Abstract
Purpose
Patients with triple-negative breast cancer (TNBC) with pathologic complete response (pCR) to neoadjuvant chemotherapy (NAC) have superior survival outcomes compared to those with residual disease after NAC. This study investigated the value of three biomarkers, p53, Ki-67, and Bcl-2 for predicting pCR in NAC-treated patients with TNBC.
Methods
Between 2003 and 2012, 198 patients with pathologically confirmed primary TNBC were treated with two different taxane-based chemotherapeutic regimens prior to surgery. Before NAC, expression of p53 (cutoff 25%), Ki-67 (cutoff 10%), and Bcl-2 (cutoff 10%) was assessed immunohistochemically in core biopsy specimens. The incidence of pCR was correlated with the expression of these biomarkers.
Results
Overall, pCR occurred in 37 of the 198 patients (18.7%). A significant association was observed between the pCR rate and overexpression of the p53 and Ki-67 biomarkers. Multivariate analysis showed that only p53 expression was independently associated with pCR to NAC (odds ratio, 3.961; p=0.003). The sensitivity, specificity, positive predictive value, and negative predictive value of p53 expression for predicting pCR were 77.8%, 50.3%, 26.2%, and 90.9%, respectively. The pCR rate was the lowest (5.2%) in patients with low expression of both p53 and Ki-67, and it was the highest (25.8%) when both biomarkers showed high expression.
Triple-negative breast cancer (TNBC) has been associated with poor clinical outcomes [1]. To date, no molecular targeted therapy has proven effective in TNBC. Higher rates of pathologic complete response (pCR) have been observed in patients with TNBC than in those with other types of breast cancer, and TNBC patients who achieve pCR have excellent survival outcomes. In contrast, TNBC patients who have residual disease after neoadjuvant chemotherapy (NAC) have significantly poorer survival outcomes than patients without TNBC [2]. As TNBC includes NAC-sensitive and NAC-resistant subgroups that have different survival outcomes, the ability to predict patients who will achieve pCR or residual tumor after NAC may offer clinical advantages in the management of these patients.
The tumor proliferation marker Ki-67 has been associated with response to chemotherapy. Although high Ki-67 expression was found to correlate with increased rates of clinical response and pCR following NAC [3,4], the association between Ki-67 expression and outcomes in patients with TNBC has not been determined, and Ki-67 has not been compared with other putative biomarkers in the same patient cohorts.
Bcl-2 has been found to inhibit apoptosis induced by chemotherapeutic drugs, including doxorubicin, in cancer cells [5]. However, the significance of Bcl-2 expression in predicting sensitivity to chemotherapy has not been determined.
The tumor suppressor protein p53 (TP53) has many functions in multicellular organisms, including activating DNA repair proteins, regulating the cell cycle, and initiating apoptosis. In vitro studies in tumor cell lines treated with chemotherapy agents have shown that p53 is required for the efficient activation of apoptosis, suggesting that loss of p53 may enhance cellular resistance to chemotherapeutic agents. Several studies have reported that patients with p53 mutations showed poorer responses to chemotherapy than those with wild-type p53 status [6], whereas other studies have reported conflicting results [7,8]. The expression of p53 has not been successful as a predictive marker for response to NAC.
This study investigated the value of p53, Ki-67, and Bcl-2 expression for predicting pCR in NAC-treated patients with TNBC.
The study cohort consisted of 198 TNBC patients, with a median age of 42 years (range, 24-71 years), who received NAC between January 2003 and September 2012, had known pathologic and clinical tumor responses, and from whom biopsy material was available for pathological evaluation. pCR was defined as the complete disappearance of invasive carcinoma cells in the breast and axillary lymph nodes after NAC (ypT0/is, ypN0). Patients with residual ductal carcinoma in situ were considered to have achieved pCR.
Eighty-two patients were treated with docetaxel (75 mg/m2 or 60 mg/m2) and doxorubicin (60 mg/m2 or 50 mg/m2) by intravenous infusion every 3 weeks for three cycles, followed by primary surgery and an additional three cycles of docetaxel and doxorubicin, and, if indicated, radiation therapy. An add-itional 74 patients received six cycles of neoadjuvant docetaxel and doxorubicin. Another 42 patients received four cycles of AC (doxorubicin 60 mg/m2 or 50 mg/m2, cyclophosphamide 500 mg/m2 on day 1, every 21 days), followed by four cycles of docetaxel (75 mg/m2 or 60 mg/m2). Four patients who showed progressive disease during NAC received adjuvant chemotherapy, consisting of FAC (fluorouracil, doxorubicin, and cyclophosphamide) or CMF (cyclophosphamide, methotrexate, and fluorouracil) after curative surgery. Patients underwent either mastectomy or breast-conserving surgery at the discretion of the surgeon. Sentinel lymph node biopsies were performed unless contraindicated. If radiotherapy was indicated, it was administered after surgery.
