Abstract
Influenza is a worldwide public health concern. Since the introduction of trivalent influenza vaccine in 1978, vaccination has been the primary means of prevention and control of influenza. Current influenza vaccines have moderate efficacy, good safety, and acceptable tolerability; however, they have unsatisfactory efficacy in older adults, are dependent on egg supply for production, and are time-consuming to manufacture. This review outlines the unmet medical needs of current influenza vaccines. Recent developments in influenza vaccines are also described.
Influenza viruses contain eight single-stranded, negative-sense RNA segments, and are members of the family Orthomyxoviridae. Influenza viruses are classified as type A, B, or C [1]; among these three types, types A and B cause annual influenza epidemics in humans. Based on the hemagglutinin (HA) subtype, influenza A viruses are further divided into two phylogenetic groups: group 1 (H1, H2, H5, H6, H8, H9, H11, H12, H13, H16, H17, and H18) and group 2 (H3, H4, H7, H10, H14, and H15) (Fig. 1). Influenza B viruses are divided into two antigenically distinct lineages: Victoria lineage and Yamagata lineage [2].
Since the discovery of human influenza type A virus in 1933 [3] and type B in 1940 [4], influenza viruses have been considered major causative pathogens of medically attended acute febrile respiratory illnesses in humans. Antigenic variation is a unique feature of influenza viruses, and is responsible for influenza being an ongoing public health concern. Antigenic variations occur primarily in the HA and neuraminidase (NA) genes. There are two types of antigenic variation: antigenic drift and antigenic shift.
Antigenic drift is a process of ongoing evolution of influenza viruses. It results from the accumulation of point mutations in the HA and NA genes, and occurs in both influenza A and B viruses [5]. Antigenic drift is responsible for the emergence of new viral strains causing seasonal influenza epidemics.
Antigenic shift is a less frequent but more substantial type of antigenic variation. It is defined as the emergence of a novel influenza virus including a new HA or both a new HA and a new NA that are immunologically distinct from those of the strains circulating in the human population in previous years [5]. Antigenic shift occurs through genetic reassortment between influenza A viruses from different host species. Antigenically novel viruses have the potential to cause pandemics because they may have intrinsic pathogenicity in humans and the population is usually immunologically naïve to its new surface antigens. Pandemic influenza could occur when a large population is immunologically susceptible to the novel virus, and the virus can easily and efficiently transmit among human.
During the last 100 years, there have been four influenza pandemics in 1918, 1957, 1968, and 2009 (Table 1). The 1918-1919 pandemic was the most severe, with mortality estimated to exceed 50 million people worldwide [9]. The number of A (H1N1)pdm09 respiratory deaths that occurred globally during the 2009 pandemic influenza was estimated to be between 123,000 and 203,000 in a recent modeling study [10].
In the northern hemisphere, annual influenza epidemics typically occur during the autumn and winter months. The global attack rate is estimated to be 5-10% in adults and 20-30% in children [11]. Most seasonal influenza cases are mild and self-limiting. Infection rate is highest among children [12]. However, complications and hospitalizations are more frequent among individuals aged ≥ 65 years, children aged < 2 years, and those with underlying medical conditions that confer higher risk for influenza-related complications [13]. In the latter group, influenza may cause aggravation of underlying comorbidities and death. Vaccination is the primary strategy used to prevent and control influenza.
In general, seasonal influenza vaccines are trivalent. Trivalent influenza vaccine (TIV) has been used since its introduction in 1978 [14]. In 2011, 620 million doses of seasonal TIV were produced globally [15]. TIV contains a mixture of three influenza viruses: one influenza A(H1N1) virus, one influenza A(H3N2) virus, and one influenza B virus. Each year, the World Health Organization (WHO) recommends the composition of influenza vaccines, based on the results of global influenza surveillance and the prediction of the strains most likely to circulate in the forthcoming influenza season (Fig. 2). The currently available influenza vaccines are the inactivated influenza vaccine (IIV) and live-attenuated influenza vaccine (LAIV).
There are three types of IIV: whole virus vaccines, split virus vaccines, and subunit vaccines. IIVs are typically split virus or subunit vaccines, and are produced from highly purified, egg-grown influenza viruses. The efficacies of influenza vaccines depend on various factors, primarily the age and underlying comorbidities of vaccinees and the degree of antigenic match between the vaccine and circulating influenza virus strains. The efficacy of IIV for the prevention of influenza is 66-86% in non-elderly adults when the circulating influenza virus strain matches the vaccine strain [19-22]. A meta-analysis performed in 2011 found that IIV efficacy against laboratory-confirmed influenza was 70% (95% confidence interval [CI], 55-80%) in adults when circulating influenza viruses and vaccine strains were matched [20]. In South Korea, the effectiveness of the influenza vaccine for the prevention of laboratory-confirmed influenza was 46.5-50.8% in individuals aged 19-49 years and 58.7-63.3% among those aged 50-64 years during the 2010-2011 influenza season [23].
The low effectiveness of influenza vaccine in the elderly is well described; the vaccine effectiveness against influenza-like illness was reported as 39% (95% CI, 35-43%), and the mean vaccine effectiveness against laboratory-confirmed diseases calculated from a meta-analysis was 49% (95% CI, 33-62%) [24]. However, receipt of influenza vaccine was associated with a reduction in the rate of hospitalization by 30-40% in elderly individuals [19, 25, 26]. The greatest benefit from influenza vaccination in older adults is a reduction in all-cause mortality. Vu et al. found that influenza vaccine effectiveness against all-cause mortality among community-dwelling elderly persons was 50% (95% CI, 45-56%) [25].
