu mice (male; 4 weeks old; SLAC Laboratory Animal, Shanghai, China) were randomly divided into two groups (n=5). Cultured MCF-7 cells transfected with shRNA-TUG1 or shRNA-NC were suspended in 0.2 mL phosphate buffer saline and then injected subcutaneously into the right flank of the mice. Three mice from each group were used to obtain photographs of the tumors. The tumor size was measured after three weeks and the tumor volume (mm3) was estimated using the equation: length×(width)2×0.5.
SPSS version 17.0 software (SPSS Inc., Chicago, USA) was used for statistical analysis. Results are expressed as the mean±standard deviation. Student t-test was used to estimate the significant differences between groups. In the experiment, p<0.05 was considered as statistically significant.
The expression of TUG1 was detected in breast cancer tissues and the adjacent tissues. The results of quantitative reverse transcription polymerase chain reaction (RT-qPCR) showed a significantly higher level of TUG1 in breast cancer tissues than in the controls (Figure 1A). We then investigated the level of TUG1 in four breast cancer cell lines (BT474, MCF-7, MDA-MB-231, and T47D) and the control cell line 76N. TUG1 expression was higher in the breast cancer cell lines than in the control cells (Figure 1B). Specially, MCF-7 cells expressed the highest level of TUG1: 5.7 times that of the control cells.
To investigate the function of TUG1 in the development of breast cancer, three si-RNAs targeting TUG1 (si-TUG1, si-TUG1 2, and si-TUG1 3) were used to inhibit TUG1 expression. In MCF-7 cells transfected with si-TUG1, si-TUG1 2, and si-TUG1 3, the expression of TUG1 was significantly lower than that in cells transfected with si-NC (Figure 2A). An MTT assay was then performed to explore the effect of TUG1 knockdown on the proliferation of MCF-7 cells. TUG1 knockdown induced by si-TUG1, si-TUG1 2, and si-TUG1 3 greatly inhibited cell proliferation (Figure 2B). In terms of cell cycle progression, flow cytometry analysis revealed that TUG1-knockdown cells were largely arrested at the G0/G1 phase and showed fewer S-phase cells than did cells transfected with si-NC (Figure 2C and D). Flow cytometry analysis was also used to detect differences in apoptosis between cells transfected with si-TUG1 or si-NC. The percentage of apoptotic cells in the TUG1 knockdown group was 4.2-fold that in the controls (Figure 2E and F). These data indicated that TUG1 knockdown reduced proliferation, suppressed cell cycle progression, and promoted apoptosis of MCF-7 cells.
To elucidate the molecular mechanism of TUG1 action in MCF-7 cells, the relationship between TUG1 and miR-9 was evaluated. Using the starBase v2.0 database, miR-9 was found to potentially bind to TUG1 (Figure 3A). To explore the effect of TUG1 on the expression of miR-9, the level of miR-9 was measured in MCF-7 cells with ectopic expression of TUG1. In MCF-7 cells transfected with si-TUG1, the level of miR-9 was 4.3 times that in the control (Figure 3B). In contrast, miR-9 was significantly downregulated in cells with TUG1 overexpression (Figure 3C). Furthermore, the expression of TUG1 was decreased in MCF-7 cells transfected with the miR-9 mimic and increased in MCF-7 cells transfected with the miR-9 inhibitor (Figure 3D). To verify the relationship between TUG1 and miR-9, a dual luciferase reporter assay was performed by constructing a reporter containing the binding site in TUG1-WT or the mutant. The luciferase activity of the reporter containing TUG1-WT was reduced in cells transfected with the miR-9 mimic (Figure 3E). In addition, TUG1 expression was negatively correlated with the level of miR-9 in breast cancer tissues (Figure 3F).
To examine the effect of miR-9 combined with TUG1 knockdown on cell proliferation, cell cycle progression, and apoptosis, MCF-7 cells were divided into four groups: transfected with si-NC, transfected with si-TUG1, transfected with si-TUG1+miR-9 inhibitor, and transfected with miR-9 inhibitor. As shown in Figure 4A, the expression of miR-9 was downregulated in cells transfected with si-TUG1+miR-9 inhibitor. MiR-9 inhibition abrogated the reduction of cell proliferation induced by si-TUG1 (Figure 4B). Proliferation and cell cycle progression were significantly higher and the proportion of apoptotic cells was lower in MCF-7 cells transfected with miR-9 inhibitor than in MCF-7 cells transfected with si-TUG1+miR-9 inhibitor. In addition, miR-9 inhibition also reversed the effect of TUG1 on the cell cycle and cell apoptosis (Figure 4C-F).
As it has been reported that miR-9 may suppress proliferation of breast cancer cells by regulating the expression of MTHFD2, we examined the regulation of MTHFD2 by TUG1. As is shown in Figure 5A and B, the mRNA level and protein level of MTHFD2 were reduced in MCF-7 cells transfected with si-TUG1. The miR-9 inhibitor reversed the decrease in expression of MTHFD2 induced by si-TUG1. In breast cancer tissues, TUG1 expression was positively correlated with expression of MTHFD2 (Figure 5C).