This retrospective study protocol was reviewed and approved by the Institutional Review Board (approval number: 1003-020-311) of the Seoul National University Hospital. Because this study was performed using a total of 198 consecutive patients in our database, and involved no more than minimal risk for the subjects, the Institutional Review Board approved our request for the waiver of informed consent. This study followed the recommendations of the Declaration of Helsinki for biomedical research involving human subjects.
Pretreatment biopsy material was used for immunohistochemistry (IHC) analyses to avoid any effects of chemotherapy on the expression levels of the proteins. Pretreatment formalin-fixed, paraffin-embedded tissue blocks were used for IHC. Estrogen receptor (ER), progesterone receptor (PR), human epidermal growth factor receptor 2 (HER2), p53, Bcl-2, and Ki-67 expression levels were evaluated by the avidin-biotin complex technique for IHC [6]. Tissue sections (4 µm thick) were cut from paraffin-embedded tissue blocks, deparaffinized in xylene, rehydrated with graded ethanol, and immersed in Tris-buffered saline. After antigen retrieval, the sections were incubated with primary antibodies as described [9]. Mouse monoclonal primary antibodies to ER (1:50), PR (1:50), p53 (1:1,200), Bcl-2 (1:50), and Ki-67 (1:800) were obtained from Dako Corp. (Carpinteria, USA), whereas antibody to HER2 (1:200) was obtained from Novocastra Laboratories Ltd. (New-castle-Upon-Tyne, UK). Sections were subsequently incubated with biotinylated antimouse IgG antibody and streptavidin horseradish peroxidase (Zymed Laboratories, San Francisco, USA). High expression of Ki-67 was defined as expression in ≥10% of tumor tissues, as the 10% cutoff provided the best predictive value for prognosis in our institution [10]. Bcl-2 expression was judged positive when ≥10% of the nuclei and cytoplasm of tumor cells were stained. Pathological records in our institution are designated as either positive or negative for Bcl-2 expression. IHC positivity in ≥25% of the tumor cells was considered high expression. This value was almost equivalent to the cutoff provided by a previous study in our institution [1]. Tumors were regarded as negative for ER and PR expression when <1% of the tumor cells showed nuclear staining. HER2 was considered negative when the HER2 score was 0 or 1+ upon IHC, or when the HER2 score was 2+ and HER2/CEP17 gene amplification was negative (as assessed by using fluorescence in situ hybridization). TNBCs were defined as ER-negative, PR-negative, and HER2-negative breast cancers.
The associations between the clinicopathological characteristics and pathological response after NAC were calculated using the chi-squared and Fisher exact tests. We used a stepwise regression model to approximate the risk ratio of achieving pCR in multiple regression analyses according to the baseline factors. Disease-free survival (DFS) was defined as the interval from the date of operation to the date when disease relapse or progression was first documented. Kaplan-Meier curves were generated to evaluate the DFS. All values were two-sided, and statistical significance was defined as p<0.05. SPSS version 19.0 software (IBM Corp., Armonk, USA) was used for all statistical analyses.
Of the 198 patients, 123 (62.1%) presented with clinical stage III at the time of initial diagnosis and 63 (31.8%) presented with clinical stage IIB. Median tumor size was 4.3 cm (range, 0.8-9.8 cm), and 132 women (66.7%) underwent breast-conserving surgery. The relationships between pretreatment clinicopathological characteristics and expression levels of p53, Ki-67, and Bcl-2 are shown in Table 1. None of these clinicopathological characteristics showed significantly different distributions according to the levels of these three biomarkers.
After NAC, the complete absence of residual invasive carcinoma cells in the breast was confirmed by histological examination in 42 of the 198 patients (21.2%); however, five of these 42 patients had residual carcinoma cells in the resected lymph nodes. Therefore, 37 of the 198 patients (18.7%) achieved pCR. Of the clinicopathological factors assessed, the initial tumor size was found to be significantly associated with pCR, whereas overexpression of p53 and Ki-67 (Table 2, Figure 1), and high nuclear grade indicated a high likelihood of achieving pCR (Table 2). The pCR rate was significantly higher in patients with high p53 expression (≥25%) than in those with low p53 expression (<25%) (27.1% vs. 6.8%, p<0.001), and it was almost significantly higher in patients with high Ki-67 expression (≥10%) than in those with low Ki-67 expression (<10%) (19.5% vs. 5.9%, p=0.056). In contrast, the level of expression of Bcl-2 was not significantly associated with the pCR rate. The sensitivity, specificity, positive predictive value, and negative predictive value of p53 expression for predicting pCR were 77.8%, 50.3%, 26.2%, and 90.9%, respectively. When p53 and Ki-67 were analyzed in conjunction, the pCR rate was the lowest (5.2%) in patients with low levels of both biomarkers, and it was the highest (25.8%) in patients with high levels of both (Figure 2). Considering the independent significant factors and the clinically important chemotherapy regimens, multiple regression analysis showed that p53 expression was the only independent factor associated with pCR (odds ratio, 3.961; p=0.003) (Table 3). The group that achieved pCR tended to show superior DFS than the group that failed to achieve pCR (Figure 3A). However, expression of p53 alone could not predict pCR prognosis accurately (Figure 3B).
