Abstract
Purpose
This study was performed to assess frequency, timings of occurrence, and predictors of radiologic lung damage (RLD) after forward-planned intensity-modulated radiotherapy (FIMRT) for whole breast irradiation.
Methods
We retrospectively reviewed medical records of 157 breast cancer patients and each of their serial chest computed tomography (CT) taken 4, 10, 16, and 22 months after completion of breast radiotherapy (RT). FIMRT was administered to whole breast only (n=152), or whole breast and supraclavicular regions (n=5). Dosimetric parameters, such as mean lung dose and lung volume receiving more than 10 to 50 Gy (V10-V50), and clinical parameters were analyzed in relation to radiologic lung damage.
Results
In total, 104 patients (66.2%) developed RLD after whole breast FIMRT. Among the cases of RLD, 84.7% were detected at 4 months, and 15.3% at 10 months after completion of RT. More patients of 47 or younger were found to have RLD at 10 months after RT than patients older than the age (11.7% vs. 2.9%, p=0.01). In univariate and multivariate analyses, age >47 and V40 >7.2% were significant predictors for higher risk of RLD.
Conclusion
RLD were not infrequently detected in follow-up CT after whole breast FIMRT. More detected cases of RLD among younger patients are believed to have developed at later points after RT than those of older patients. Age and V40 were significant predictors for RLD after whole breast intensity-modulated radiotherapy.
Adjuvant radiotherapy (RT) after breast-conserving surgery (BCS) reduces disease recurrence and cancer death in patients with localized breast cancer [1]. Nonetheless, radiation can cause injury to organs surrounding breast, mainly to ipsilateral lung. Symptomatic or radiographic lung injury after conventional breast RT develops not infrequently with the incidence ranging between 5.5% and 100% according to previous reports [2,3,4,5,6,7,8,9].
For the last decade, intensity-modulated radiotherapy (IMRT) after BCS has been in use to reduce radiation-related toxicities. IMRT improves dose homogeneity throughout the targeted breast, and significantly decrease skin injury when compared to conventional radiation techniques [10]. Meanwhile, there have been a few studies that reported reduced lung volume irradiated by IMRT compared to conventional RT in breast cancer [11]. Most of the studies focused on analyzing dosimetric effects of IMRT on irradiated lung volume rather than evaluating clinical effects on lung injuries in patients with breast cancer. Few studies have reported on radiologic or clinical pulmonary toxicity after IMRT for breast cancer.
The present study was performed to assess frequency, timings of occurrence, and predictors of pulmonary toxicity after forward-planned intensity-modulated radiotherapy (FIMRT) for whole breast irradiation.
Between January 2006 to August 2009, 507 patients were treated with postoperative RT for stage 0 to III breast cancer at the Department of Radiation Oncology, Hallym University Sacred Heart Hospital. Our policy on breast RT specifies as standard tangential beam RT for patients with modified radical mastectomy, and FIMRT for those with BCS. A total of 323 patients received FIMRT for breast cancer during the period. Inclusion criteria of the current study were as follows: 1) completion of RT per plan; 2) follow-ups with serial chest computed tomography (CT) scans after completion of RT; 3) More than 12 months of follow-up periods. A total of 166 cases were ineligible for the present analysis due to the following reasons: 1) For 109 patients who had surgery and clinical follow-ups at outside hospitals, relevant data were unavailable; 2) Dose-volume histogram data were absent in 55 cases due to technical problems in radiation treatment planning system; 3) And lastly, radiation therapies were not completed on two patients as initially planned. Accordingly, 157 patients were deemed eligible for the present analysis.
The median age of the patients was 47 years old. Supraclavicular lymph nodes were irradiated in five patients. A total of 108 patients were treated with postoperative adjuvant chemotherapy with regimens consisted of taxane-based multi-agent chemotherapy, or 5-fluorouracil, epirubicin, and cyclophosphamide (FEC), or 5-fluorouracil, doxorubicin, and cyclophosphamide (FAC) before RT. RT was started median 21 days (range, 9-113) after completion of chemotherapy. Tamoxifen or aromatase-inhibitors was prescribed to 119 patients for 2 to 5 years, and the medication was concurrently administered with RT for 52 patients. The characteristics of the analyzed patients are summarized in Table 1. The current study was reviewed and approved by the Institutional Review Board of Hallym University Sacred Heart Hospital (approval number, 2014-I010).
CT scans for RT planning were performed for each patient. A set of transverse images of CT were transferred to a Pinnacle treatment-planning workstation (ADAC Laboratories, Milpitas, USA). Target volume consisted of remnant breast for patients with less than four lymph nodes metastasis, and supraclavicular lymph nodes along with the remnant breast for patients with four or more lymph nodes metastasis.
