Abstract
Purpose
It has been demonstrated ellagic acid can inhibit tumor growth. However, the mechanism that elicits the anti-proliferative effect of ellagic acid is poorly understood. Our objective in this study was to evaluate the biological activity of ellagic acid by comparing the anti-proliferative effect and the apoptotic pathway of ellagic acid between the 2 human breast cancer cell lines.
Methods
The MCF-7 and MDA-MB-231 human breast cancer cell lines were used as cell models. The anti-proliferstive effect was evaluated by using a MTT assay. The cell cycle was analyzed by flow cytometry. Western blotting was performed to show the expressions of bcl-xL, cytochrome c, surviving, c-fos and pS2.
Results
The ellagic acid in the MDA-MB-231 cells showed significant anti-proliferative effects with dose dependent pattern. The anti-proliferative effects in MCF-7 cells were observed in only at a high concentration. Ellagic acid had no effect on the cell cycle in both breast cancer cells. In MDA-MB-231, the expression of bcl-xL was decreased with the decreasing concentration of ellagic acid. The expression of cytochrome c in the cytosol was increased with the decreased expression of bcl-xL. Ellagic acid also decreased the expression of survivin. In the MCF-7 cells, the expressions of bcl-xL and cytochrome c showed no change after treatment with ellagic acid even at a high dose. Ellagic acid was able to induce an up-regulation of c-fos and pS2 protein in MCF-7.
Conclusion
Ellagic acid has an anti-proliferative effect in the MDA-MB-231 cells. This effect of ellagic acid is through the intrinsic pathway in MDA-MB-231 cells. However, the expression of bcl-xL showed no change in the MCF-7 cells. Ellagic acid has a different anti-proliferative effect between the two human breast cancer cell lines.
Pomegranate (Punica granatum) is an ancient fruit that has been used as a medicine in many cultural areas, and nowadays it is widely consumed as fresh fruits or processed into such forms as juice, jam, and wine in west and asian countries.(1) It has anti-inflammatory effects, anti-oxidant effects, anti-angiogenic potential and anti-proliferative effects.(2-4) Epidemiologically, some reports showed that the extract of pomegranate has chemopreventive and anti-cancer effect on breast cancer.(5)
Pomegranate contains numerous phytochemicals such as ellagic acid, quercetin, kaempferol and luteolin glycosides.(6,7) Among these compounds, ellagic aicd has been demonstrated to have antitumor and anti-apoptotic effect in bladder cancer cells, prostate cancer cells, cervical cancer cells, colon cancer cells, oral cancer cells and leukemic cells.(4,8-10) Only a few investigators reported about the relation between ellagic acid and breast cancer. However the effect of ellagic acid on breast cancer is still not well understood.(6,11-12)
The mechanism by ellagic acid which elicits anti-proliferative effects still remains poorly understood, although it has been found that the apoptosis caused by ellagic acid is associated with p53/p21 expression, cell cycle arrest, regulating matrix metalloproteinases, vascular endothelial growth factor expression and platelet-derived growth factor.(9,13,14) Other studies demonstrated that ellagic acid provoked the induction of apoptosis via intrinsic pathway through bcl-xL down-regulation with mitochondrial release of cytochrome c into the cytosol.(9,15)
Our objective in this study was to evaluate the biological activity of ellagic acid by comparing the anti-proliferative effect and apoptotic pathway of ellagic acid between ER positive MCF-7 and ER negative MDA-MB-231 human breast cancer cell lines.
Ellagic acid was supplied from Calbiochem (San Diego, USA). Fetal bovine serum (FBS) was obtained from JRH Bioscience, Inc. (St. Lenexa, USA). Other chemicals, unless otherwise specified, were purchased from Sigma Chemical Co. (St. Louis, USA).
ER-positive MCF-7 and ER-negative MDA-MB-231 human breast cancer cells lines were obtained from the Korean Cell Link Bank (Seoul, Korea).
These were cultured in Dulbecco's modified Eagle's medium (DMEM) (Gibco BRL, Thessalonica, Greece) supplemented with 10% fetal bovine serum in an incubator with 5% CO2 under 37℃. For experiments, cells were cultured for 3 days in phenol red free DMEM (Gibco BRL, Thessalonica, Greece) and 5% (v/v) charcoal-dextran stripped FBS (CSS; Hyclone, Logon, USA) prior to use and they were maintained in the same media. Cell suspensions were diluted to a cell density of 5×105 cells/cm2 into a 96-well plate for 3-(4,5-dimethylthiazol-2-yl)-2,5-diphenyltetrazolium bromide (MTT) assay. For western blotting, cancer cells were cultured in 100-mm tissue culture Petri dishes. The following day, chemicals dissolved in DMSO were added. The final DMSO concentration did not exceed 0.3% (v/v). They were incubated in a 5% CO2 atmosphere and 100% humidity.