Our finding that the degree of p53 expression in TNBCs was strongly associated with increased response to NAC is in agreement with the results of several previous studies [11,12,13,14,15,16]. A meta-analysis of 26 studies also demonstrated the effectiveness of p53 expression for predicting the response to NAC in breast cancer [17]. Although that study found that increased expression of TP53 was associated with higher objective response and complete response rates, it had innate limitations, including different measurements of TP53 expression (protein or mRNA) and different cutoff values for TP53 overexpression by IHC and those obtained by gene amplification. Moreover, studies analyzing the predictive value of p53 were heterogeneous in patient characteristics, breast cancer subtypes, and chemotherapy regimens. Many of these clinical reports involved small cohorts, with inconsistent methods of measuring p53 expression. Moreover, most of these studies did not distinguish among patients with breast cancer subtypes, and study populations were treated with heterogeneous chemotherapeutic regimens.
Mutations in the TP53 gene have been observed more frequently in TNBCs than in other breast cancer subtypes. For instance, the frequency of TP53 mutations was found to be higher in basal than in luminal type tumors [18]. Several studies have shown that p53 is a marker of a more favorable response to NAC in patients with TNBC. For example, p53 overexpression was strongly correlated with pCR rate in TNBCs [19], and mutant TP53 was identified as a major independent predictive factor of pCR in patients treated with anthracycline-based NAC [20].
In assessing tumor markers in breast cancer patients, we limited the study subjects to patients with the molecular TNBC subtype that responded to taxane-based NAC, thus avoiding any effects of a heterogeneous patient population. Our findings are therefore more reliable, in that p53 is predictive of pCR in patients with TNBC treated with taxane-based NAC.
Positive Bcl-2 expression has been associated with poor survival and reduced sensitivity to chemotherapy in patients with TNBC [21,22]. Although we found that Bcl-2 expression was not significantly associated with pCR in TNBC patients, patients belonging to the Bcl-2-negative group tended to be more chemosensitive than those belonging to the Bcl-2-positive group. This finding is in agreement with previous results showing that Bcl-2 could not predict response to NAC [23].
Univariate analysis showed an almost significant association between Ki-67 expression and pCR, consistent with the results of our previous study [15]. In multiple regression analysis, while Ki-67 could not be identified as an independent predictor of pCR, the combination of p53 and Ki-67 had greater predictive accuracy than p53 alone. No association has been reported between high Ki-67 expression and response rate [11,24], although high expression of Ki-67 was associated with higher response rate to NAC. The role of Ki-67 is not yet conclusive because of heterogeneous patient populations, small sample sizes, and different chemotherapeutic regimens in previous studies. In conclusion, this study was designed to assess whether the IHC markers p53, Ki-67, and Bcl-2 could predict the response to NAC in patients with TNBC. Our results indicate that the tumors with high p53 expression may respond better to taxane-based NAC. Measurement of these markers before starting NAC may assist in making treatment decisions and in predicting response to treatment.
Figures and Tables
![]() | Figure 1Pathologic complete response (pCR) rate according to p53, Ki-67, and Bcl-2 expression in triple-negative breast cancer (n=198). |
![]() | Figure 2Pathologic complete response (pCR) rate according to the combination of p53 and Ki-67 expression. |
![]() | Figure 3(A) Disease-free survival (DFS) according to pathologic complete response (pCR) and non-pCR. (B) DFS according to the combination of p53 and pCR. |
Table 1
Prechemotherapy clinicopathological characteristics
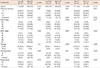
Table 2
Correlations between pathologic response and clinicopathological characteristics
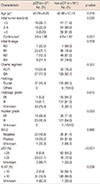
Table 3
Multiple logistic regression analysis for factors affecting pathologic complete response in triple-negative breast cancer
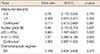
Notes
This work was presented at the 2012 San Antonio Breast Cancer Symposium on December 4-8, 2012 as a poster presentation.
References
1. Keam B, Im SA, Kim HJ, Oh DY, Kim JH, Lee SH, et al. Prognostic impact of clinicopathologic parameters in stage II/III breast cancer treated with neoadjuvant docetaxel and doxorubicin chemotherapy: paradoxical features of the triple negative breast cancer. BMC Cancer. 2007; 7:203.