Vaccine effectiveness can be affected by antigen mismatch. Vaccine mismatch can result in significant consumption of medical resources and socioeconomic burden. In the 1997-1998 influenza season, a poor match between vaccine antigen (A/Wuhan/359/59 (H3N2)) and circulating influenza virus (A/Sydney/05/97) was observed [27]. The influenza vaccine was associated with a 35-39% prevention in all-cause mortality in the 1997-1998 season, whereas the reduction was 60-61% in the 1996-1997 season (when the match was good) [28]. Although it is clear that antigenic drift can lead to reduced vaccine effectiveness, not all strains that have undergone antigenic drift elude vaccine-induced immunity in the population [27].
The LAIV is administered via the intranasal route, and the LAIV strains replicate in the epithelial cells of the nasopharynx. LAIV induce strain-specific IgA production, and these IgA responses are associated with protection against influenza illness [29]. LAIV was first licensed for use in the US in 2003 [30]. LAIV showed superior efficacy to non-adjuvanted TIV in children (aged 6 months to 18 years) [31]. In children aged 15-71 months, the efficacy of LAIV was 89-93% against culture-confirmed influenza, and the vaccinees had 16-33% fewer episodes of febrile otitis media [32-34].
However, in persons aged 17-49 years, LAIV is less efficacious. Comparative studies have proven that the efficacy of LAIV is similar or inferior to that of TIV [31]. The decreased efficacy of LAIV in adults could be due to past exposure to similar influenza viruses. Acquired, cross-reactive immunity limits replication of the attenuated virus administered via the intranasal route in some adults [35].
LAIV was approved for use in healthy non-pregnant individuals aged 2-49 years. LAIV is not indicated in young children who are under 2 years of age because of the risk of wheezing [36]. Safety data on LAIV for individuals with underlying comorbidities that confer higher risk for influenza complications are limited.
Current influenza vaccines have shown moderate efficacy, good safety, and acceptable tolerability. However, IIVs do not meet all medical needs (Table 2). LAIV is available only for healthy individuals aged 2-49 years. There is a clear need for egg-free, rapidly produced, safe, efficacious, and broadly protective influenza vaccines.
Several strategies for the development of better influenza vaccines have been introduced recently. The goals of these strategies are to enhance vaccine immunogenicity, broaden vaccine-induced immunity, establish alternative methods for the rapid production of vaccines, and improve acceptability and delivery of influenza vaccines. New and recently approved influenza vaccines are described below.
The essential role of adjuvants is dose sparing, enabling a rapid and broad immune response and expansion of the antibody response [37]. Using an adjuvant is one of the best methods of enhancing the performance of TIV, especially for older adults.
The first generation of adjuvanted influenza vaccines was introduced in the 1950s, and formulations included the emulsification of antigens with mineral oil [38, 39]. These vaccines induced greater and more sustained antibody responses and enabled antigen sparing [40-42]. However, mineral oil adjuvanted vaccines also induced unwanted local reactions such as cysts and sterile abscesses [43].
Aluminum salts have been widely used as adjuvants [44]. It is thought that the mode of action of aluminum salts is through the increased attraction and uptake of antigen presenting cells and activation of the inflammasome complex with increased efficiency of the innate immunity [45]. Aluminum salts induce a profound Th2 response [46]. However, they are less effective in evoking Th1 cell-mediated immunity.
TIV containing oil-in-water emulsion was first licensed in Europe in 1997. MF59 is a microfluidized, oil-in-water emulsion containing squalene. The oil is stabilized by adding a water-soluble surfactant (polysorbate 80, Tween 80) and an oil-soluble surfactant (sorbitan trioleate, Span 85) [47]. MF59 stimulates an influx of inflammatory cells and establishes a localized immunostimulatory environment [48]. Proliferation of antigen presenting cells and helper T cells increases the B cell response and enhances antibody production [48].
Fluad® (Novartis, Basel, Switzerland) is a seasonal MF59-adjuvanted IIV (aIIV) indicated for adults aged ≥ 65 years. The immunogenicity of MF59-aIIV has been evaluated in several studies. A meta-analysis has shown that elderly subjects with chronic diseases who received MF59-aIIV (compared with those who received IIV alone) had geometric mean hemagglutination inhibition titer (GMT) ratios of > 1.0 against the H3N2 antigen, suggesting that MF59-aIIV possessed enhanced immunogenicity [49]. Other studies have shown that MF59-aIIV can induce greater immune responses than conventional TIV in older adults, although immune responses vary according to strain and influenza season [50, 51]. MF59-aIIV can confer cross-protection against heterovariant strains in the elderly [52-54]. MF59-aIIVs have also shown superior vaccine effectiveness against laboratory confirmed influenza in the elderly, and have led to 25% reductions in the risk of hospitalization for influenza or pneumonia compared with nonadjuvanted IIVs [55, 56]. During the 7 days post-immunization, rates of local reactions were higher in MF59-aIIV recipients than in non-adjuvanted IIV recipients; however, these local reactions were mostly mild [51]. In South Korea, Fluad® (Novartis) was introduced in 2009 and has been used in persons 65 years of age and older. It has shown superior long-term immunogenicity in older adults compared with non-adjuvanted IIV [57].
Another oil-in-water emulsion, AS03, contains α-tocopherol, squalene and polysorbate 80 [45]. In a phase 3 randomized trial comparing AS03-aIIV with non-adjuvanted IIV in elderly individuals, a significant increase in efficacy was observed for AS03-aIIV against influenza A/H3N2 (relative efficacy 22.0%, 95% CI, 5.68-35.49%) [58]. Reductions in rates of all-cause death and pneumonia were also found with AS03-aIIV [58].