In all patients, FIMRT was applied. The superior, inferior, and lateral borders of the beams were 2 cm beyond the palpable breast tissue. The medial edges were located at midline, and the superficial borders of beams allowed 2 cm of flash beyond the breast. The prescription dose to whole breast was 50.4 Gy to calculation point with a daily dose of 1.8 Gy. For the treatment planning, the dose distribution was calculated with density correction for open tangential fields. Three-dimensional isodose surfaces ranging from the 100% isodose surface in 3% increments were visualized in the beams-eye view. Multileaf collimator (MLC) segments were formed for each of the isodose surfaces in ascending order from the 100% isodose surface. Typically, seven MLC segments were constructed for each tangential beam. Three monitor unit was allocated to each segment, and the dose of the open tangential beam was manually adjusted to deliver homogenous dose of 50.4 Gy throughout the breast.
For patients who need supraclavicular lymph nodes irradiation, three-field RT was administered with a single isocenter and asymmetric collimation. The superior jaws of the tangents were matched to the inferior jaw of the supraclavicular field. The supraclavicular field was treated with single field photon beam, and breast fields were treated with IMRT as abovementioned method. If a boost was required, dose of 10.8 Gy in six fractions was administered with electron beam to the surgical bed.
For dosimetric analysis, each lung was outlined from the apex to the base. Mean dose to lung (MLD), and the percentage of ipsilateral lung volumes receiving more than 10, 20, 30, 40, and 50 Gy (V10-50) were calculated. Furthermore, central lung distance (CLD) that was measured from the posterior tangential field edge to the posterior part of the chest wall at the center of the field was evaluated on the individual plans.
All patients were followed with chest CT scans first at 4 months after the completion of RT, and were seen with CT scans at every 6 month interval thereafter. History taking and physical examination were performed to assess symptomatic lung toxicity. To evaluate lung toxicity, we examined four sets of the CT scans that were taken 4, 10, 16, and 22 months after the completion of RT for each patient. Radiologic lung damage (RLD) at each CT scans were evaluated by comparing them with planning CT scan that was taken before RT. The RLD was graded according to the scoring system of Nishioka et al. [12]; grade 0, no significant changes in the radiation fields; grade 1, only pleural thickening in the radiation fields; grade 2, pulmonary changes (plaque-like or heterogenous density) in ≤50% area of radiation fields; and grade 3, pulmonary changes (plaque-like or heterogenous density) in >50% area of radiation fields.
The relation between RLD and clinical and dosimetric factors was analyzed with logistic regression model. Clinical factors such as patient's age, medication of taxane, concurrent administration of tamoxifen during RT, and interval between chemotherapy and RT were considered as binary variables. The CLD, MLD, V10-50 were analyzed as continuous variables. Optimal cutoff point of the each variable was defined as a value that had lowest p-value by logistic regression analysis. Calculation of the area under the curve (AUC) of receiver operating characteristics (ROC) was used to assess the most predictive dosimetric parameter for RLD. As the AUC of ROC value gets closer to 1.0, the parameter becomes more predictive of lung damage. All clinical parameters and the most predictive dosimetric parameter were evaluated by multivariate logistic regression analysis. Time to occurrence of RLD was estimated using the Kaplan-Meier method. Comparisons of factors between the patients with symptomatic lung toxicity and the patients without such symptoms were performed by Mann-Whitney test and Fisher exact test. Statistical significance was calculated at the 95% confidence interval (p<0.05) and all analysis was performed using SAS version 9.1 (SAS Institute, Cary, USA).
The median follow-up time of patients was 68 months (range, 12-99 months). All patients except three were undertaken four sets of CT scans at 4, 10, 16, and 22 months after RT. One patient had two sets of CT and two patients had three sets of CT. All of the three patients had CT scans 4 and 10 months after RT. The reasons for undertaking less number of follow-up CTs were due to cancer recurrence in two patients, and a refusal of CT by 1. In total, 624 sets of chest CT scans of the 157 patients were reviewed. RLD was found in 104 patients (66.2%), including 77 patients with grade 1, 24 patients with grade 2, and three patients with grade 3. The alterations in grades of RLD were consecutively evaluated with follow-up chest CT scans. Fifty-six patients (53.8%) showed constant grade, 45 (44.2%) had decreasing grade, and 3 (2.0%) had increasing followed by decreasing grade of RLD.
To determine the most predictive dosimetric factor for development of RLD, logistic regression analysis and calculation of AUCs of ROC were performed. All dosimetric factors such that CLD, MLD, and V10-50 were predictive for RLD with statistical significances in univariate analysis. However, only V40 was significant factor for RLD in multivariate analysis. According to the ROC analysis, V40 was the most predictive factor for development of RLD (AUC of ROC, 0.710), and the proper cutoff point was 7.2% (Table 2). The results of univariate and multivariate analyses of the dosimetric or clinical parameters in relation to RLD are summarized in Table 3. Age and V40 were significant predictors for RLD in univariate and multivariate analyses. Probabilities of RLD according to age and V40 were depicted in Figure 1.
