Abstract
Purpose
This study investigated the additive effect of photodynamic therapy (PDT) plus traditional radiotherapy (RT) for patients with breast cancer and chest wall recurrence.
Methods
A total of 40 patients with recurrent breast cancer were prospectively randomized to receive RT alone (group A, n=20) or PDT and RT in combination (group B, n=20). Traditional RT at a dose of 50 Gy was delivered in 25 fractions with or without exposure to 5-aminolevulinic acid and red light as PDT.
Results
The response rates were not statistically different between the groups, but more patients achieved a complete response (CR) in group B (50%) than in group A (20%). The median time to CR in group B was significantly shorter than that in group A (109.6 days vs. 175.2 days, p=0.001). Adverse event profiles were not different between the groups.
Breast cancer is the most common malignancy among women. The incidence of breast cancer is high in many countries and other regions, and this malignancy is among the major causes of death. The local relapse rate of breast cancer is approximately 5% to 45% after surgery [1,2,3], primarily because of residual tumor infiltration to the skin, subcutaneous nodules, and rarely the chest wall. Tumor size, the histological tumor grade, the extent of lymph node involvement, and vascular invasion are strongly associated with chest wall recurrence. Although local recurrence after breast-conserving surgery can be treated via mastectomy, this recurrence might indicate a risk of distant metastasis, which is more difficult to treat [4]. There are several treatment options for chest wall recurrence. Surgical resection is the preferred treatment with a better prognosis for node-negative patients who experienced local recurrence 1 year after mastectomy [5], but it is not applicable for patients who require full thickness chest wall resection or have a poor skin condition. Therefore, surgical resection is only applicable for a small number of patients [6], and postoperative radiotherapy (RT) or other systemic treatment is required to prevent distant metastasis. Other treatment options such as radiation therapy, chemotherapy, and electrochemical therapy can be considered when surgery is not applicable. However, patients who received prior radiotherapy will not be amenable to further RT. In addition, inoperable progressive skin lesions of breast cancer can be treated with miltefosine solution. A phase III randomized, controlled clinical trial demonstrated a response rate of 33.3% with miltefosine compared with 3.7% in the placebo, whereas grade 3 or 4 cutaneous reactions were observed in 33.4% of miltefosine-treated patients versus 7.4% for the placebo group [7].
Light has been considered a therapeutic agent for thousands of years [8,9]. The combination of light and certain chemicals can promote apoptosis. The use of hematoporphyrin derivatives for the treatment of breast cancer with chest wall recurrence was first discovered in 1966 [10,11]. The development of photodynamic therapy (PDT) succeeded in basic scientific studies [12,13,14], and clinical research was conducted [13]. The therapeutic advantages of PDT are its abilities to target breast tumors and minimize damage to other normal tissues. It also has synergistic anticancer effects with chemotherapy. The photodynamic reaction requires a photosensitizer, light, and oxygen. A series of photochemical and photobiological reactions can result in the death of cancer cells. The sensitizer molecule absorbs light and then emits fluorescence after excitation, during which the energy released produces singlet oxygen [15]; the therapeutic effect of PDT is influenced by the type of photosensitizer, lighting conditions, tissue metabolism, and cell types [16].
The anticancer effects of PDT include direct killing of cancer cells and indirect induction of apoptosis and immune responses against the tumor. In this study, we investigated the efficacy and safety of PDT in combination with traditional RT for the treatment of breast cancer.
The study was conducted at the Tianjin Medical University Cancer Institute and Hospital between April 2010 and September 2011. The study was approved by the local ethics committee (Tianjin Cancer Hospital Medical Ethics Committee; approval number, Ebc200910). The inclusion criteria were as follows: all patients must have undergone radical mastectomy and completed adjuvant chemotherapy and/or endocrine therapy or RT before a histological diagnosis of the local recurrence of invasive ductal carcinoma. In addition, the longest diameter of the invasive lesion at the recurrent site must be <6 cm. The exclusion criteria included bilateral breast cancer and/or distant metastases. Patients with photosensitivity diseases were also excluded. Eligible patients were equally randomized prospectively into two groups. Group A patients received chest wall RT only, and group B patients received the combination of PDT and chest wall RT (PDT-RT). The therapeutic outcomes were stratified according to the presence of oozing scabs. PDT was administered as an intravenous infusion of 5-aminolevulinic acid at a dose of 4 mg/kg. Forty-eight hours later, red light (wavelength, 632.8 nm) at a median dose (288 J/cm2) of 100 mW was administered each time before RT. RT included 6-MeV energy electron beam irradiation with a dose of 50 Gy (25 fractions) delivered to the full chest wall and ipsilateral supraclavicular fossa according to institutional practice (Figure 1). In patients in group B, chest wall RT was administered 1 day after PDT. Efficacy and safety assessments included vital signs, physical examinations, tumor size, morphological changes of the lesion, and adverse events. All patients were assessed daily during the week of PDT and followed up once every month after the completion of treatment for a total of 6 months. Follow-up consisted of digital photography and a physical examination for every patient. The primary and secondary endpoints of this study were the objective response rate and adverse events, respectively. Complete response was defined as the disappearance of palpable, radiographical, and/or visually apparent tumors. Partial response was defined as a ≥50% decrease in the sum of the products of the perpendicular diameters of the measurable lesions. Stable disease was defined as a <50% decrease or <25% increase in the products of the perpendicular diameters of the measurable lesions. Disease progression was defined as a ≥25% increase in any measurable lesion or the appearance of a new lesion. The study endpoints were compared between the groups using SPSS version 17.0 for Windows (SPSS Inc., Chicago, USA). The chi-square test was used to compare categorical variables between the groups. All statistical tests were two-sided, and p-values <0.05 were considered statistically significant.
A total of 40 patients with histologically confirmed chest wall recurrence were equally randomized into groups A (n=20) and B (n=20). More than 70% of the patients were aged 35 to 65 years. All patients underwent efficacy and safety evaluations in this study. The baseline characteristics of the patients are listed in Table 1. No significant difference in baseline characteristics was observed between the groups.