2. Liedtke C, Mazouni C, Hess KR, André F, Tordai A, Mejia JA, et al. Response to neoadjuvant therapy and long-term survival in patients with triple-negative breast cancer. J Clin Oncol. 2008; 26:1275–1281.


3. Bozzetti C, Musolino A, Camisa R, Bisagni G, Flora M, Bassano C, et al. Evaluation of HER-2/neu amplification and other biological markers as predictors of response to neoadjuvant anthracycline-based chemotherapy in primary breast cancer: the role of anthracycline dose intensity. Am J Clin Oncol. 2006; 29:171–177.


4. Penault-Llorca F, Abrial C, Raoelfils I, Chollet P, Cayre A, Mouret-Reynier MA, et al. Changes and predictive and prognostic value of the mitotic index, Ki-67, cyclin D1, and cyclo-oxygenase-2 in 710 operable breast cancer patients treated with neoadjuvant chemotherapy. Oncologist. 2008; 13:1235–1245.


5. Ohmori T, Podack ER, Nishio K, Takahashi M, Miyahara Y, Takeda Y, et al. Apoptosis of lung cancer cells caused by some anti-cancer agents (MMC, CPT-11, ADM) is inhibited by bcl-2. Biochem Biophys Res Commun. 1993; 192:30–36.


6. Tiezzi DG, Andrade JM, Ribeiro-Silva A, Zola FE, Marana HR, Tiezzi MG. HER-2, p53, p21 and hormonal receptors proteins expression as predictive factors of response and prognosis in locally advanced breast cancer treated with neoadjuvant docetaxel plus epirubicin combination. BMC Cancer. 2007; 7:36.


7. Anelli A, Brentani RR, Gadelha AP, Amorim De Albuquerque A, Soares F. Correlation of p53 status with outcome of neoadjuvant chemotherapy using paclitaxel and doxorubicin in stage IIIB breast cancer. Ann Oncol. 2003; 14:428–432.


