Abstract
Purpose
Breast cancer is the most common type of cancer in women. Despite various pharmacological developments, the identification of new therapies is still required for treating breast cancer. Crab is often recommended as a traditional medicine for cancer. This study aimed to determine the in vitro effect of a hydroalcoholic crab shell extract on a breast cancer cell line.
Methods
In this experimental study, MCF7 breast cancer cell line was used. Crab shell was powdered and a hydroalcoholic (70° ethanol) extract was prepared. Five concentrations (100, 200, 400, 800, and 1,000 µg/mL) were added to the cells for three periods, 24, 48, and 72 hours. The viability of the cells were evaluated using trypan blue and 3-(4, 5-dimethylthiazol-2-yl)-2,5-diphenyltetrazolium bromide assays. Cell apoptosis was determined using the terminal deoxynucleotidyl transferase dUTP nick end labeling method. Nitric oxide (NO) level was assessed using the Griess method. Data were analyzed using analysis of variance, and p<0.05 was considered significant.
Results
Cell viability decreased depending on dose and time, and was significantly different in the groups that were treated with 400, 800, and 1,000 µg/mL doses compared to that in the control group (p<0.001). Increasing the dose significantly increased apoptosis (p<0.001). NO secretion from MCF7 cells significantly decreased in response to different concentrations of the extract in a dose- and time-dependent manner (p<0.050).
Cancer is a major health problem and one of the main causes of mortality worldwide [1]. At present, breast cancer is the most common cancer in women around the world [2]. The common therapies for cancer are surgery and chemotherapy. Chemotherapeutical agents greatly damage cell DNA, affecting normal cells as well as cancer cells, and cause side effects such as digestive problems, leukopenia, and hair loss. Furthermore, drug resistance limits the effectiveness of chemotherapy [3].
Approximately 60% of the drugs approved for cancer treatment are derived from natural sources. Vincristine, etoposide, irinotecan, taxane, and camptothecin are examples of plant-derived compounds [4]. One of the important compounds in cancer treatment is chitin, (C8H13O5N)n. It was first derived from a fungus and is the first known polysaccharide [5]. Potent acids can dissociate chitin, a water-insoluble polymer, into acetic acid and chitosan. Chitosan is a nontoxic polymer produced by the deacetylation of chitin. Chitin is the main component of arthropod exoskeletons, tendons, and respiratory tract lining, and cell walls of the majority of fungi. Crustacean shells are a source of chitin (20%-30%). Crab shell also contains lipidic pigments such as carotenoids (astaxanthin, astatine, and canthaxanthin), lutein, and β-carotene [6].
Jeon and Kim [7] found that oligomers of chitosan exhibit antitumor activities in vitro and in vivo. Low-molecular-weight chitosan has been shown to have remarkable antimetastatic effects against lung cancer in mice [8]. Maeda and Kimura [9] investigated the antitumor effects of low-molecular-weight chitosan and reported a decline in tumor growth and final tumor weight in mice with sarcomas. In recent years, the viability of cancer cells has remarkably decreased in some cell lines, regardless of whether the chito-oligosaccharide (COS) derivatives were positively or negatively charged [10].
Carotenoids are yellow-orange pigments that are found in all higher plants and some animals. A diet containing carotenoids can decrease the risk of cancer in various tissues. Clinical studies have shown that some carotenoids have powerful antitumor activities both in vitro and in vivo [11]. The anticancer activities of different natural carotenoids as well as β-carotene have been documented. Carotenoids (α-carotene and lutein), zeaxanthin, lycopene, astaxanthin, crocetin, and β-carotene can prevent cancer [12]. Sachindra et al. [13] examined the quantitative and qualitative distribution of carotenoids in the meat and shell of the major marine crab (Charybdis cruciata) and fresh-water crab (Potamon). The total carotenoid content in both species was low. Astaxanthin and its esters, and zeaxanthin were the major carotenoids found in the fresh-water crab extract.
Selenium is an essential part of the diet of organisms, including humans, and acts as a protective factor against cancer. Although the anticancer activity of selenium is not completely known, it has been suggested that various mechanisms, such as antioxidant protection by selenoenzymes, inhibit the development of tumor cells, regulate and modulate the cell cycle, inhibit apoptosis, and repair DNA. Recent studies have indicated that selenium not only has the potential to prevent cancer but could also be used to treat cancer and enhance the effectiveness of cancer therapy in combination with other anticancer drugs or radiation [14]. In cell culture models, selenium compounds have been shown to inhibit the development of cancer cells by reducing cell proliferation via cell cycle control or an increase in apoptosis [15].
Considering the current prevalence of breast cancer and the necessity to identify and develop new pharmaceutical compounds, especially those with a natural origin, as well as the primary information obtained from traditional medicine, this study was aimed to determining the effect of hydroalcoholic crab shell extract on the viability, apoptosis, and nitric oxide (NO) secretion of MCF7 cell line.
The MCF7 cell line was treated with hydroalcoholic crab shell extract (100, 200, 400, 800, and 1,000 µg/mL doses) for three periods, 24, 48, and 72 hours. Each experiment was repeated 3 to 5 times and the means of the data were used.
Fresh-water crab was prepared and identified in terms of sex and species (Potamon persicum) by a zoologist (from Razi University, Kermanshah, Iran). The crab shell was powdered, and 5 g of powder was dissolved in 150 mL of 70% ethanol for 48 hours in darkness. It was subsequently filtered through filter paper and dried to evaporate the alcohol; thereafter, the final powder was weighed (0.59 g). The solvent of the final powder was a serum-free cell culture medium (RPMI 1640), and the prepared concentrations were passed through a 0.22-µm filter before use.