To verify the effect of TUG1 on tumor growth in vivo, nude mice (five per group) were subcutaneously inoculated with MCF-7 breast cancer cells transfected with shRNA-TUG1 or shRNA-NC. The tumor was then imaged and the tumor volume was measured. As shown in Figure 6, TUG1 knockdown significantly suppressed tumor growth.
Recently, numerous studies have identified that aberrant expression of lncRNAs is closely linked with the initiation and development of cancer and has an influence on cancer prognosis; these findings have provided novel insights for cancer therapy [16]. Despite accumulating knowledge about the role and function of lncRNAs in biological processes and disease pathology, the molecular mechanism of lncRNAs in cancers has not yet been fully elucidated. To date, a variety of lncRNAs have been identified as potential biomarkers and prognostic targets in breast cancer, such as urothelial carcinoma associated 1 (UCA1), MALAT1 and growth-arrest-specific transcript 5 (GAS5) [171819]. Nevertheless, it is necessary to explore new targets and enrich our understanding of cancer pathology. The lncRNA TUG1 is upregulated in several cancer cells and tissues, including in colorectal cancer, gastric cancer, and osteosarcoma [202122]. However, its role in breast cancer remains unclear.
In the present study, we explored the function of TUG1 in breast cancer. Higher expression of TUG1 was observed in breast cancer tissues and cell lines than in the corresponding controls. As MCF-7 cells expressed the highest level of TUG1 amongst the cell lines studied, we studied the effect of TUG1 knockdown on MCF-7 cell proliferation and apoptosis. We observed that TUG1 knockdown reduced proliferation, suppressed cell cycle progression, and promoted apoptosis of breast cancer cells. These findings indicated that TUG1 might play a crucial role in promoting proliferation and inhibiting apoptosis of breast cancer cells. Our results are consistent with previous reports showing that TUG1 could act as an oncogene. Conversely, TUG1 is also a potential tumor suppressor in other cancers. For example, Li et al. [10] reported that TUG1 promoted apoptosis of glioma cells by activating intrinsic pathways and inhibiting antiapoptotic pathways.
It is well known that lncRNAs can serve as a “sponge” to regulate the expression of miRNAs [23]. From an online database, we found that TUG1 could potentially bind to miR-9. To verify this relationship, a dual luciferase reporter assay was used, and the result showed that luciferase activity of the reporter containing TUG1-WT was reduced in cells transfected with the miR-9 mimic. In addition, the expression of miR-9 was reduced in cells transfected with pcDNA-TUG1 and increased in cells transfected with si-TUG1. TUG1 expression was negatively correlated with the level of miR-9 in breast cancer tissues. Furthermore, miR-9 inhibition reversed the effect of TUG1 on the cell cycle progression and apoptosis.
Mounting evidence demonstrates that miR-9 plays important roles in breast cancer by targeting diverse genes. For example, Wang et al. [24] showed that overexpression of miR-9 in human breast cancer was associated with lymph node metastasis. MiR-9 was found to be upregulated in tumors with aggressive phenotypes, and its upregulated has been linked to the poor prognosis of breast cancer [15]. Zhou et al. [25] reported that higher expression of miR-9 is significantly associated with local recurrence of breast cancer in patients with a positive tumor estrogen receptor status. As one of the identified targets of miR-9, MTHFD2, regulated by miR-9, contributes to the anti-proliferative activity of miR-9 in breast cancer [26]. In this study, we examined the regulation of MTHFD2 by TUG1 and found that MTHFD2 expression was reduced in MCF-7 cells transfected with si-TUG1. In breast cancer tissue, TUG1 level was positively correlated with MTHFD2 expression. MTHFD2 was recently identified as a drug target that prevents cell migration and invasion of breast cancer [27]. Thus, the function of TUG1 in breast cancer might be associated with the regulation of miR-9 and MTHFD2.
We investigated the regulation of TUG1 and miR-9 in MDA-MB-231 cells. As shown in Supplementary Figure 1 (available online), the level of miR-9 was not significantly different in MDA-MB-231 cells transfected with either si-TUG1 or si-NC. Moreover, miR-9 mimics did not affect the level of TUG1 in MDA-MB-231 cells (Supplementary Figure 2, available online). These data indicate that TUG1 may not function in MDA-MB-231 cells through the regulation of miR-9. There are two possible explanations for these results. MDA-MB-231 cells originate from triple-negative breast cancer, while MCF-7 cells were derived from estrogen and progesterone receptor positive, human epidermal growth factor receptor negative breast cancer. In addition, MDA-MB-231 cells are p53-mutant breast cancer cells [28], while MCF-7 cells are p53 wildtype breast cancer cells. It has been reported that p53 plays a key role in regulating TUG1 expression, and TUG1 functions may be dependent on p53 status [29]. In this study, we found that TUG1 was also upregulated in MDA-MB-231 cells. As MDA-MB-231 cells are aggressive and invasive, TUG1 might be associated with these properties; however, this conclusion needs further investigation.