Among the 104 patients who showed RLD, 89 patients were found to have RLD at 4 months after RT and 15 patients showed RLD at 10 months after RT. Patients younger than 47 were more likely to show RLD at 10 months after RT than patients older than 47, with statistical significance (Table 4).
Four patients (2.5%) developed symptomatic lung toxicity. Symptoms for the four patients were treated with conservative management such as antitussive or nonsteroidal anti-inflammatory drugs. No patient needed steroid treatment. Factors associated with symptomatic lung toxicity were shown in Table 5. Median value of CLD was significantly different between the patients with pulmonary symptom and the patients without it.
RLD was found in 66.2% of the patients and symptomatic lung toxicity was detected in 2.5% of the patients after whole breast FIMRT in the current study. In the previous studies of conventional breast RT, the incidence of RLD was reported to be in the range of 37.0% to 100.0% by chest CT assessment [2,3,7,8,9]. Number of patients, target of RT (remnant breast vs. chest wall), fields of irradiation (local only vs. locoregional), and timing of follow-up chest CT were different in the studies. Although it is difficult to directly compare the current investigation with the previous studies of conventional breast RT, the proportion of patients with RLD in the present study is similar to that of the other reports in conventional RT. Tangential beam IMRT can reduce the irradiated lung volume more than conventional whole breast RT [11,13]. However, this dosimetric effect by IMRT does not seem to have resulted in fewer incidence of RLD than the conventional RT. Tangential beam arrangement itself in both conventional RT and IMRT helps the irradiated lung volume become small in whole breast irradiation. Moreover, IMRT with confined beams by tangential field is limited in reducing radiation does to ipsilateral lung. Thus, RLD might not be remarkably reduced by tangential beam IMRT compared to conventional RT in whole breast irradiation as shown in the current study. Nonetheless, randomized comparison of lung damage between conventional method and IMRT is warrant to ascertain the influences of IMRT on lung toxicity in breast cancer.
Patients' age and V40 were important predictors for RLD after whole breast irradiation in this study. The significance of age was also demonstrated in the studies of conventional whole breast irradiation [7,8,9,14]. Older age were related to higher probability of lung injury after breast RT. In the previous studies, the reference ages dividing the incidence of RLD into two groups varied between 58 and 59. Meanwhile, the reference age was 47 in the current study. The discrepancy in the reference ages of RLD between the current and the previous reports is likely attributable to the median ages of study populations. The median ages of patients in the previous studies were about 10 years older than those in the present study. This is in accordance with the fact that the median age of South Korean breast cancer patients is 49 years old which is over ten years younger than that of its Caucasian counterparts [15]. Nonetheless, the probability of RLD was higher among older patients in the current study as in the previous reports.
Radiation dose to lung and volume of lung irradiated are considered as significant predictors for RLD after breast irradiation [4,7,8]. It has been suggested in the studies of conventional breast irradiation that V20 [4], V13, V20 and V30 [16], V25 [8], or MLD, V20, and the radiation dose to 25% of the ipsilateral lung [7] are important factors in predicting lung injury. Similar to these studies, variables such as V20, V30, and MLD showed significant impact on RLD in our univariate analyses. However, V40 was only significant factor in multivariate analysis of the current study. Based on this result, lung volume irradiated with high dose is thought to be important in predicting RLD after tangential beam whole breast IMRT. Nevertheless, previous studies found lung volume receiving medium radiation dose such as V10-30 as a significant factor for lung damage after conventional RT for lung cancer [17] or breast cancer [4,7,8]. In regional lymph nodes treating breast cancer or lung cancer, multiple radiation fields are used and large volume of lungs are irradiated. Thus, lung volume receiving medium doses might be significantly different between the patients with lung complication and those without it, and therefore, it appeared to be statistically significant predictor for lung damage. On the other hand, in tangential fields IMRT for whole breast only irradiation, volume of irradiated lung is smaller than that of lung cancer or regional lymph nodes treating breast cancer. And radiation fields are restricted as tangents in these treatments. Therefore, the difference of lung volume irradiated with medium doses between the patients with pneumonitis and the patients without it might be too small to show a statistical significance. Comparisons of the dosimetric parameters for lung damage after breast RT were seldom reported. The effects of lung volume receiving high dose radiation on lung toxicity need to be evaluated in further studies.