Skin is an attractive target for influenza vaccine delivery. The epidermis and dermis contain abundant antigen presenting cells, Langerhans cells, and dermal dendritic cells [59]. Intradermal (ID) immunization triggers antigen migration through lymphatic ducts and consequent stimulation of resident dendritic cells. Activation and migration of dermal dendritic cells lead to potent T cell activation [60].
The major objectives of the introduction of ID vaccines are dose sparing and improvement of immune response. ID influenza vaccines induce similar immune responses, at lower antigen doses, in healthy adults and patients with comorbidities [61-64]. In the elderly, ID influenza vaccines showed improved immunogenicity compared with conventional IIV at full antigen doses [65]. Studies of the tolerability and safety of ID influenza vaccines have shown the incidence of systemic events to be comparable in ID influenza vaccine recipients and conventional IIV recipients, whereas local reactions were frequently observed in ID influenza vaccinees [65-67]. Most of these local reactions were transient.
The first available ID influenza vaccine, Intanza® (Sanofi Pasteur, Lyon, France), also known as IDflu® (Sanofi Pasteur), was licensed in 2009 as a seasonal influenza vaccine in Europe (9 µg HA per strain for adults aged 18-59 years; 15 µg HA per strain for adults aged ≥ 60 years) [68, 69]. Fluzone® Intradermal (Sanofi Pasteur) containing 9 µg HA per strain was licensed in the US for use in adults aged 18-64 years in 2011 [70]. In South Korea, IDflu® 15 µg (Sanofi Pasteur) and IDflu® 9 µg (Sanofi Pasteur) were approved in 2010 for use in persons aged ≥ 60 years and 18-59 years, respectively.
High-dose (HD) influenza vaccine contains 60 µg of HA for each influenza strain, whereas the standard dose (SD) influenza vaccine contains 15 µg of HA for each strain. Several studies have documented that HD vaccine is more immunogenic than SD influenza vaccine in the elderly [71]. A randomized, controlled, double-blind phase 3 trial showed that HD influenza vaccine evoked a significantly stronger antibody response than SD vaccine [71]. The enhanced immunogenicity induced by HD vaccine was documented in persons aged ≥ 70 years and patients with cardiopulmonary disease. In a recently published randomized, controlled, phase 2 trial comparing the immunogenicity and safety of HD and ID vaccines, the HD vaccine was more immunogenic than the ID vaccine in individuals aged ≥ 65 years [72]. In HIV-infected persons older than 18 years, those vaccinated with HD vaccine had higher levels of seroprotection against H1N1 and influenza B than those vaccinated with the SD influenza vaccine [73]. Local reactions developed more frequently in recipients of the HD vaccine than in those of the SD vaccine [74]. However, HD vaccine was well-tolerated in the elderly [72, 75].
The US Food and Drug Administration (FDA) approved Fluzone® (Sanofi Pasteur) High Dose (Sanofi Pasteur) in 2009 for the prevention of influenza in persons aged ≥ 65 years [74]. HD influenza vaccine has not been approved for use in South Korea.
Cell-culture technologies provide a number of advantages over egg-based methods for manufacturing influenza vaccines. Cell-culture influenza vaccine (CCIV) overcomes the dependency on chicken egg supply and the risk of egg contamination that exists in the manufacture of egg-based vaccines. Manufacture of CCIV is faster and can be more easily scaled up than the manufacture of egg-based vaccines. In addition, the initial purity of the vaccine is higher with CCIV. The introduction of CCIV would substantially contribute to an improvement in global stockpiles of pandemic influenza vaccines.
Several cell lines have been developed for influenza vaccine manufacture [76]. Currently, Madin Darvy Canine Kidney (MDCK) and Vero cells have been licensed for the production of influenza vaccine. In 2001, Solvay Pharmaceuticals Inc. received a license for Influvac® TC (Solvay Pharmaceuticals Inc. Brussels, Belgium), an influenza vaccine produced in MDCK cells, in The Netherlands. However, Influvac® TC (Solvay Pharmaceuticals Inc.) was not commercially distributed. In June 2007, Optaflu® (Novartis), a trivalent subunit MDCK CCIV was approved in Europe [77]. In the US, Flucelvax® (Novartis), a trivalent subunit IIV containing viruses propagated in MDCK cells, was approved in 2012 [13]. Optaflu® (Novartis) and Flucelvax® (Novartis) were approved for individuals from the age of 18 years. Celtura® (Novartis) is a monovalent, cell culture-derived, inactivated subunit vaccine containing A(H1N1)pdm09 virus with the adjuvant MF59 [78]. Celtura® (Novartis) has been licensed in Europe. Celvapan® (Baxter, Deerfield, IL) is a whole-virion, Vero cell-derived, inactivated pandemic influenza vaccine containing A(H1N1)pdm09 virus and was approved for persons aged ≥ 6 months in Europe. Preflucel® (Baxter) is a Vero cell-derived, split, inactivated vaccine. A double-blind, placebo-controlled, phase 3 trial of Vero cell-culture-derived influenza vaccine was performed in healthy adults (age 18-49 years) in the US [79, 80]. A PER.C6 cell-derived influenza vaccine is now in development. Studies have reported that CCIV has immunogenicity and safety comparable to those of egg-based influenza vaccines [78, 79, 81, 82].
Two antigenically distinct lineages of influenza B viruses, Yamagata lineage and Victoria lineage, have co-circulated since the late 1980s [83]. There is little or no cross-protection between these two influenza B lineages. Current TIVs contain only one influenza B virus antigen, representing one lineage. This leads to reduced influenza vaccine effectiveness especially when the predominant circulating influenza B virus does not match the vaccine strain. The dominant circulating influenza B lineage was different from the vaccine strain in 5 of the 10 influenza seasons in the US from 2001 to 2011 [84]. In Europe from 2003 to 2011, the predominant circulating lineage differed from the vaccine strain in 4 of 8 seasons, and the mismatch rate of influenza B samples was estimated to be 58% [84].