In summary, this study demonstrated that TUG1 is highly expressed in breast cancer tissues and cells. TUG1 knockdown was significantly associated with decreased proliferation and increased apoptosis of breast cancer cells. We also found that TUG1 promoted breast cancer cell proliferation by inhibiting miR-9. These data provide new insights into the function of TUG1 in the development of breast cancer.
Figures and Tables
Figure 1
Expression of taurine-upregulated gene 1 (TUG1) in breast cancer tissues and cell lines. (A) Expression of TUG1 in 24 breast cancer tissues and paired normal tissues detected by quantitative reverse transcription polymerase chain reaction (RT-qPCR) (n=24, each). (B) Expression of TUG1 in several breast cancer cell lines BT474, MCF-7, MDA-MB-231, T47D and normal mammary epithelial cell line 76N using RT-qPCR (n=6, each). Data represent mean±SD.
*p<0.05 vs. the corresponding control.
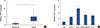
Figure 2
Effects of taurine-upregulated gene 1 (TUG1) on cell proliferation, cell cycle and cell apoptosis in MCF-7 cells. (A) The interference efficiency of si-TUG1, si-TUG1 2, and si-TUG1 3 on the expression of TUG1. (B) MTT assay showed that si-TUG1, si-TUG1 2, and si-TUG1 3 significantly reduced proliferation rate of MCF-7 cells. (C, D) si-TUG1 suppressed cell cycle progression in MCF-7 cells analyzed by flow cytometry. The bar chart represented the percentage of cells in G1–G0, S, or G2–M phase, as indicated. (E, F) TUG1 knockdown induced cell apoptosis of MCF-7 cells. Data represent mean±SD (n=6, each).
si-NC=control si-RNA; OD=optaical density.
*p<0.05 vs. si-NC.
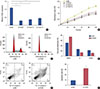
Figure 3
Taurine-upregulated gene 1 (TUG1) regulated the expression of miR-9. (A) Binding site was predicted between TUG1 and microRNA-9 (miR-9) according to starBase v2.0 database. (B) Effects of TUG1 knockdown on the expression of miR-9. (C) Effects of TUG1 overexpression on the expression of miR-9. (D) The expression of TUG1 in MCF-7 cells transfected with miR-9 mimic or inhibitor. (E) Luciferase activity of reporters containing TUG1-WT or TUG1-mut sequence in MCF-7 cells transfected with miR-9 mimic or control. (F) Correlation analysis was performed to detect the relationship between TUG1 and miR-9 in breast cancer tissues. Data represent mean±SD (n=6, each).
si-NC=control si-RNA; NC=negative control.
*p<0.05 versus the corresponding control.
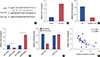
Figure 4
Effects of microRNA-9 (miR-9) inhibitor on the function of taurine-upregulated gene 1 (TUG1) on cell proliferation, cell cycle and cell apoptosis. MCF-7 cells were transfected with si-NC, si-TUG1, si-TUG1+miR-9 inhibitor and miR-9 inhibitor. (A) The expression of miR-9 in these cells. (B) MTT assay was performed to examine these cells' proliferation. (C, D) Cell cycle was detected in these cells. (E, F) Cell apoptosis was detected by flow cytometry. Data represent mean±SD (n=6, each).
si-NC=control si-RNA.
*p<0.05 vs. si-NC; †p<0.05 vs. si-TUG1; ‡p<0.05 vs. miR-9 inhibitor.
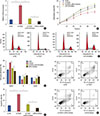
Figure 5
Effect of taurine-upregulated gene 1 (TUG1) on the expression of methylenetetrahydrofolate dehydrogenase 2 (MTHFD2) in breast cancer. (A) The mRNA level of MTHFD2 was detected in MCF-7 cells transfected with si-NC or si-TUG1 or si-TUG1+ microRNA-9 (miR-9) inhibitor (n=6, each). (B) The protein level of MTHFD2 was decreased in MCF-7 cells transfected with si-NC or si-TUG1 or si-TUG1+ miR-9 inhibitor (n=6, each). (C) TUG1 was positively correlated with MTHFD2 expression in breast cancer tissues (n=24, each). Data represent mean±SD.
si-NC=control si-RNA; GRPDH=glyceraldehyde-3-phosphate dehydrogenase.
*p<0.05 vs. si-NC; †p<0.05 vs. si-TUG1.
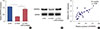
Figure 6
Effect of taurine-upregulated gene 1 (TUG1) on the tumor growth in vivo. (A) The tumor picture from mouse model of MCF-7 cells transfected with shRNA-TUG1 (n=3, each) or shRNA-NC (n=3, each). (B) The tumor volume in mouse model of MCF-7 cells transfected with shRNA-TUG1 (n=5, each) or shRNA-NC (n=5, each). Data represent mean±SD.
*p<0.05 vs. shRNA-NC.
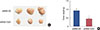
References
1. Yan S, Jiao X, Zou H, Li K. Prognostic significance of c-Met in breast cancer: a meta-analysis of 6010 cases. Diagn Pathol. 2015; 10:62.