Some studies have reported concurrent administration of tamoxifen with RT enhances the risk of radiation induced lung injury [9,18,19,20]. Other studies have not found such effects [5,16,21,22]. Tamoxifen induces transforming growth factor-β which is a key molecule in the early signaling cascade, and is thought to result in fibrosis. However, we found that the use of tamoxifen is not a relevant variable in predicting the risk of radiologic lung injury after whole breast IMRT. This is because the current study adopted different radiation fields and methods than the studies reporting negative predictive effect of tamoxifen on lung toxicity. The studies that reported on pulmonary fibrogenic effect of tamoxifen included large percentage of patients who were irradiated on regional lymph nodes as axillary, infraclavicular, supraclavicular, or internal mammary lymph nodes [9,18]. Irradiated lung volume in the above-mentioned studies might be larger than that of our study in which only five patients received supraclavicular lymph nodes irradiation. Concurrent administration of tamoxifen may increase radiologic lung damage more in cases of large lung volume irradiation than in small volume irradiation. The relation between tamoxifen and irradiated lung volume regarding lung fibrosis needs to be evaluated in future researches.
In the current study, all RLD were developed within 10 months after RT, and in 15 patients, alterations of lung were detected at 10 months after RT without preceding lung changes. Histopathologic response of lung by radiation is demonstrated radiologically in two patterns. Early phase which occurs within 4 to 12 weeks after completion of RT manifests as ground-glass opacities or consolidation in the irradiated fields. Late phase of lung damage, so called radiation fibrosis, usually develops within 6 to 12 months after RT, and is displayed as traction bronchiectasis, volume loss, and consolidation in the fields of radiation [23]. The fibrotic change of lung is caused mainly by progression of early radiation lung damage. Besides, de novo fibrotic change without early-phase reaction occurs in small proportion of patients [7,24]. The frequencies of de novo lung fibrosis after breast irradiation were reported at 11.4% in plain chest radiographs evaluations by Fröhlich [24], and 2.5% in the examination of chest CT by Kahán et al. [7]. Similar to these reports, the incidence of de novo pulmonary fibrosis stood at 9.5% in this study. While few studies have determined factors related to the occurrence of de novo lung fibrosis, the present study found that age is significantly relevant to the development of de novo lung fibrosis. In this study, de novo pulmonary fibrosis occurred more frequently in the patients aged 47 or under than the patients aged over 47, at the incidence of 11.7% (12 of 104) and 2.9% (3 of 104), respectively. Of note, the overall incidence of RLD was higher in older patients whereas, de novo pulmonary fibrosis was more frequently detected in younger patients.
Incidence of symptomatic pneumonitis ranged 1% to 18.9% after conventional breast RT in previous studies [4,7,8,9,14]. Similarly in the current study, symptomatic lung toxicity rarely developed despite frequent radiologic lung alteration following whole breast IMRT. Patients with pulmonary symptoms had larger CLD than the asymptomatic patients. However, it is difficult to draw any conclusion due to small number of patients with the symptoms.
In conclusions, it was not uncommon to detect RLD in follow-up CT after whole breast FIMRT. More detected cases of RLD among younger patients are believed to have developed at later points after RT than those of older patients. Age and V40 were significant predictors for RLD after whole breast IMRT.
Figures and Tables
Figure 1
The probabilities of radiation lung damage according to age and percent of lung volume receiving more than 40 Gy (V40). (A) The patients older than 47 years of age showed higher probability of radiologic lung damage (RLD) in comparison to the patients younger than 47 years of age (0.75 vs. 0.61, p=0.02). (B) In addition, the patients of V40 >7.2% had higher probability of RLD than the patients of V40 ≤7.2% with statistical significance (0.89 vs. 0.55, p<0.01).
RT=radiotherapy.
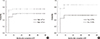
Table 2
Univariate logistic regression analyses in dosimetric parameters
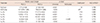
RLD=radiologic lung damage; AUC=area under the curve; ROC=receiver-operator characteristic; CLD=central lung distance; MLD=mean lung dose; Vx=percent of lung volume receiving more than X Gy.
*Median value of each dosimetric factor in patients with RLD and those without RLD; †Logistic regression analysis; ‡The cutoff point of the each variable was defined as a value that had lowest p-value by logistic regression analysis; §As the AUC of ROC value gets closer to 1.0, the parameter becomes more predictive of RLD.
Table 3
Univariate and multivariate analyses on radiologic lung injury after whole breast forward-planned intensity-modulated radiotherapy
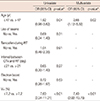
Notes
References
1. Early Breast Cancer Trialists' Collaborative Group (EBCTCG). Darby S, McGale P, Correa C, Taylor C, Arriagada R, et al. Effect of radiotherapy after breast-conserving surgery on 10-year recurrence and 15-year breast cancer death: meta-analysis of individual patient data for 10,801 women in 17 randomised trials. Lancet. 2011; 378:1707–1716.