Overall response rates were not significantly different between the groups (Table 2), but the complete response rate was higher in group B (50%) than in group A (20%) (p=0.047). The median time to complete response in group B was significantly shorter than that in group A (109.6 days vs. 175.2 days, p=0.001). Among patients in group B, all patients with lesions <1.0 cm in diameter responded to the treatment, whereas the response rate decreased with increasing lesion size (p<0.001). Similarly, the response rate also decreased with increasing lesion size in group A (p<0.001) (Figure 2). Further stratified analysis of response rates according to the presence of oozing scabs was performed in each group. The response rate was not associated with the presence of oozing scabs in group A (p=0.144), but the absence of oozing scabs was associated with a higher response rate in group B (p=0.037) (Table 3). At a median follow-up of 6 months after treatment, 14 (70%) and 16 (80%) patients in groups A and B, respectively, did not experience a second episode of chest wall recurrence (p=0.47). Systemic progression was also not observed in 17 (85%) and 18 (90%) patients in groups A and B, respectively.
No treatment-emergent death was observed in this study. One patient in group A experienced severe chest pain, and the condition improved with analgesics. One patient in group B experienced severe skin toxicity, and the condition improved with appropriate treatment during hospitalization. No other severe adverse event was observed. A total of five patients required dose modification in group B, versus no patients in group A. No significant difference was observed for the incidence of other mild or moderate adverse events between the groups (Table 4).
It is well noted that approximately 5% to 45% of patients with breast cancer experience local recurrence [1,2,3] after surgery, and many of them have distant metastases. Adjuvant RT is an important disease management strategy to reduce the risk of relapse and further distant spread of disease. However, several safety issues are associated with RT, such as nausea, vomiting, other systemic symptoms, redness, itching and skin peeling, and the emergence of a new tumor rarely. PDT is another noninvasive treatment that selectively targets cancer cells, with little damage to normal cells. The common adverse reactions associated with PDT include skin hypersensitivity to light, and only a small number of patients experience dyspnea, dysphagia, or reactive edema. PDT is an alternative treatment option for patients with advanced breast cancer who are unsuitable or not amenable to conventional treatment.
In this study, only patients with chest wall recurrence were randomized to compare the treatment outcomes between RT and PDT-RT. Despite the insignificant difference in efficacy between the two treatment modalities, more patients treated with PDT-RT achieved a CR within a shorter period than those treated with RT alone. Nuclear DNA is the main target for ionizing radiation-induced cytotoxicity. PDT causes several types of DNA lesions by generating singlet oxygen. There may be potential synergistic interactions between PDT and RT [17]. It remains unclear whether PDT and RT interact synergistically or additively. It has been demonstrated that regardless of the sequence of application, the action of PDT and RT was usually additive [18]. Prinsze et al. [19] found that these treatments had synergistic effects in L929 fibroblasts, whereas their effects were additive in Chinese hamster ovary and T24 cells. In another study, the researchers believed only simultaneous PDT and RT treatment resulted in a synergic interaction [20]. The study indicated that approximately half of the damage caused by RT was repaired within 5 minutes, and the remainder was repaired within 30 minutes [21]. This may explain why only simultaneous PDT and RT treatment displayed a synergic interaction, as time intervals <1 minute are important for the "fast reparation system" to work effectively. In our study, we treated the patients with PDT followed by RT. The results illustrated that more patients treated with PDT-RT achieved a CR within a shorter period than those treated with RT alone. Further investigation is required to determine whether the treatments interact synergistically or additively. Nevertheless, a shorter time to response may offer a better quality of life to patients. Considering the small number of patients accrued in this study, further investigation on the treatment benefit with a larger sample size is required.
The treatment efficacy of PDT depends on the cellular uptake of the photosensitizer. As the concentration gradient of the photosensitizer increases between tumors and surrounding normal cells that remove the photosensitizer as time passes, more cancer cells will be killed. The treatment effect is also associated with the wavelength of the light used for PDT. In our study, light with a wavelength of 630 nm and a skin penetration depth of 0.5 to 1.0 cm was selected [22]. The PDT-RT treatment effect was obvious for lesions <1 cm in diameter and noticeable for lesions >1 cm in diameter. Some studies demonstrated that PDT could damage micro-blood vessels [23,24] and result in tissue hypoxia [25], hindering tumor growth. In addition, some studies found that PDT could induce lymphocyte, leukocyte, and macrophage infiltration, indicating activation of the immune response [26,27]. de Vree et al. [28] discovered that PDT could promote neutrophil aggregation and that it could inhibit tumor growth in a mouse model, and Korbelik et al. [29] observed a higher, albeit without significance, cure rate in the PDT-treated tumors of SCID mice reconstituted with BALB/c bone marrow. These findings demonstrated a possible relationship between the efficacy of PDT and the immune response, and they are consistent with our study results.
Further stratified analysis according to the chest wall condition demonstrated that the presence of oozing scabs did not influence treatment outcomes; however, among patients who received PDT-RT, patients without oozing scabs were more likely to respond to the therapy than those with oozing scabs. It might be possible that the presence of oozing scabs could affect the treatment outcome because these scabs could influence the amount of light penetrating the skin to reach the target lesions. PDT consists of three essential components: a photosensitizer, light, and oxygen. The effect of PDT is limited in a hypoxic environment, whereas oozing itself appears to reflect serious conditions such as tissue necrotization and hypoxia. The presence of oozing scabs might be a predictive factor of treatment outcome, and the removal of oozing scabs might improve the therapeutic outcome of PDT.
The major toxicity of PDT was skin sensitivity to light, which occurred in approximately 20% of patients [30]. Other RT-associated side effects included nausea, vomiting, other systemic symptoms, exposure site redness, and itching and skin peeling. No significant difference in toxicity profiles was noted between the treatment modalities, and the combination treatment can be considered safe.
Figures and Tables
Figure 2
Comparisons of response rates between groups according to the size of lesion.
PDT=photodynamic therapy; RT=traditional radiotherapy.
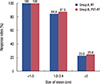
Notes
References
1. Lee MC, Jagsi R. Postmastectomy radiation therapy: indications and controversies. Surg Clin North Am. 2007; 87:511–526.