The primary advantage of quadrivalent influenza vaccine (QIV) is that it provides cover for the risk that the selected influenza B vaccine lineage does not match the circulating lineage. The WHO recommended the inclusion of a second influenza B vaccine strain in QIVs for the 2013-2014 season [16]. Studies have demonstrated that QIV provides non-inferior immunogenicity against three influenza vaccine strains compared with TIV [85-87]. QIV contributes to additional immune response against the added alternative influenza B lineage. The safety and reactogenicity of QIV have been shown to be generally consistent with those of TIV [85, 86].
A trivalent recombinant HA influenza vaccine, FluBlok® (Protein Sciences, Meriden, CT), was approved for individuals 18 through 49 years of age in 2013 [88]. For recombinant vaccines, the gene encoding the HA protein is cloned into baculovirus vectors [89]. HA proteins are expressed in the insect cell line infected with these vectors; there is no need to use eggs or live influenza viruses in this process.
FluBlok® (Protein Science) contains 45 µg of each of the three HA proteins, giving a total of 135 µg of HA [90]. In a randomized, placebo-controlled trial, the overall protective efficacy of FluBlok® (Protein Science) without regard to strains match against culture-confirmed influenza was 44.6% (95% CI, 18.8-62.6%) in healthy adults aged 18-49 years [91]. FluBlok® (Protein Science) recipients more frequently experienced local injection site pain and muscle aches than placebo recipients; however, reported pain was rated as mild for 94% of events [91].
A number of approaches are being investigated to develop advanced influenza vaccines (Fig. 3). The virus-like particle influenza vaccine contains HA, NA, and influenza matrix 1 (M1) protein. In this process, recombinant viral vectors that express HA, NA, and M1 protein are used to infect cells [89]. The expressed influenza proteins spontaneously form particles which are similar in structure to wild-type viruses. Replication of the recombinant viral vectors does not depend on the function of the expressed HA or NA [92].
Viral-vectored influenza vaccines use other viruses to deliver influenza HA proteins to the immune system. Influenza HA genes are cloned into vector viruses such as adenovirus, vaccinia virus, Newcastle disease virus, or baculovirus, to express HA protein [89]. Some recombinant systems may allow for faster and greater vaccine production than conventional methods [92]. However, concerns that preexisting immunity against viral vectors may interfere with vaccine efficacy need to be overcome.
DNA-based influenza vaccines are made by placing HA gene into a plasmid. Intramuscular injection of DNA-based vaccines induces transfection of host cells and consequent expression of the antigen in the host. DNA-based vaccines are stable and can be rapidly produced. Reports have indicated favorable performance of the DNA-based vaccines in animal models [93, 94]. However, their poor immunogenicity in humans suggests the need for further investigation and more clinical trials [95].
A universal vaccine is ideal for providing broad-spectrum immunity against multiple influenza viral strains. Several conserved regions of the influenza virus have been investigated as candidates for a universal vaccine. The highly conserved ectodomain of the M2 protein of influenza A viruses and a stalk domain of HA have been explored [96]. T cell epitope-based vaccines using nucleoprotein and M1 protein have also been tested [97]. A T cell vaccine containing multiple T cell epitopes against influenza proteins has also been studied [98].
Current egg-derived influenza vaccines are effective and safe in the prevention and control of influenza. However, we need to improve upon IIVs in terms of strength and longevity of immunogenicity, range of cross-protection, and the time and process required for manufacture. It is evident that the currently available influenza vaccines are not optimal, especially in older adults.
Recently, new influenza vaccines including adjuvanted vaccines, CCIVs, and QIVs have been introduced, providing options for solving the remaining clinical challenges. Novel platforms for viral growth, such as cell-culture systems, may resolve the problems related to the pandemic influenza vaccine production in terms of response time, capacity, and efficiency. Research and development of future influenza vaccines with novel technologies will lead to the production of universal influenza vaccines with broad, potent, and persistent protective immunogenicity.
Figures and Tables
Figure 1
Phylogenetic tree of hemagglutinin (HA) of influenza A and B viruses. Phylogeny was generated based on amino acid sequences of HA of influenza A (H1-H18) and B viruses. Representative viruses were chosen in GenBank and sequence alignment was performed using Clustal W. MEGA 5 software was used to construct the phylogenetic tree.
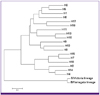
Figure 2
WHO recommendations on the composition of influenza vaccines from 1978-1979 to 2013-2014 for the northern hemisphere [16-18].
For influenza B viruses, orange-colored bars indicate B/Yamagata lineage viruses, blue-colored bars B/Victoria lineage viruses, and gray-colored bars influenza B viruses with unknown lineage.
aA(H3N2) virus antigenically similar to the cell-propagated prototype virus A/Victoria/361/2011. bFor the quadrivalent influenza vaccines containing two influenza B viruses.
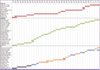
Figure 3
Novel approaches to the development of new influenza vaccines. Brief diagrams for the process to produce virus-like particle influenza vaccines (A), viral-vectored influenza vaccines (B), and DNA-based influenza vaccines (C) are presented.
HA, Hemagglutinin; NA, neuraminidase; M1, matrix 1 protein.
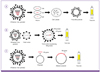
Table 3
Quadrivalent influenza vaccines recently approved by the US Food and Drug Administration [13]
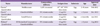
References
1. Hilleman MR. Realities and enigmas of human viral influenza: pathogenesis, epidemiology and control. Vaccine. 2002; 20:3068–3087.