A total of 3×104 MCF7 cells were cultured in each well of a 24-well culture dish. Subsequently, 750 µL of RPMI 1640 (containing 10% fetal bovine serum) was added to each well and the dishes were kept in a CO2 incubator for 24 hours. The supernatants were then removed and 1 mL of serum-free RPMI 1640 medium containing 0 (control), 100, 200, 400, 800, and 1,000 µg/mL concentrations of crab shell extract was added to each well. After 24 hours, the supernatant of each well was removed and frozen at -20℃ for NO measurement. The cells were then detached using trypsin (0.25%, 100 µL), stained with trypan blue, and counted using a Neubauer chamber, and then, their viability was calculated. Cell viability was similarly calculated for wells incubated for 48 and 72 hours.
The basis of this test is that the mitochondrial enzyme succinate dehydrogenase in viable cells is capable of reducing the tetrazolium MTT salt to insoluble formazan, which is purple in color; the greater the number of viable cells, the greater the intensity of the stain and vice versa. To perform the test, 15×103 MCF7 cells were loaded into a 96-well plate and 200 µL of RPMI medium containing 7% serum was added. After 24-hour incubation, 200 µL of various concentrations of the extract (0, 100, 200, 400, 800, and 1,000 µg/mL) dissolved in serum-free RPMI 1640 medium was added to the wells. The cells were separately incubated with different doses for 24, 48, and 72 hours.
After incubation, the MTT test was performed in which the supernatant from each well was removed and 500 µL of MTT solution (5 mg/mL) was added to each well and incubated for 3 hours. The supernatant from each well was then removed and 100 µL of dimethyl sulfoxide was added to dissolve the formazan crystals at room temperature for 30 minutes. The optical density of each well was measured using an enzyme-linked immunosorbent assay (ELISA) reader (STAT FAX 2100; Awareness Technology, Westport, USA) at 570 and 630 nm. The viability of the cells for each concentration was calculated using the following formula [16]:
NO was measured using the Griess staining method. For each sample, 400 µL of thawed supernatant was deproteinized by adding 6 mg of zinc sulfate. The samples were centrifuged at 4℃ and 12,000 g for 12 minutes. Separately, 100 µL of 0, 6.25, 12.5, 25, 50, 100, and 200 µM sodium nitrite was poured into standard wells. We then added 100 µL of the deproteinized samples to each well, and 100 µL of vanadium chloride, 50 µL of sulfanilamide, and 50 µL of N-(1-naphthyl) ethylenediamine dihydrochloride were added to all the wells (standard and samples). All wells were incubated for 15 minutes and were assessed using an ELISA Reader at wavelengths of 570 and 630 nm [17].
Apoptosis in the MCF7 cells was analyzed using the TUNEL method. This method is used to determine DNA fragmentation during apoptosis cell death. MCF7 cancer cells were cultured in 96-well culture plates with 15×103 cells in each well. Subsequently, 200 µL of RPMI 1640 medium was added to each well and incubated for 24 hours. The supernatant was then removed and 200 µL of each dose of crab shell extract was added to the cells and incubated for 72 hours. The cells were then fixed in 4% paraformaldehyde for 1 hour. The permeability of the cell membranes was increased by adding 0.2% Triton x-100 solution (Sigma, St. Louis, USA) for 2 minutes on ice. The cells were then incubated with TUNEL mixture solution at 37℃ in dark conditions for 1 hour. Finally, differential staining of the cells was performed using propidium iodide (5 µg/mL; PI) and the cells were analyzed using a fluorescence microscope (Eclipse TS100; Nikon, Tokyo, Japan) after being washed with phosphate-buffered saline three times [18]. The apoptotic index of the cells was calculated as the percentage of apoptotic cells relative to the total cell number.
To determine the extract contents, it was subjected to gas chromatography-mass spectrometry (GC-MS). The type of GC device used was an Agilent 6890 with a HP-5MS column with a length of 30 m, internal diameter of 0.25 mm, and thickness of 0.25 µm. The column temperature plan consisted of a primary temperature of 50℃, with a 5-minute retention period and a thermal gradient of 3℃ every minute. The temperature was then increased to 180℃ with a velocity of 10℃ per minute, and then to 260℃ with a 4-minute retention period. Helium was used as the carrier gas with a 1-mL/min flow speed. The total analysis was completed in 45 minutes. The results obtained from the analysis were compared using the data present in the machine. Biochemical compounds (75) were obtained from the analysis, which was related to retention time and extraction of compounds from the extract (Table 1). Moreover, atomic absorption spectrometry (VGA 77; Varian Inc., Mulgrave, Australia) was used to determine the amount of selenium in the extract.
Comparison of the mean MCF7 cell viabilities analyzed using the trypan blue method after 24, 48, and 72 hours indicated a significant difference between the groups treated with crab shell extract (400, 800, and 1,000 µg/mL) compared to the control group (p<0.001). Increasing the dose significantly decreased the cell viability (Figure 1A, B).
Comparison of the mean MCF7 cell viabilities analyzed using the MTT assay after 24, 48, and 72 hours revealed a significant difference between the groups treated with crab shell extract (400, 800, and 1,000 µg/mL) in comparison with the control group. Cell viability was again significantly decreased by increasing the dose (Figure 2).
NO concentration was evaluated using the Griess method. The effect of different concentrations of crab shell extract on MCF7 cells after 24, 48, and 72 hours indicated a dose- and time-dependent decrease in NO secretion. The difference compared to the control group was significant with the 800 and 1,000 µg/mL doses (p<0.050) (Figure 3).
The apoptosis index of MCF7 cells treated with crab shell extract showed an increase from control to experimental groups in order of concentration, exhibiting a significant difference with 400 (p<0.050), and 800 and 1,000 µg/mL (p<0.001) doses (Figure 4A, B).
In this study, the effect of crab shell hydroalcoholic extract on a breast cancer cell line (MCF7) was evaluated. Five doses (100, 200, 400, 800, and 1,000 µg/mL) of the extract were prepared and their effects on the cells were analyzed over three time periods of 24, 48, and 72 hours. MTT assay and trypan blue methods were used to determine cell viability. A decreasing trend was observed in cell viability along with a rising trend in apoptosis, with significant differences with 400, 800, and 1,000 µg/mL doses compared with the control group.