2. Danish Breast Cancer Cooperative Group. Nielsen HM, Overgaard M, Grau C, Jensen AR, Overgaard J. Study of failure pattern among high-risk breast cancer patients with or without postmastectomy radiotherapy in addition to adjuvant systemic therapy: long-term results from the Danish Breast Cancer Cooperative Group DBCG 82 b and c randomized studies. J Clin Oncol. 2006; 24:2268–2275.


3. Ragaz J, Olivotto IA, Spinelli JJ, Phillips N, Jackson SM, Wilson KS, et al. Locoregional radiation therapy in patients with high-risk breast cancer receiving adjuvant chemotherapy: 20-year results of the British Columbia randomized trial. J Natl Cancer Inst. 2005; 97:116–126.


4. van der Pol CC, van Geel AN, Menke-Pluymers MB, Schmitz PI, Lans TE. Prognostic factors in 77 curative chest wall resections for isolated breast cancer recurrence. Ann Surg Oncol. 2009; 16:3414–3421.


5. Chagpar A, Meric-Bernstam F, Hunt KK, Ross MI, Cristofanilli M, Singletary SE, et al. Chest wall recurrence after mastectomy does not always portend a dismal outcome. Ann Surg Oncol. 2003; 10:628–634.


6. Koppert LB, van Geel AN, Lans TE, van der Pol C, van Coevorden F, Wouters MW. Sternal resection for sarcoma, recurrent breast cancer, and radiation-induced necrosis. Ann Thorac Surg. 2010; 90:1102–1108.e2.


7. Leonard R, Hardy J, van Tienhoven G, Houston S, Simmonds P, David M, et al. Randomized, double-blind, placebo-controlled, multicenter trial of 6% miltefosine solution, a topical chemotherapy in cutaneous metastases from breast cancer. J Clin Oncol. 2001; 19:4150–4159.