2. O'Brien KM, Fei C, Sandler DP, Nichols HB, DeRoo LA, Weinberg CR. Hormone therapy and young-onset breast cancer. Am J Epidemiol. 2015; 181:799–807.
3. Shuvalov O, Petukhov A, Daks A, Fedorova O, Ermakov A, Melino G, et al. Current genome editing tools in gene therapy: new approaches to treat cancer. Curr Gene Ther. 2015; 15:511–529.


4. Gibb EA, Brown CJ, Lam WL. The functional role of long non-coding RNA in human carcinomas. Mol Cancer. 2011; 10:38.


5. Xing Z, Park PK, Lin C, Yang L. LncRNA BCAR4 wires up signaling transduction in breast cancer. RNA Biol. 2015; 12:681–689.


6. Jadaliha M, Zong X, Malakar P, Ray T, Singh DK, Freier SM, et al. Functional and prognostic significance of long non-coding RNA MALAT1 as a metastasis driver in ER negative lymph node negative breast cancer. Oncotarget. 2016; 7:40418–40436.


7. Ke H, Zhao L, Feng X, Xu H, Zou L, Yang Q, et al. NEAT1 is required for survival of breast cancer cells through FUS and miR-548. Gene Regul Syst Bio. 2016; 10:Suppl 1. 11–17.


8. Young TL, Matsuda T, Cepko CL. The noncoding RNA taurine upregulated gene 1 is required for differentiation of the murine retina. Curr Biol. 2005; 15:501–512.


9. Zhang Q, Geng PL, Yin P, Wang XL, Jia JP, Yao J. Down-regulation of long non-coding RNA TUG1 inhibits osteosarcoma cell proliferation and promotes apoptosis. Asian Pac J Cancer Prev. 2013; 14:2311–2315.


10. Li J, Zhang M, An G, Ma Q. LncRNA TUG1 acts as a tumor suppressor in human glioma by promoting cell apoptosis. Exp Biol Med (Maywood). 2016; 241:644–649.