The MTT colorimetric assay was performed to identify tumor cell growth after treatment of each concentration (0, 10, 25, 50, 100, 250 µM) of ellagic acid. Plates were incubated in the presence of MTT dye for 4 hr. Mitochondrial dehydrogenase activity reduced the yellow MTT dye to a purple formazan, which was then solubilized with acidified isopropanol and absorbance was read at 570 nm on an enzyme-linked immunosorbent assay (ELISA) plate reader (Molecular Devices Inc., Union city, USA).
Cell growth was also assessed by cell count. Cells were plated in 100-mm tissue culture Petri dishes and were cultured by the method as mentioned above. After 24 hr of incubation, cells were dislodged from the vessel bottom by treatment with a trypsin-EDTA solution. After vigorous pipetting, the cell numbers were measured by counting under a light microscope using a Neubauer hemocytometer.
Cell cycle phase distribution was analyzed by flow cytometry (Becton-Dickinson Immunocytometry System, Franklin Lakes, USA). Cultures were incubated with each dose of ellagic acid for 24 hr and harvested. The cells were fixed in 70% ethanol at -20℃ overnight. Cells were washed twice with PBS and stained with PBS containing 40 µg/mL propidium iodide, 100 µg/mL RNase (Sigma, St. Louis, USA) and 0.1% triton x-100. After 30 min at 37℃ in the dark, the cells were analyzed with flow cytometry equipped with an argon laser at 488 nm. Distribution of cells in different phases of cell cycle was determined using a software program Modfit (Becton-Dickinson Immunocytometry System).
To harvest the cells, they were washed twice with PBS and scraped from culture dishes with lysis buffer containing 50 mM Tris-Cl (pH7.4), 150 mM NaCl, 1% Triton X-100, 0.1 mM DTT, 1mM EDTA, 1 mM PMSF and 1 µg/mL aprotinin. Cell lysates were centrifuged at 10,000×g for 10 min. After total protein extraction, 20 µg of proteins were separated by SDS-PAGE on 10% acrylamide Laemmli gels and transferred onto nitrocellulose membranes overnight. Membranes were blocked with the primary antibody for 90 min with 3% nonfat milk in PBS containing 0.5% Tween 20 and incubated for 90 min at room temperature with bcl-xL (R&D systems Inc., USA), survivin (Santa Cruz Biotechnology Inc., Santa Cruz, USA), pS2 (Santa Cruz Biotechnology Inc., Santa Cruz, USA) and c-fos (Calbiochem, San Diego, USA) antibodies. After incubation with peroxidase-conjugated secondary antibody for 90 min at room temperature, blots were developed using the ECL detection system (Amersham Biosciences, Uppsala, Sweden). The results of western blotting were standardized with the density of β-actin.
Mitochondria and cytosolic fractions were prepared using a Mitochondria fractionation kit (Active Motif, Carlsbad, USA) according to the manufacturer's recommendations and other investigators who used this kit.(16,17) Western blotting was performed using the above method with cytochrome c antibody (Santa Cruz Biotechnology Inc., Santa Cruz, USA).
The concentration-dependent effects of ellagic acid on proliferation of MBA-MB-231 and MCF-7 human breast cancer cell lines are shown in Figure 1. In MDA-MB-231 cell lines, ellagic acid showed significant anti-proliferative effects with dose dependent manner. In MCF-7 cells, the anti-proliferative effect was observed in only high concentration (more than 250 µM).
Figure 2 represents that anti-proliferative effect of ellagic acid on MDA-MB-231 cells is dose-time dependent pattern. However, there was no time dependent anti-proliferation effect in MCF-7 cells.
Cell count showed the same result with MTT assay (data is not shown).
The effects of ellagic acid on cell cycle in MDA-MB-231 cell line and MCF-7 cell line were determined using flow cytometry. The results for cell cycle analysis indicated that, during the 24 hr time period, ellagic acid has no influence on the change of cell cycle on both type of cell lines (Figure 3).
Ellagic acid decreased expression of bcl-xL and increased mitochondrial release of cytochrome c on MDA-MB-231 cells according to the increase of concentration. In addition, expression of survivin in MDA-MB-231 cells decreased with dose-dependent pattern.