2. McCullers JA, Saito T, Iverson AR. Multiple genotypes of influenza B virus circulated between 1979 and 2003. J Virol. 2004; 78:12817–12828.


3. Smith W, Andrewes CH, Laidlaw PP. A virus obtained from influenza patients. Lancet. 1933; 2:66–68.


6. Dawood FS, Iuliano AD, Reed C, Meltzer MI, Shay DK, Cheng PY, Bandaranayake D, Breiman RF, Brooks WA, Buchy P, Feikin DR, Fowler KB, Gordon A, Hien NT, Horby P, Huang QS, Katz MA, Krishnan A, Lal R, Montgomery JM, Mølbak K, Pebody R, Presanis AM, Razuri H, Steens A, Tinoco YO, Wallinga J, Yu H, Vong S, Bresee J, Widdowson MA. Estimated global mortality associated with the first 12 months of 2009 pandemic influenza A H1N1 virus circulation: a modelling study. Lancet Infect Dis. 2012; 12:687–695.


7. Programme WHOGI. Pandemic influenza preparedness and response: a WHO guidance document: World Health Organization. 2009.
8. Team DPIP. UK Influenza pandemic preparedness strategy. 2011.
9. Kageyama S. Pandemic influenza: a never-ending story. Yonago Acta Med. 2011; 54:41–48.
10. Simonsen L, Spreeuwenberg P, Lustig R, Taylor RJ, Fleming DM, Kroneman M, Van Kerkhove MD, Mounts AW, Paget WJ. GLaMOR Collaborating Teams. Global Mortality Estimates for the 2009 Influenza Pandemic from the GLaMOR Project : A Model ing Study. PLoS Med. 2013; 10:e1001558.
11. WHO. Vaccines: Influenza. Accessed 19 November 2013. Available at: http://www.who.int/biologicals/vaccines/influenza/en/.
12. Terebuh P, Uyeki T, Fukuda K. Impact of influenza on young children and the shaping of United States influenza vaccine policy. Pediatr Infect Dis J. 2003; 22:10 Suppl. S231–S235.


13. Centers for Disease Control and Prevention (CDC). Prevention and control of seasonal influenza with vaccines. Recommendations of the Advisory Committee on Immunization Practices--United States, 2013-2014. MMWR Recomm Rep. 2013; 62:1–43.
14. Hannoun C. The evolving history of influenza viruses and influenza vaccines. Expert Rev Vaccines. 2013; 12:1085–1094.


15. Partridge J, Kieny MP. Global production capacity of seasonal influenza vaccine in 2011. Vaccine. 2013; 31:728–731.


16. WHO. WHO recommendations on the composition of influenza virus vaccines. Accessed 19 November 2013. Available at: http://www.who.int/influenza/vaccines/virus/recommendations/en/.
17. Richard SA, Viboud C, Miller MA. Evaluation of Southern Hemisphere influenza vaccine recommendations. Vaccine. 2010; 28:2693–2699.


18. Influenza research database. World health organization recommendations for composition of influenza vaccines. Accessed 16 December 2013. Available at: http://www.fludb.org/brc/vaccineRecommend.do?decorator=influenza.
19. Nichol KL. Efficacy and effectiveness of influenza vaccination. Vaccine. 2008; 26:Suppl 4. D17–D22.


20. DiazGranados CA, Denis M, Plotkin S. Seasonal influenza vaccine efficacy and its determinants in children and non-elderly adults: a systematic review with meta-analyses of controlled trials. Vaccine. 2012; 31:49–57.


21. Jefferson TO, Rivetti D, Di Pietrantonj C, Rivetti A, Demicheli V. Vaccines for preventing influenza in healthy adults. Cochrane Database Syst Rev. 2007; CD001269.
22. Edwards KM, Dupont WD, Westrich MK, Plummer WD Jr, Palmer PS, Wright PF. A randomized controlled trial of cold-adapted and inactivated vaccines for the prevention of influenza A disease. J Infect Dis. 1994; 169:68–76.


23. Choi WS, Noh JY, Seo YB, Baek JH, Lee J, Song JY, Park DW, Lee JS, Cheong HJ, Kim WJ. Case-control study of the effectiveness of the 2010-2011 seasonal influenza vaccine for prevention of laboratory-confirmed influenza virus infection in the Korean adult population. Clin Vaccine Immunol. 2013; 20:877–881.


24. Beyer WE, McElhaney J, Smith DJ, Monto AS, Nguyen-Van-Tam JS, Osterhaus AD. Cochrane re-arranged: Support for policies to vaccinate elderly people against influenza. Vaccine. 2013; 31:6030–6033.


25. Vu T, Farish S, Jenkins M, Kelly H. A meta-analysis of effectiveness of influenza vaccine in persons aged 65 years and over living in the community. Vaccine. 2002; 20:1831–1836.