11. Bartel DP. MicroRNAs: genomics, biogenesis, mechanism, and function. Cell. 2004; 116:281–297.
12. Krichevsky AM, King KS, Donahue CP, Khrapko K, Kosik KS. A microRNA array reveals extensive regulation of microRNAs during brain development. RNA. 2003; 9:1274–1281.


13. Lu J, Luo H, Liu X, Peng Y, Zhang B, Wang L, et al. miR-9 targets CXCR4 and functions as a potential tumor suppressor in nasopharyngeal carcinoma. Carcinogenesis. 2014; 35:554–563.


14. Wang H, Zhang W, Zuo Y, Ding M, Ke C, Yan R, et al. miR-9 promotes cell proliferation and inhibits apoptosis by targeting LASS2 in bladder cancer. Tumour Biol. 2015; 36:9631–9640.


15. Gwak JM, Kim HJ, Kim EJ, Chung YR, Yun S, Seo AN, et al. MicroRNA-9 is associated with epithelial-mesenchymal transition, breast cancer stem cell phenotype, and tumor progression in breast cancer. Breast Cancer Res Treat. 2014; 147:39–49.


16. Im JH, Muschel RJ. New evidence of lncRNA role in tumor progression and metastasis. Hepatobiliary Surg Nutr. 2012; 1:55–56.
17. Tuo YL, Li XM, Luo J. Long noncoding RNA UCA1 modulates breast cancer cell growth and apoptosis through decreasing tumor suppressive miR-143. Eur Rev Med Pharmacol Sci. 2015; 19:3403–3411.
18. Xu S, Sui S, Zhang J, Bai N, Shi Q, Zhang G, et al. Downregulation of long noncoding RNA MALAT1 induces epithelial-to-mesenchymal transition via the PI3K-AKT pathway in breast cancer. Int J Clin Exp Pathol. 2015; 8:4881–4891.
19. Han L, Ma P, Liu SM, Zhou X. Circulating long noncoding RNA GAS5 as a potential biomarker in breast cancer for assessing the surgical effects. Tumour Biol. 2016; 37:6847–6854.


20. Sun J, Ding C, Yang Z, Liu T, Zhang X, Zhao C, et al. The long non-coding RNA TUG1 indicates a poor prognosis for colorectal cancer and promotes metastasis by affecting epithelial-mesenchymal transition. J Transl Med. 2016; 14:42.


21. Zhang E, He X, Yin D, Han L, Qiu M, Xu T, et al. Increased expression of long noncoding RNA TUG1 predicts a poor prognosis of gastric cancer and regulates cell proliferation by epigenetically silencing of p57. Cell Death Dis. 2016; 7:e2109.


22. Ma B, Li M, Zhang L, Huang M, Lei JB, Fu GH, et al. Upregulation of long non-coding RNA TUG1 correlates with poor prognosis and disease status in osteosarcoma. Tumour Biol. 2016; 37:4445–4455.


23. Kallen AN, Zhou XB, Xu J, Qiao C, Ma J, Yan L, et al. The imprinted H19 lncRNA antagonizes let-7 microRNAs. Mol Cell. 2013; 52:101–112.


24. Wang J, Zhao H, Tang D, Wu J, Yao G, Zhang Q. Overexpressions of microRNA-9 and microRNA-200c in human breast cancers are associated with lymph node metastasis. Cancer Biother Radiopharm. 2013; 28:283–288.


25. Zhou X, Marian C, Makambi KH, Kosti O, Kallakury BV, Loffredo CA, et al. MicroRNA-9 as potential biomarker for breast cancer local recurrence and tumor estrogen receptor status. PLoS One. 2012; 7:e39011.


26. Selcuklu SD, Donoghue MT, Rehmet K, de Souza Gomes M, Fort A, Kovvuru P, et al. MicroRNA-9 inhibition of cell proliferation and identification of novel miR-9 targets by transcriptome profiling in breast cancer cells. J Biol Chem. 2012; 287:29516–29528.


27. Liu F, Liu Y, He C, Tao L, He X, Song H, et al. Increased MTHFD2 expression is associated with poor prognosis in breast cancer. Tumour Biol. 2014; 35:8685–8690.