In MCF-7 cells, expressions of bcl-xL and cytochrome c were not changed and expression of survivin was decreased with dose-dependent pattern. Although the expression of survivin in MCF-7 was decreased with stimulation of ellagic acid, the range of decrease was lesser than that in MDA-MB-231 (Figure 4).
It means that the anti-proliferative effect of ellagic acid appears dominantly through apoptotic pathway, especially decrease of bcl-xL and survivin, in MDA-MB-231 cells. Although decrease of expression of survivin was observed also in MCF-7, the stimulation of apoptotic pathway by ellagic acid was not enough to induce the anti-proliferative effect in MCF-7 cells.
Expression of c-fos and pS2 in MCF-7 cells after 48 hr of exposure to E2, ellagic acid and ICI182,780 were evaluated to investigate whether ellagic acid could stimulate ER or not. Ellagic acid was able to induce an up-regulation of c-fos and pS2 protein (Figure 5). It induced pS2 protein with 1.39-fold and c-fos with 1.51-fold more than E2. The expression of pS2 and c-fos by ellagic acid was blocked by co-treatment of cells with ICI182,780.
Ellagic acid inhibited proliferation of MDA-MB-231 cells, but the effect on the proliferation of MCF-7 cells was shown only in high concentration. These anti-proliferative effects of ellagic acid in two types of human breast cancer cell lines were very distinguishable. Anti-proliferation effect of ellagic acid has been studied in many kind of cancer cell lines although the exact mechanism has not been well investigated.(4,18-21) Many investigators reported that ellagic acid represents anti-proliferative activity through the induction of cell cycle arrest, apoptosis or antioxidant effects.(6,9,22)
Cell cycle arrest that is induced by ellagic acid is not definite and still controversial. Some studies showed that ellagic acid induced G0/G1 phase arrest in cervical epithelial carcinoma cell, leukemic cells, bladder cancer cells and colorectal adenocarcinoma cells.(9,23) Another studies reported that it caused S phase arrest.(15) The other investigator informed that ellagic acid has no significant influence on cell cycle.(9) In present study, cell cycle was not changed by ellagic acid in both MDA-MB-231 cells and MCF-7 cells.
Although the influence of ellagic acid on cell cycle is not definite, most of reports agreed that ellagic acid induces apoptosis in various types of cancer cells.(15,23) In general, two major pathways are involved in apoptosis. One is the mitochondrion-initiated pathway and the other is the cell surface death receptor pathway. A report showed that ellagic acid induces apoptosis via mitochondrial-initiated pathway in colon cancer cells. It showed that this phenomenon occurs through bcl-xL down-regulation with mitochondrial release of cytochrome c into the cytosol activation of initiator caspase 9 and effecter caspase 3.(15) Bcl-xL belongs to the antiapoptotic proteins of the bcl-2 family, and decrease of bcl-xL stimulates release of cytochrome c from mitochondria. The released cytochrome c activates caspase 3 and it induces apoptosis. In our study, the expression of bcl-xL in MDA-MB-231 was definitely decreased with the increase of concentration of ellagic acid. In addition, cytochrome c in cytosol increased with the decrease the expression of bcl-xL. However, expressions of bcl-xL and cytochrome c in MCF-7 cells were not changed after treatment of ellagic acid even in high dose. It seems that the expression of bcl-xL might have the relation with the different response of both cell lines to ellagic acid. Other studies also reported that down regulation of bcl-xL protein expression in various tumor cell lines resulted in activation of apoptosis and decreased cellular proliferation.(24,25)
The expression of survivin, which belongs to IAP family was also examined. IAP family can bind to the cell death proteases such as caspase 3 and caspase 7 and inhibits caspase activity and cell death in cells exposed to apoptotic stimuli.(26,27) In breast cancer, survivin is a significant predictor of survival in patients with poor prognostic breast cancer.(28) Our result presents that ellagic acid decreases survivin in both human breast cancer cell lines. This indicates that one of targets of ellagic acid is IAP family, although the process is not exactly same in both cells. Decreased amount of survivin expression were larger in MDA-MB-231 cell than that in MCF-7.