The NO level in the MCF7 culture medium of extract-treated cells significantly decreased depending on dose and time at 800 and 1,000 µg/mL doses after 48 and 72 hours. Generally, 24, 48, and 72-hour periods [19] and 100-1,000 µg/mL doses [20] are used to analyze the effects of natural compounds on cancer cell lines. The antiproliferative properties of fresh-water crab shell extract can be attributed to the presence of carotenoids, chitin derivatives (COS and chitosan), and selenium, which induce apoptosis and decrease NO secretion.
Chitin is the second natural polymer frequently found in the exoskeleton of crustaceans and cell wall of fungi. Chitosan and COS are its main derivatives, for which the antiproliferative properties have been reported in different cancer cell lines in various studies [9,10,11]. COS extracted from fungi has also been reported to inhibit the development of a liver carcinoma cell line (HepG2) at five concentrations and over three periods [19], which is similar to the findings of the present study in terms of inhibition of MCF7 cell proliferation at different doses and times.
The effect of raw and cooked crab shell extract and its derivative carotenoids on a liver-tumor cell line (AH109A) has previously been evaluated and the inhibitory effect observed has been attributed to the antioxidant effect of the extract [21]. However, in the present study, the inhibitory effect of crab shell extract on a breast cancer cell line was associated with NO decrease and induction of apoptosis. The inhibitory effect on MCF7 cells was due to decreasing NO secretion, as reported by Yin et al. [22], although decreasing cyclooxygenase 2 and cytokine expression are additional factors to consider.
A decrease in NO secretion and dose- and time-dependent decrease in the viability of a liver carcinoma cell line (HepG2) and subsequent larynx carcinoma (Hep2) have been attributed to saffron extract and its carotenoids [23]; this finding is in line with the results of the present study. The effect of one of the components of selenium, methyl selenic acid, on the viability of MCF7 cancer cells assessed using the MTT assay showed inhibition of cell development by 50% over three periods of 24, 48, and 72 hours and an increase in cell apoptosis after 48 hours [19]. As in the present study, increasing the dose and time caused cell growth to decline but viability did not fall below 50%, which could be because raw extract was used in the present study unlike the previous study that used distinct and pure compounds. Furthermore, similar apoptotic effects of selenocysteine on MCF7 cells and of sodium selenite on a prostate cancer cell line (JCA1, DU145) have been reported in the studies of Chen and Wong [18] and Gasparian et al. [24], respectively.
Moreover, an antioxidant and antiproliferative effect on a human bladder cancer cell line (T24) has been reported after 48 hours, depending on chitosan concentration [25]. The effect of one of the compounds derived from chitin, astaxanthin, on a human esophagus cancer cell line (TE-4) over 24, 48, and 72 hours was investigated, and dose-dependent inhibition was observed [26]. Furthermore, astaxanthin derived from the green alga Haematococcus pluvialis exerted an inhibitory effect on a large intestine cancer cell line (HCT-116) via apoptosis induction [27]. The findings obtained in the present study are similar to those obtained previously, and confirm the findings regarding different cancer cell lines. Our study had limitations: we did not evaluate the effect of crab sell extract on normal breast epithelial cells. Moreover, using MCF10A cell as normal breast epithelial cell line, can't substitute normal cell characteristic completely. MCF10A cell line has a near-diploid karyotype with modest genetic modifications [28].
In conclusion, crab shell extract inhibited the proliferation of a breast cancer cell line in a dose- and time-dependent manner. It seems that the extract exerts its effect through a reduction in NO production and induction of apoptosis. Chitin derivatives, carotenoids, and selenium are important factors to consider in the process of growth inhibition.
Figures and Tables
Figure 1
(A) MCF7 viability (%) after 24, 48, and 72-hour exposed to different doses of crab shell extract. Significant difference between the experiments and control groups were seen.
*p<0.05; †p<0.001. (B) MCF7 cells in control and different doses of the extract at 72 hours by invert microscope (×100).
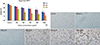
Figure 2
MCF7 cell line viability (%) in different crab shell extracts and different culture periods. There is significant difference between the groups.
*p<0.05; †p<0.001.
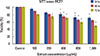
Figure 3
Nitric oxide (NO) levels in different groups and different culture periods. Significant difference between groups.
*p<0.05.
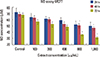
Figure 4
(A) MCF7 apoptotic index (%) after 72-hour with different amounts of crab shell extract. Significant difference between groups. *p<0.05; †p<0.001. (B) Identification of apoptosis in MCF7 cell line following terminal deoxynucleotidyl transferase dUTP nick end labeling (TUNEL) staining and counterstaining with propidium iodide after 72 hours of exposure crab shell extract. TUNEL positive cells (apoptotic nuclei) identified by a distinct bright yellow stained chromatin (arrows). C+: The positive control was incubated with ethanol 10% for 10 minutes, all cells are seen as bright yellow. C-: The positive control was incubated with label solution without enzyme solution. No TUNEL positive cells were observed (×200).
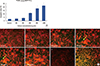
Notes
References
1. Rani D, Somasundaram VH, Nair S, Koyakutty M. Advances in cancer nanomedicine. J Indian Inst Sci. 2012; 92:187–218.
2. Curado MP. Breast cancer in the world: incidence and mortality. Salud Publica Mex. 2011; 53:372–384.
3. Kateb B, Chiu K, Black KL, Yamamoto V, Khalsa B, Ljubimova JY, et al. Nanoplatforms for constructing new approaches to cancer treatment, imaging, and drug delivery: what should be the policy? Neuroimage. 2011; 54:Suppl 1. S106–S124.