2. Lind PA, Svane G, Gagliardi G, Svensson C. Abnormalities by pulmonary regions studied with computer tomography following local or local-regional radiotherapy for breast cancer. Int J Radiat Oncol Biol Phys. 1999; 43:489–496.


3. Ooi GC, Kwong DL, Ho JC, Lock DT, Chan FL, Lam WK, et al. Pulmonary sequelae of treatment for breast cancer: a prospective study. Int J Radiat Oncol Biol Phys. 2001; 50:411–419.


4. Lind PA, Wennberg B, Gagliardi G, Fornander T. Pulmonary complications following different radiotherapy techniques for breast cancer, and the association to irradiated lung volume and dose. Breast Cancer Res Treat. 2001; 68:199–210.


5. Wennberg B, Gagliardi G, Sundbom L, Svane G, Lind P. Early response of lung in breast cancer irradiation: radiologic density changes measured by CT and symptomatic radiation pneumonitis. Int J Radiat Oncol Biol Phys. 2002; 52:1196–1206.


6. Järvenpää R, Holli K, Pitkänen M, Hyödynmaa S, Rajala J, Lahtela SL, et al. Radiological pulmonary findings after breast cancer irradiation: a prospective study. Acta Oncol. 2006; 45:16–22.


7. Kahán Z, Csenki M, Varga Z, Szil E, Cserháti A, Balogh A, et al. The risk of early and late lung sequelae after conformal radiotherapy in breast cancer patients. Int J Radiat Oncol Biol Phys. 2007; 68:673–681.