When we focused on breast cancer, there were only few studies of the role of ellagic acid. Recently, anti-angiogenesis effect of ellagic acid on breast cancer cell line was reported.(29) However, most of the papers were only about the chemoprevention effect of ellagic acid on breast cancer. Almost all investigators experienced using various cancer cell lines-such as colorectal cancer, prostate cancer or lung cancer. MCF-7 was only added as one of these cancer cell lines. We hoped to avoid the bias that ellagic acid is less effective compared with other kind of cancer, and studied the antiproliferative effect of ellagic acid on MDA-MB-231 and MCF-7 which have been used most commonly as breast cancer cell lines. The results showed that if we could find specific marker for ellagic acid, it could show superior effect on some portion of breast cancers.
The limitation of this study is the small number of cancer cell lines that were experienced. Even though MDA-MB-231 cells and MCF-7 cells are most frequently studied cell lines, they can not represent all characteristics of breast cancer. Experiences using various breast cancer cell lines might find the specific target of ellagic acid, and this pioneer study could be helpful to investigate this field.
To investigate whether the different effect of ellagic acid in these breast cancer cell lines has any relation with estrogen receptor, we studied the expression of c-fos and pS2 in MCF-7 cells treated with ellagic acid. c-fos and pS2 has been examined for sensitivity to ligand structure in MCF-7 cells.(30) Ellagic acid more powerfully stimulated the expression of c-fos and pS2 rather than E2 and these expressions were inhibited by ER antagonist, ICI 182,780. Putting these various result together, ellagic acid might have an anti-estrogenic effect in MCF-7 cells. Interestingly, although ellagic acid inhibits the cell proliferation induced by E2, expression of c-fos and pS2 treated with ellagic acid is much more increased than E2 treated one. Therefore, the effect of ellagic acid could be appeared mainly through ER in MCF-7.
Our results indicate that ellagic acid has anti-proliferative effect in MDA-MB-231 cells. This effect of ellagic acid appears through apoptotic pathway such as the decrease of expression of bcl-xL and decrease of expression of survivin that are members of IAP family. Although there was no definitive anti-proliferative effect in MCF-7 cells, suppression of expression of survivin were observed also. These data suggest that ellagic acid has different effects on each breast cancer cell line. If specific target of ellagic acid could be identified in breast cancer, ellagic acid may be used as a chemotherapeutic agent for the treatment of breast cancer.
Figures and Tables
Figure 1
The concentration-dependent effects of ellagic acid on proliferation of human breast cancer cell lines. Cells were exposed to different concentrations of ellagic acid for 24 hr. Then, cell proliferation was evaluated by MTT assay. The tests were performed in more than three times and the error bars designate the SEM. The asterisk indicates significant difference (p<0.05) compared to control cells (0 µM) by the Tukey test.
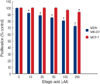
Figure 2
The concentration-dependent effects of ellagic acid on proliferation of human breast cancer cell lines. Cells were exposed to different concentrations of ellagic acid for 24 hr. Then, cell proliferation was evaluated by MTT assay. The tests were performed in more than three times and the error bars designate the SEM. An asterisk indicates significant difference (p<0.05) compared to control cells (0 µM) by the Tukey test. (A) MDA-MB-231 cell line (B) MCF-7 cell line.
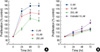
Figure 3
Effects of ellagic acid on cell cycle kinetics in human breast cancer cell lines. The tests were performed in more than three times and the error bars designate the SEM. (A) MDA-MB-231 cells, (B) MCF-7 cells.
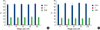
Figure 4
Effects of ellagic acid on expression of bcl-xL, cytochrome c and survivin in human breast cancer cell lines. Cells were cultured and treated with each dose ellagic acid for 24 hr. The asterisk indicates statistical significance (p<0.05) from control cells (0 µM) after 24 hr after treatment by Mann Whitney test. (A) Western blot analysis of bcl-xL upon treatment with ellagic acid. (B) Western blot analysis of cytochrome c release from mitochondria into the cytosol in MDA-MB-231 cells upon treatment with ellagic acid. (C) Western blot analysis of survivin upon treatment with ellagic acid.
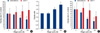
References
1. Gu L, Kelm MA, Hammerstone JF, Beecher G, Holden J, Haytowitz D, et al. Concentrations of proanthocyanidins in common foods and estimations of normal consumption. J Nutr. 2004. 134:613–617.