4. Grever MC. Cancer drug discovery and development. In : De Vita VH, Rosenberg SA, editors. Cancer: Principles and Practice of Oncology. Philadelphia: Lippincott-Raven;2001. p. 328–339.
5. Domard A, Domard M. Chitosan: structure-properties relationship and biomedical applications. In : Dumitriu S, editor. Polymeric Biomaterials. 2nd ed. New York: Marcel Dekker Inc.;2002. p. 187–212.
6. Acosta N, Jiménez C, Borau V, Heras A. Extraction and characterization of chitin from crustaceans. Biomass Bioenergy. 1993; 5:145–153.


7. Jeon YJ, Kim SK. Antitumor activity of chitosan oligosaccharides produced in ultrafiltration membrane reactor system. J Microbiol Biotechnol. 2002; 12:503–507.
8. Tsukada K, Matsumoto T, Aizawa K, Tokoro A, Naruse R, Suzuki S, et al. Antimetastatic and growth-inhibitory effects of N-acetylchitohexaose in mice bearing Lewis lung carcinoma. Jpn J Cancer Res. 1990; 81:259–265.


9. Maeda Y, Kimura Y. Antitumor effects of various low-molecular-weight chitosans are due to increased natural killer activity of intestinal intraepithelial lymphocytes in sarcoma 180-bearing mice. J Nutr. 2004; 134:945–950.