8. Krengli M, Sacco M, Loi G, Masini L, Ferrante D, Gambaro G, et al. Pulmonary changes after radiotherapy for conservative treatment of breast cancer: a prospective study. Int J Radiat Oncol Biol Phys. 2008; 70:1460–1467.


9. Varga Z, Cserháti A, Kelemen G, Boda K, Thurzó L, Kahán Z. Role of systemic therapy in the development of lung sequelae after conformal radiotherapy in breast cancer patients. Int J Radiat Oncol Biol Phys. 2011; 80:1109–1116.


10. Pignol JP, Olivotto I, Rakovitch E, Gardner S, Sixel K, Beckham W, et al. A multicenter randomized trial of breast intensity-modulated radiation therapy to reduce acute radiation dermatitis. J Clin Oncol. 2008; 26:2085–2092.


11. Morganti AG, Cilla S, de Gaetano A, Panunzi S, Digesù C, Macchia G, et al. Forward planned intensity modulated radiotherapy (IMRT) for whole breast postoperative radiotherapy. Is it useful? When? J Appl Clin Med Phys. 2011; 12:3451.


12. Nishioka A, Ogawa Y, Hamada N, Terashima M, Inomata T, Yoshida S. Analysis of radiation pneumonitis and radiation-induced lung fibrosis in breast cancer patients after breast conservation treatment. Oncol Rep. 1999; 6:513–517.


13. Smith W, Menon G, Wolfe N, Ploquin N, Trotter T, Pudney D. IMRT for the breast: a comparison of tangential planning techniques. Phys Med Biol. 2010; 55:1231–1241.


14. Dörr W, Bertmann S, Herrmann T. Radiation induced lung reactions in breast cancer therapy. Modulating factors and consequential effects. Strahlenther Onkol. 2005; 181:567–573.


15. Jung YS, Na KY, Kim KS, Ahn SH, Lee SJ, Park HK, et al. Nation-wide Korean breast cancer data from 2008 using the breast cancer registration program. J Breast Cancer. 2011; 14:229–236.


16. Lind PA, Wennberg B, Gagliardi G, Rosfors S, Blom-Goldman U, Lideståhl A, et al. ROC curves and evaluation of radiation-induced pulmonary toxicity in breast cancer. Int J Radiat Oncol Biol Phys. 2006; 64:765–770.


17. Kim TH, Cho KH, Pyo HR, Lee JS, Zo JI, Lee DH, et al. Dose-volumetric parameters for predicting severe radiation pneumonitis after three-dimensional conformal radiation therapy for lung cancer. Radiology. 2005; 235:208–215.


18. Bentzen SM, Skoczylas JZ, Overgaard M, Overgaard J. Radiotherapy-related lung fibrosis enhanced by tamoxifen. J Natl Cancer Inst. 1996; 88:918–922.


19. Huang EY, Wang CJ, Chen HC, Sun LM, Fang FM, Yeh SA, et al. Multivariate analysis of pulmonary fibrosis after electron beam irradiation for postmastectomy chest wall and regional lymphatics: evidence for non-dosimetric factors. Radiother Oncol. 2000; 57:91–96.


20. Koc M, Polat P, Suma S. Effects of tamoxifen on pulmonary fibrosis after cobalt-60 radiotherapy in breast cancer patients. Radiother Oncol. 2002; 64:171–175.


21. Holli K, Pitkänen M, Järvenpää R, Rajala J, Lahtela S, Hyödynmaa S, et al. Early skin and lung reactions in breast cancer patients after radiotherapy: prospective study. Radiother Oncol. 2002; 64:163–169.


22. Harris EE, Christensen VJ, Hwang WT, Fox K, Solin LJ. Impact of concurrent versus sequential tamoxifen with radiation therapy in early-stage breast cancer patients undergoing breast conservation treatment. J Clin Oncol. 2005; 23:11–16.


23. Choi YW, Munden RF, Erasmus JJ, Park KJ, Chung WK, Jeon SC, et al. Effects of radiation therapy on the lung: radiologic appearances and differential diagnosis. Radiographics. 2004; 24:985–997.


24. Fröhlich D. Radiation pneumopathy. I. Analysis of clinical and x-ray findings. Radiobiol Radiother (Berl). 1987; 28:309–319.