9. Ackroyd R, Kelty C, Brown N, Reed M. The history of photodetection and photodynamic therapy. Photochem Photobiol. 2001; 74:656–669.


10. Lipson RL, Gray MJ, Baldes EJ. Haematoporphyria derivative for detection and management of cancer. In : Proceedings of the 9th International Cancer Congress; 1966. p. 393.
11. Gray MJ, Lipson R, Maeck JV, Parker L, Romeyn D. Use of hematoporphyrin derivative in detection and management of cervical cancer. Am J Obstet Gynecol. 1967; 99:766–771.


12. Rosenthal DI, Glatstein E. Clinical applications of photodynamic therapy. Ann Med. 1994; 26:405–409.


13. Sutedja TG, Postmus PE. Photodynamic therapy in lung cancer: a review. J Photochem Photobiol B. 1996; 36:199–204.


14. Xue LY, Chiu SM, Oleinick NL. Photochemical destruction of the Bcl-2 oncoprotein during photodynamic therapy with the phthalocyanine photosensitizer Pc 4. Oncogene. 2001; 20:3420–3427.


15. Saczko J, Chwiłkowska A, Kulbacka J, Berdowska I, Zieliński B, Drag-Zalesińska M, et al. Photooxidative action in cancer and normal cells induced by the use of photofrin in photodynamic therapy. Folia Biol (Praha). 2008; 54:24–29.
16. Moan J, Peng Q. An outline of the hundred-year history of PDT. Anticancer Res. 2003; 23(5A):3591–3600.
17. Ramakrishnan N, Clay ME, Friedman LR, Antunez AR, Oleinick NL. Post-treatment interactions of photodynamic and radiation-induced cytotoxic lesions. Photochem Photobiol. 1990; 52:555–559.


18. Ben-Hur E, Kol R, Marko R, Riklis E, Rosenthal I. Combined action of phthalocyanine photosensitization and gamma-radiation on mammalian cells. Int J Radiat Biol. 1988; 54:21–30.


19. Prinsze C, Penning LC, Dubbelman TM, VanSteveninck J. Interaction of photodynamic treatment and either hyperthermia or ionizing radiation and of ionizing radiation and hyperthermia with respect to cell killing of L929 fibroblasts, Chinese hamster ovary cells, and T24 human bladder carcinoma cells. Cancer Res. 1992; 52:117–120.
20. Kavarnos G, Nath R, Bongiorni P. Visible-light and X irradiations of Chinese hamster lung cells treated with hematoporphyrin derivative. Radiat Res. 1994; 137:196–201.


21. Wang J, Hyun W, Lamborn K, Deen DF. Measurement of radiation-induced damage in human glioma cells with flow cytometry. Cancer Res. 1996; 56:154–157.
22. Pass HI. Photodynamic therapy in oncology: mechanisms and clinical use. J Natl Cancer Inst. 1993; 85:443–456.


23. Fingar VH, Kik PK, Haydon PS, Cerrito PB, Tseng M, Abang E, et al. Analysis of acute vascular damage after photodynamic therapy using benzoporphyrin derivative (BPD). Br J Cancer. 1999; 79:1702–1708.


24. Dolmans DE, Kadambi A, Hill JS, Waters CA, Robinson BC, Walker JP, et al. Vascular accumulation of a novel photosensitizer, MV6401, causes selective thrombosis in tumor vessels after photodynamic therapy. Cancer Res. 2002; 62:2151–2156.
25. Busch TM, Wileyto EP, Emanuele MJ, Del Piero F, Marconato L, Glatstein E, et al. Photodynamic therapy creates fluence rate-dependent gradients in the intratumoral spatial distribution of oxygen. Cancer Res. 2002; 62:7273–7279.
26. Henderson BW, Dougherty TJ. How does photodynamic therapy work. Photochem Photobiol. 1992; 55:145–157.


27. Shumaker BP, Hetzel FW. Clinical laser photodynamic therapy in the treatment of bladder carcinoma. Photochem Photobiol. 1987; 46:899–901.


28. de Vree WJ, Essers MC, de Bruijn HS, Star WM, Koster JF, Sluiter W. Evidence for an important role of neutrophils in the efficacy of photodynamic therapy in vivo. Cancer Res. 1996; 56:2908–2911.
29. Korbelik M, Krosl G, Krosl J, Dougherty GJ. The role of host lymphoid populations in the response of mouse EMT6 tumor to photodynamic therapy. Cancer Res. 1996; 56:5647–5652.
30. Stukavec J, Horak L, Duchac V, Jirasek T, Rakusan J, Karaskova M, et al. Comparison of photodynamic therapy with phthalocyanine and photofrin in human colorectal carcinoma. Neoplasma. 2008; 55:127–129.