10. Huang R, Mendis E, Rajapakse N, Kim SK. Strong electronic charge as an important factor for anticancer activity of chitooligosaccharides (COS). Life Sci. 2006; 78:2399–2408.


11. Tanaka T, Shnimizu M, Moriwaki H. Cancer chemoprevention by carotenoids. Molecules. 2012; 17:3202–3242.


12. Nishino H, Murakosh M, Ii T, Takemura M, Kuchide M, Kanazawa M, et al. Carotenoids in cancer chemoprevention. Cancer Metastasis Rev. 2002; 21:257–264.
13. Sachindra NM, Bhaskar N, Mahendrakar NS. Carotenoids in crabs from marine and fresh waters of India. Lebenson Wiss Technol. 2005; 38:221–225.


14. Brozmanová J, Mániková D, Vlčková V, Chovanec M. Selenium: a double-edged sword for defense and offence in cancer. Arch Toxicol. 2010; 84:919–938.


16. Ghosh K, Chandra K, Ojha AK, Sarkar S, Islam SS. Structural identification and cytotoxic activity of a polysaccharide from the fruits of Lagenaria siceraria (Lau). Carbohydr Res. 2009; 344:693–698.


17. Miranda KM, Espey MG, Wink DA. A rapid, simple spectrophotometric method for simultaneous detection of nitrate and nitrite. Nitric Oxide. 2001; 5:62–71.


18. Chen T, Wong YS. Selenocystine induces caspase-independent apoptosis in MCF-7 human breast carcinoma cells with involvement of p53 phosphorylation and reactive oxygen species generation. Int J Biochem Cell Biol. 2009; 41:666–676.


19. Shah YM, Kaul A, Dong Y, Ip C, Rowan BG. Attenuation of estrogen receptor alpha (ERalpha) signaling by selenium in breast cancer cells via downregulation of ERalpha gene expression. Breast Cancer Res Treat. 2005; 92:239–250.


20. Shen KT, Chen MH, Chan HY, Jeng JH, Wang YJ. Inhibitory effects of chitooligosaccharides on tumor growth and metastasis. Food Chem Toxicol. 2009; 47:1864–1871.


21. Nakahara S, Miura Y, Yagasaki K. Inhibitory action of carotenoids in crab shell on the invasion of hepatoma cells co-cultured with mesothelial cells. European Society of Animal Cell Technology. Japanese Association for Animal Cell Technology. Animal Cell Technology: Challenges for the 21st Century. Boston: Kluwer;2002. p. 409–413.
22. Yin MB, Li ZR, Tóth K, Cao S, Durrani FA, Hapke G, et al. Potentiation of irinotecan sensitivity by Se-methylselenocysteine in an in vivo tumor model is associated with downregulation of cyclooxygenase-2, inducible nitric oxide synthase, and hypoxia-inducible factor 1alpha expression, resulting in reduced angiogenesis. Oncogene. 2006; 25:2509–2519.


23. Parizadeh MR, Gharib FG, Abbaspour AR, Afshar JT, Ghayour-Mobarhan M. Effects of aqueous saffron extract on nitric oxide production by two human carcinoma cell lines: hepatocellular carcinoma (HepG2) and laryngeal carcinoma (Hep2). Avicenna J Phytomed. 2011; 1:43–50.
24. Gasparian AV, Yao YJ, Lü J, Yemelyanov AY, Lyakh LA, Slaga TJ, et al. Selenium compounds inhibit I kappa B kinase (IKK) and nuclear factor-kappa B (NF-kappa B) in prostate cancer cells. Mol Cancer Ther. 2002; 1:1079–1087.
25. Kuppusamy S, Karuppaiah J. Antioxidant and cytotoxic efficacy of chitosan on bladder cancer. Asian Pac J Trop Dis. 2012; 2:Suppl 2. S769–S773.


26. Lim SA, Lee JY, Jung WH, Lim EH, Joo MK, Lee BJ, et al. Anticancer effects of astaxanthin and alpha-tocopherol in esophageal cancer cell lines. Korean J Helicobacter Up Gastrointest Res. 2011; 11:170–175.