2. Kim ND, Mehta R, Yu W, Neeman I, Livney T, Amichay A, et al. Chemopreventive and adjuvant therapeutic potential of pomegranate (Punica Granatum) for human breast cancer. Breast Cancer Res Treat. 2002. 71:203–217.


3. Adams LS, Seeram NP, Aggarwal BB, Takada Y, Sand D, Heber D. Pomegranate juice, total pomegranate ellagitannins, and punicalagin suppress inflammatory cell signaling in colon cancer cells. J Agric Food Chem. 2006. 54:980–985.


4. Seeram NP, Adams LS, Henning SM, Niu Y, Zhang Y, Nair MG, et al. In vitro antiproliferative, apoptotic and antioxidant activities of punicalagin, ellagic acid and a total pomegranate tannin extract are enhanced in combination with other polyphenols as found in pomegranate juice. J Nutr Biochem. 2005. 16:360–367.


5. Setchell KD, Cassidy A. Dietary isoflavones: biological effects and relevance to human health. J Nutr. 1999. 129:758S–767S.


6. Papoutsi Z, Kassi E, Tsiapara A, Fokialakis N, Chrousos GP, Moutsatsou P. Evaluation of estrogenic/antiestrogenic activity of ellagic acid via the estrogen receptor subtypes ERalpha and ERbeta. J Agric Food Chem. 2005. 53:7715–7720.


7. Aggarwal BB, Shishodia S. Suppression of the nuclear factor-kappaB activation pathway by spice-derived phytochemicals: reasoning for seasoning. Ann N Y Acad Sci. 2004. 1030:434–441.


8. Saleem A, Husheem M, Harkonen P, Pihlaja K. Inhibition of cancer cell growth by crude extract and the phenolics of terminalia chebula retz. fruit. J Ethnopharmacol. 2002. 81:327–336.


9. Li TM, Chen GW, Su CC, Lin JG, Yeh CC, Cheng KC, et al. Ellagic acid induced p53/p21 expression, G1 arrest and apoptosis in human bladder cancer T24 cells. Anticancer Res. 2005. 25:971–979.
10. Mertens-Talcott SU, Percival SS. Ellagic acid and quercetin interact synergistically with resveratrol in the induction of apoptosis and cause transient cell cycle arrest in human leukemia cells. Cancer Lett. 2005. 218:141–151.


11. Smith WA, Freeman JW, Gupta RC. Effect of chemopreventive agents on DNA adduction induced by the potent mammary carcinogen dibenzo[a,l]pyrene in the human breast cells MCF-7. Mutat Res. 2001. 480-481:97–108.


12. Maciel ME, Castro GD, Castro JA. Inhibition of the rat breast cytosolic bioactivation of ethanol to acetaldehyde by some plant polyphenols and folic acid. Nutr Cancer. 2004. 49:94–99.