26. Nichol KL, Margolis KL, Wuorenma J, Von Sternberg T. The efficacy and cost effectiveness of vaccination against influenza among elderly persons living in the community. N Engl J Med. 1994; 331:778–784.


27. Carrat F, Flahault A. Influenza vaccine: the challenge of antigenic drift. Vaccine. 2007; 25:6852–6862.


28. Nordin J, Mullooly J, Poblete S, Strikas R, Petrucci R, Wei F, Rush B, Safirstein B, Wheeler D, Nichol KL. Influenza vaccine effectiveness in preventing hospitalizations and deaths in persons 65 years or older in Minnesota, New York, and Oregon: data from 3 health plans. J Infect Dis. 2001; 184:665–670.


29. Ambrose CS, Wu X, Jones T, Mallory RM. The role of nasal IgA in children vaccinated with live attenuated influenza vaccine. Vaccine. 2012; 30:6794–6801.


31. Ambrose CS, Levin MJ, Belshe RB. The relative efficacy of trivalent live attenuated and inactivated influenza vaccines in children and adults. Influenza Other Respir Viruses. 2011; 5:67–75.


32. Longini IM, Halloran ME, Nizam A, Wolff M, Mendelman PM, Fast PE, Belshe RB. Estimation of the efficacy of live, attenuated influenza vaccine from a two-year, multi-center vaccine trial: implications for influenza epidemic control. Vaccine. 2000; 18:1902–1909.


33. Belshe RB, Mendelman PM, Treanor J, King J, Gruber WC, Piedra P, Bernstein DI, Hayden FG, Kotloff K, Zangwill K, Iacuzio D, Wolff M. The efficacy of live attenuated, cold-adapted, trivalent, intranasal influenzavirus vaccine in children. N Engl J Med. 1998; 338:1405–1412.


34. Belshe RB, Gruber WC. Prevention of otitis media in children with live attenuated influenza vaccine given intranasally. Pediatr Infect Dis J. 2000; 19:5 Suppl. S66–S71.


35. Monto AS, Ohmit SE, Petrie JG, Johnson E, Truscon R, Teich E, Rotthoff J, Boulton M, Victor JC. Comparative efficacy of inactivated and live attenuated influenza vaccines. N Engl J Med. 2009; 361:1260–1267.


36. Prosser LA, Meltzer MI, Fiore A, Epperson S, Bridges CB, Hinrichsen V, Lieu TA. Effects of adverse events on the projected population benefits and cost-effectiveness of using live attenuated influenza vaccine in children aged 6 months to 4 years. Arch Pediatr Adolesc Med. 2011; 165:112–118.


37. Reed SG, Orr MT, Fox CB. Key roles of adjuvants in modern vaccines. Nat Med. 2013; 19:1597–1608.


38. Salk JE, Laurent AM, Bailey ML. Direction of research on vaccination against influenza; new studies with immunologic adjuvants. Am J Public Health Nations Health. 1951; 41:669–677.


39. Salk JE, Laurent AM. The use of adjuvants in studies on influenza immunization. I. Measurements in monkeys of the dimensions of antigenicity of virus-mineral oil emulsions. J Exp Med. 1952; 95:429–447.
40. Herbert WJ, Selwyn S, Philp JR. Field trials of adjuvant and saline influenza vaccines. Br J Prev Soc Med. 1965; 19:97–100.


41. . Antibody responses and clinical reactions with saline and oil adjuvant influenza virus vaccines. Br Med J. 1955; 2:1229–1232.
42. Stuart-Harris CH. Adjuvant influenza vaccines. Bull World Health Organ. 1969; 41:617–621.
43. Davis DJ, Philip RN, Bell JA, Vogel JE, Jensen DV. Epidemiologic studies on influenza in familial and general population observations. Am J Hyg. 1961; 73:138–147.


47. Martin JT. Development of an adjuvant to enhance the immune response to influenza vaccine in the elderly. Biologicals. 1997; 25:209–213.


48. Tsai TF. Fluad®-MF59®-adjuvanted influenza vaccine in older adults. Infect Chemother. 2013; 45:159–174.


49. Banzhoff A, Nacci P, Podda A. A new MF59-adjuvanted influenza vaccine enhances the immune response in the elderly with chronic diseases: results from an immunogenicity meta-analysis. Gerontology. 2003; 49:177–184.


50. Squarcione S, Sgricia S, Biasio LR, Perinetti E. Comparison of the reactogenicity and immunogenicity of a split and a subunit-adjuvanted influenza vaccine in elderly subjects. Vaccine. 2003; 21:1268–1274.


51. Podda A. The adjuvanted influenza vaccines with novel adjuvants: experience with the MF59-adjuvanted vaccine. Vaccine. 2001; 19:2673–2680.


52. Ansaldi F, Canepa P, Parodi V, Bacilieri S, Orsi A, Compagnino F, Icardi G, Durando P. Adjuvanted seasonal influenza vaccines and perpetual viral metamorphosis: the importance of cross-protection. Vaccine. 2009; 27:3345–3348.


53. Del Giudice G, Hilbert AK, Bugarini R, Minutello A, Popova O, Toneatto D, Schoendorf I, Borkowski A, Rappuoli R, Podda A. An MF59-adjuvanted inactivated influenza vaccine containing A/Panama/1999 (H3N2) induced broader serological protection against heterovariant influenza virus strain A/Fujian/2002 than a subunit and a split influenza vaccine. Vaccine. 2006; 24:3063–3065.