8. Kandioler-Eckersberger D, Ludwig C, Rudas M, Kappel S, Janschek E, Wenzel C, et al. TP53 mutation and p53 overexpression for prediction of response to neoadjuvant treatment in breast cancer patients. Clin Cancer Res. 2000; 6:50–56.
9. Yonemori K, Tsuta K, Shimizu C, Hatanaka Y, Hashizume K, Ono M, et al. Immunohistochemical expression of PTEN and phosphorylated Akt are not correlated with clinical outcome in breast cancer patients treated with trastuzumab-containing neo-adjuvant chemotherapy. Med Oncol. 2009; 26:344–349.


10. Jung SY, Han W, Lee JW, Ko E, Kim E, Yu JH, et al. Ki-67 expression gives additional prognostic information on St. Gallen 2007 and Adjuvant! Online risk categories in early breast cancer. Ann Surg Oncol. 2009; 16:1112–1121.


11. von Minckwitz G, Sinn HP, Raab G, Loibl S, Blohmer JU, Eidtmann H, et al. Clinical response after two cycles compared to HER2, Ki-67, p53, and bcl-2 in independently predicting a pathological complete response after preoperative chemotherapy in patients with operable carcinoma of the breast. Breast Cancer Res. 2008; 10:R30.


12. Bottini A, Berruti A, Bersiga A, Brizzi MP, Brunelli A, Gorzegno G, et al. p53 but not bcl-2 immunostaining is predictive of poor clinical complete response to primary chemotherapy in breast cancer patients. Clin Cancer Res. 2000; 6:2751–2758.
13. Mieog JS, van der Hage JA, van de Vijuer MJ, van de Velde CJ. Cooperating Investigators of the EORTC. Tumour response to preoperative anthracycline-based chemotherapy in operable breast cancer: the predictive role of p53 expression. Eur J Cancer. 2006; 42:1369–1379.


14. Tan DS, Marchió C, Jones RL, Savage K, Smith IE, Dowsett M, et al. Triple negative breast cancer: molecular profiling and prognostic impact in adjuvant anthracycline-treated patients. Breast Cancer Res Treat. 2008; 111:27–44.


15. Keam B, Im SA, Lee KH, Han SW, Oh DY, Kim JH, et al. Ki-67 can be used for further classification of triple negative breast cancer into two subtypes with different response and prognosis. Breast Cancer Res. 2011; 13:R22.


16. Carey LA, Dees EC, Sawyer L, Gatti L, Moore DT, Collichio F, et al. The triple negative paradox: primary tumor chemosensitivity of breast cancer subtypes. Clin Cancer Res. 2007; 13:2329–2334.


17. Chen MB, Zhu YQ, Xu JY, Wang LQ, Liu CY, Ji ZY, et al. Value of TP53 status for predicting response to neoadjuvant chemotherapy in breast cancer: a meta-analysis. PLoS One. 2012; 7:e39655.


18. Dumay A, Feugeas JP, Wittmer E, Lehmann-Che J, Bertheau P, Espié M, et al. Distinct tumor protein p53 mutants in breast cancer subgroups. Int J Cancer. 2013; 132:1227–1231.
19. Sakuma K, Kurosumi M, Oba H, Kobayashi Y, Takei H, Inoue K, et al. Pathological tumor response to neoadjuvant chemotherapy using anthracycline and taxanes in patients with triple-negative breast cancer. Exp Ther Med. 2011; 2:257–264.


20. Bertheau P, Espié M, Turpin E, Lehmann J, Plassa LF, Varna M, et al. TP53 status and response to chemotherapy in breast cancer. Pathobiology. 2008; 132–139.
21. Wang TT, Phang JM. Effects of estrogen on apoptotic pathways in human breast cancer cell line MCF-7. Cancer Res. 1995; 55:2487–2489.
22. Gasparini G, Barbareschi M, Doglioni C, Palma PD, Mauri FA, Boracchi P, et al. Expression of bcl-2 protein predicts efficacy of adjuvant treatments in operable node-positive breast cancer. Clin Cancer Res. 1995; 1:189–198.