13. Losso JN, Bansode RR, Trappey A 2nd, Bawadi HA, Truax R. In vitro anti-proliferative activities of ellagic acid. J Nutr Biochem. 2004. 15:672–678.


14. Labrecque L, Lamy S, Chapus A, Mihoubi S, Durocher Y, Cass B, et al. Combined inhibition of PDGF and VEGF receptors by ellagic acid, a dietary-derived phenolic compound. Carcinogenesis. 2005. 26:821–826.


15. Larrosa M, Tomas-Barberan FA, Espin JC. The dietary hydrolysable tannin punicalagin releases ellagic acid that induces apoptosis in human colon adenocarcinoma Caco-2 cells by using the mitochondrial pathway. J Nutr Biochem. 2006. 17:611–625.


16. Bowes T, Singh B, Gupta RS. Subcellular localization of fumarase in mammalian cells and tissues. Histochem Cell Biol. 2007. 127:335–346.


17. Tondera D, Santel A, Schwarzer R, Dames S, Giese K, Klippel A, et al. Knockdown of MTP18, a novel phosphatidylinositol 3-kinase-dependent protein, affects mitochondrial morphology and induces apoptosis. J Biol Chem. 2004. 279:31544–31555.


18. Stearns V, Coop A, Singh B, Gallagher A, Yamauchi H, Lieberman R, et al. A pilot surrogate end point biomarker trial of perillyl alcohol in breast neoplasia. Clin Cancer Res. 2004. 10:7583–7591.


19. Wagner JE, Huff JL, Rust WL, Kingsley K, Plopper GE. Perillyl alcohol inhibits breast cell migration without affecting cell adhesion. J Biomed Biotechnol. 2002. 2:136–140.


20. Nhan S, Anderson KE, Nagamani M, Grady JJ, Lu LJ. Effect of a soymilk supplement containing isoflavones on urinary F2 isoprostane levels in premenopausal women. Nutr Cancer. 2005. 53:73–81.


21. Nikander E, Metsa-Heikkila M, Tiitinen A, Ylikorkala O. Evidence of a lack of effect of a phytoestrogen regimen on the levels of C-reactive protein, E-selectin, and nitrate in postmenopausal women. J Clin Endocrinol Metab. 2003. 88:5180–5185.


22. Larrosa M, Gonzalez-Sarrias A, Garcia-Conesa MT, Tomas-Barberan FA, Espin JC. Urolithins, ellagic acid-derived metabolites produced by human colonic microflora, exhibit estrogenic and antiestrogenic activities. J Agric Food Chem. 2006. 54:1611–1620.


23. Narayanan BA, Re GG. IGF-II down regulation associated cell cycle arrest in colon cancer cells exposed to phenolic antioxidant ellagic acid. Anticancer Res. 2001. 21:359–364.
24. Kuo PL, Hsu YL, Kuo YC, Chang CH, Lin CC. The anti-proliferative inhibition of ellipticine in human breast MDA-MB-231 Cancer cells is through cell cycle arrest and apoptosis induction. Anticancer Drugs. 2005. 16:789–795.


25. Xiao D, Vogel V, Singh SV. Benzyl isothiocyanate-induced apoptosis in human breast cancer cells is initiated by reactive oxygen species and regulated by Bax and Bak. Mol Cancer Ther. 2006. 5:2931–2945.


26. Ka H, Hunt JS. Temporal and spatial patterns of expression of inhibitors of apoptosis in human placentas. Am J Pathol. 2003. 163:413–422.


27. Tamm I, Wang Y, Sausville E, Scudiero DA, Vigna N, Oltersdorf T, et al. IAP-family protein survivin inhibits caspase activity and apoptosis induced by Fas (CD95), Bax, caspases, and anticancer drugs. Cancer Res. 1998. 58:5315–5320.
28. Li QX, Zhao J, Liu JY, Jia LT, Huang HY, Xu YM, et al. Survivin stable knockdown by siRNA inhibits tumor cell growth and angiogenesis in breast and cervical cancers. Cancer Biol Ther. 2006. 5:860–866.