54. Ansaldi F, Bacilieri S, Durando P, Sticchi L, Valle L, Montomoli E, Icardi G, Gasparini R, Crovari P. Cross-protection by MF59-adjuvanted influenza vaccine: neutralizing and haemagglutination-inhibiting antibody activity against A(H3N2) drifted influenza viruses. Vaccine. 2008; 26:1525–1529.


55. Van Buynder PG, Konrad S, Van Buynder JL, Brodkin E, Krajden M, Ramler G, Bigham M. The comparative effectiveness of adjuvanted and unadjuvanted trivalent inactivated influenza vaccine (TIV) in the elderly. Vaccine. 2013; 31:6122–6128.


56. Mannino S, Villa M, Apolone G, Weiss NS, Groth N, Aquino I, Boldori L, Caramaschi F, Gattinoni A, Malchiodi G, Rothman KJ. Effectiveness of adjuvanted influenza vaccination in elderly subjects in northern Italy. Am J Epidemiol. 2012; 176:527–533.


57. Song JY, Cheong HJ, Noh JY, Seo YB, Choi WS, Cho GJ, Hwang TG, Kim WJ. Long-term and cross-reactive immunogenicity of inactivated trivalent influenza vaccine in the elderly: MF59-adjuvanted vaccine versus unadjuvanted vaccine. J Med Virol. 2013; 85:1591–1597.


58. McElhaney JE, Beran J, Devaster JM, Esen M, Launay O, Leroux-Roels G, Ruiz-Palacios GM, van Essen GA, Caplanusi A, Claeys C, Durand C, Duval X, El Idrissi M, Falsey AR, Feldman G, Frey SE, Galtier F, Hwang SJ, Innis BL, Kovac M, Kremsner P, McNeil S, Nowakowski A, Richardus JH, Trofa A, Oostvogels L. Influence65 study group. AS03-adjuvanted versus non-adjuvanted inactivated trivalent influenza vaccine against seasonal influenza in elderly people: a phase 3 randomised trial. Lancet Infect Dis. 2013; 13:485–496.


59. Koutsonanos DG, Compans RW, Skountzou I. Targeting the skin for microneedle delivery of influenza vaccine. Adv Exp Med Biol. 2013; 785:121–132.


60. Nicolas JF, Guy B. Intradermal, epidermal and transcutaneous vaccination: from immunology to clinical practice. Expert Rev Vaccines. 2008; 7:1201–1214.


61. Durando P, Iudici R, Alicino C, Alberti M, de Florentis D, Ansaldi F, Icardi G. Adjuvants and alternative routes of administration towards the development of the ideal influenza vaccine. Hum Vaccin. 2011; 7:Suppl. 29–40.


62. Belshe RB, Newman FK, Cannon J, Duane C, Treanor J, Van Hoecke C, Howe BJ, Dubin G. Serum antibody responses after intradermal vaccination against influenza. N Engl J Med. 2004; 351:2286–2294.


63. Jo YM, Song JY, Hwang IS, Lee J, Oh SC, Kim JS, Kim SR, Kim WJ, Cheong HJ. Dose sparing strategy with intradermal influenza vaccination in patients with solid cancer. J Med Virol. 2009; 81:722–727.


64. Beran J, Ambrozaitis A, Laiskonis A, Mickuviene N, Bacart P, Calozet Y, Demanet E, Heijmans S, Van Belle P, Weber F, Salamand C. Intradermal influenza vaccination of healthy adults using a new microinjection system: a 3-year randomised controlled safety and immunogenicity trial. BMC Med. 2009; 7:13.


65. Arnou R, Icardi G, De Decker M, Ambrozaitis A, Kazek MP, Weber F, Van Damme P. Intradermal influenza vaccine for older adults: a randomized controlled multicenter phase III study. Vaccine. 2009; 27:7304–7312.


66. Durando P, Alicino C, Alberti M, Sticchi L, Turello V, Marensi L, Caiazzo AL, Panico MG, Giugliano F, Parlato A, Peluso F, Sgricia S, Icardi G. Italian Intradermal Influenza Vaccine Working Group. Acceptance and safety of the intradermal influenza vaccine among the elderly in Italy: an on-field national study. Adv Ther. 2012; 29:312–326.


67. Marra F, Young F, Richardson K, Marra CA. A meta-analysis of intradermal versus intramuscular influenza vaccines: immunogenicity and adverse events. Influenza Other Respir Viruses. 2013; 7:584–603.


69. Duggan ST, Plosker GL. Intanza 15 mug intradermal seasonal influenza vaccine in older adults (aged ≥60 years): profile report. BioDrugs. 2010; 24:407–409.


70. Moro PL, Harrington T, Shimabukuro T, Cano M, Museru OI, Menschik D, Broder K. Adverse events after Fluzone® Intradermal vaccine reported to the Vaccine Adverse Event Reporting System (VAERS), 2011-2013. Vaccine. 2013; 31:4984–4987.


71. Falsey AR, Treanor JJ, Tornieporth N, Capellan J, Gorse GJ. Randomized, double-blind controlled phase 3 trial comparing the immunogenicity of high-dose and standard-dose influenza vaccine in adults 65 years of age and older. J Infect Dis. 2009; 200:172–180.


72. Tsang P, Gorse GJ, Strout CB, Sperling M, Greenberg DP, Ozol-Godfrey A, Diazgranados C, Landolfi V. Immunogenicity and safety of Fluzone® intradermal and high-dose influenza vaccines in older adults ≥65 years of age: A randomized, controlled, phase II trial. Vaccine. 2013; pii S0264-410X.


73. McKittrick N, Frank I, Jacobson JM, White CJ, Kim D, Kappes R, DiGiorgio C, Kenney T, Boyer J, Tebas P. Improved immunogenicity with high-dose seasonal influenza vaccine in HIV-infected persons: a single-center, parallel, randomized trial. Ann Intern Med. 2013; 158:19–26.


74. Sullivan SJ, Jacobson R, Poland GA. Advances in the vaccination of the elderly against influenza: role of a high-dose vaccine. Expert Rev Vaccines. 2010; 9:1127–1133.


75. DiazGranados CA, Dunning AJ, Jordanov E, Landolfi V, Denis M, Talbot HK. High-dose trivalent influenza vaccine compared to standard dose vaccine in elderly adults: safety, immunogenicity and relative efficacy during the 2009-2010 season. Vaccine. 2013; 31:861–866.


76. Hess RD, Weber F, Watson K, Schmitt S. Regulatory, biosafety and safety challenges for novel cells as substrates for human vaccines. Vaccine. 2012; 30:2715–2727.


77. Doroshenko A, Halperin SA. Trivalent MDCK cell culture-derived influenza vaccine Optaflu® (Novartis Vaccines). Expert Rev Vaccines. 2009; 8:679–688.


78. Reynales H, Astudillo P, de Vallière S, Hatz C, Schlagenhauf P, Rath B, Velentgas P, Fariña A, Sales-Carmona V, Groth N. A prospective observational safety study on MF59® adjuvanted cell culture-derived vaccine, Celtura® during the A/H1N1 (2009) influenza pandemic. Vaccine. 2012; 30:6436–6443.


79. Barrett PN, Berezuk G, Fritsch S, Aichinger G, Hart MK, El-Amin W, Kistner O, Ehrlich HJ. Efficacy, safety, and immunogenicity of a Vero-cell-culture-derived trivalent influenza vaccine: a multicentre, double-blind, randomised, placebo-controlled trial. Lancet. 2011; 377:751–759.


80. Chan CY, Tambyah PA. Preflucel®: a Vero-cell culture-derived trivalent influenza vaccine. Expert Rev Vaccines. 2012; 11:759–773.


81. Vinnemeier CD, Fischer-Herr J, Meyer S, Liebig K, Theeß W, Burchard GD, Cramer JP. Immunogenicity and safety of an inactivated 2012/2013 trivalent influenza vaccine produced in mammalian cell culture (Optaflu®): An open label, uncontrolled study. Hum Vaccin Immunother. 2013; [In press].
82. Frey S, Vesikari T, Szymczakiewicz-Multanowska A, Lattanzi M, Izu A, Groth N, Holmes S. Clinical efficacy of cell culture-derived and egg-derived inactivated subunit influenza vaccines in healthy adults. Clin Infect Dis. 2010; 51:997–1004.


83. Belshe RB. The need for quadrivalent vaccine against seasonal influenza. Vaccine. 2010; 28:Suppl 4. D45–D53.


84. Ambrose CS, Levin MJ. The rationale for quadrivalent influenza vaccines. Hum Vaccin Immunother. 2012; 8:81–88.


85. Kieninger D, Sheldon E, Lin WY, Yu CJ, Bayas JM, Gabor JJ, Esen M, Fernandez Roure JL, Narejos Perez S, Alvarez Sanchez C, Feng Y, Claeys C, Peeters M, Innis BL, Jain V. Immunogenicity, reactogenicity and safety of an inactivated quadrivalent influenza vaccine candidate versus inactivated trivalent influenza vaccine: a phase III, randomized trial in adults aged ≥18 years. BMC Infect Dis. 2013; 13:343.
86. McKeage K. Inactivated quadrivalent split-virus seasonal influenza vaccine (Fluarix® quadrivalent): a review of its use in the prevention of disease caused by influenza A and B. Drugs. 2013; 73:1587–1594.


87. Pépin S, Donazzolo Y, Jambrecina A, Salamand C, Saville M. Safety and immunogenicity of a quadrivalent inactivated influenza vaccine in adults. Vaccine. 2013; 31:5572–5578.


88. Goldenberg MM. Pharmaceutical approval update. P T. 2013; 38:150–152.
90. Yang LP. Recombinant trivalent influenza vaccine (Flublok®): a review of its use in the prevention of seasonal influenza in adults. Drugs. 2013; 73:1357–1366.


91. Treanor JJ, El Sahly H, King J, Graham I, Izikson R, Kohberger R, Patriarca P, Cox M. Protective efficacy of a trivalent recombinant hemagglutinin protein vaccine (Flu-Blok®) against influenza in healthy adults: a randomized, placebo-controlled trial. Vaccine. 2011; 29:7733–7739.


92. Dormitzer PR, Tsai TF, Del Giudice G. New technologies for influenza vaccines. Hum Vaccin Immunother. 2012; 8:45–58.


93. Ogunremi O, Pasick J, Kobinger GP, Hannaman D, Berhane Y, Clavijo A, van Drunen Littel-van den Hurk S. A single electroporation delivery of a DNA vaccine containing the hemagglutinin gene of Asian H5N1 avian influenza virus generated a protective antibody response in chickens against a North American virus strain. Clin Vaccine Immunol. 2013; 20:491–500.


94. Li J, Jiang Y, Zhao S, Chang X, Liu J, Zeng X, Li Y, Chen H. Protective efficacy of an H5N1 DNA vaccine against challenge with a lethal H5N1 virus in quail. Avian Dis. 2012; 56:4 Suppl. 937–939.

