Abstract
Influenza directly or indirectly contributes to the four leading causes of global mortality, at rates that are highest in older adults. As the proportion of older adults in the Korean population is greater than in most other countries, influenza prevention is a greater public health priority in Korea than elsewhere. Conventional inactivated influenza vaccine (IIV) is less immunogenic and efficacious (-50%) in older than in young adults, but adjuvanting the vaccine with oil-in-water emulsion MF59® increases immunogenicity, resulting in comparatively higher levels of hemagglutination inhibition antibodies and greater protection against all influenza, as well as cases requiring hospitalization. A recent observational study demonstrated that the adjuvanted vaccine protected older adults against influenza in a year when nonadjuvanted IIV was ineffective. In another multiyear study, the adjuvanted vaccine was estimated to be 25% more effective in preventing pneumonia and influenza hospitalizations compared to nonadjuvanted vaccine. Although MF59-adjuvanted vaccine is transiently more reactogenic than nonadjuvanted vaccine, there is no evidence that it increases risks for serious adverse events, including those with an autoimmune etiology. Experience thus far indicates a favorable balance of benefit to risk for MF59. This may reflect the adjuvant's mechanism of action in which the squalene oil emulsion increases antibody responses to co-administered antigen without acting more generally as an immunopotentiator.
In the 2010 Global Burden of Disease analysis of causes of death, ischemic heart disease, stroke, chronic obstructive pulmonary disease and lower respiratory tract infection were the four leading causes [1]. As acute influenza infections can exacerbate or can directly contribute to fatal outcomes for all of these conditions [2], especially among older adults, routine influenza vaccination with the use of improved vaccines could reduce mortality significantly on a global scale. Prioritizing influenza prevention is increasingly important as the number and proportion of older adults is growing in every region of the world.
In Korea, the life-expectancies of 85 years for women and 78 years for men in 2010 ranked among the longest among all countries (Fig. 1) [3]. Because of this longevity it is projected that the proportion of the national population that is over 65 years will increase from 11% in 2011 to 37% by 2050, when 14% of the population will be over 80 years old. As retirement from full or part-time work is progressively delayed, prevention of influenza in older adults has a consequence not only on the healthcare system but also on lost productivity. For example, a cost-benefit analysis of influenza and pneumococcal vaccination in Japan estimated that 20% of the population over 65 years old participated in the workforce, with an average of 153 hours worked per month [4].
These trends underscore the even greater imperative in Korea to implement effective protection of older adults against influenza. Unlike most other countries, compliance with vaccination among recommended groups is high in Korea, with vaccine coverage of older adults in the range of 95%. Thus, the principal obstacle to improved influenza prevention among the older Korean population is the relatively poor efficacy of the conventional vaccine.
Although trivalent inactivated influenza vaccines (IIV3) are recommended for older adults (usually defined as ≥ 65 years old) in nearly every country globally, the efficacy of these vaccines in this age group has increasingly been questioned [5, 6]. The efficacy of IIV was shown to be 50-60% in the only randomized, placebo-controlled trial in this age group, with doubts about efficacy in the oldest subjects in the study [7], while subsequent observational studies of vaccine effectiveness have been challenged on methodological grounds, and the vaccine's efficacy even in young adults has been claimed to be overestimated [5]. The relatively poor effectiveness of IIV in older vaccinees compared with younger adults is consistent with more numerous observations that consistently show a lower antibody response to vaccination with age due to immunosenescence [8, 9]. The immune system, as other physiological systems, changes and may decline with age, resulting in an impaired immune response to infection and to vaccination [10].
For these reasons, interest to improve influenza vaccines for the elderly, and indeed, for all age groups, has grown. Among the approaches to improve the performance of seasonal IIV is adjuvantation. In fact, an oil-in-water emulsion (MF59 adjuvanted IIV3 (IIVa3, Fluad) has been licensed for older adults in Europe since 1997 and its greater immunogenicity compared to nonadjuvanted IIV has been shown repeatedly [11, 12]. However, for ethical reasons it has not been possible to perform a placebo-controlled trial demonstrating efficacy in this age group. Observational studies have attempted to fill that gap and recent comparative studies have demonstrated the vaccine's higher effectiveness relative to conventional IIV. At the same time, large-scale use of MF59 in adjuvanted monovalent pandemic egg and cell-derived vaccines has provided extensive experience substantiating its safety in a more diverse population. This review summarizes recent experience on the use of MF59-adjuvanted IIV in older adults.
Fluad is an inactivated seasonal influenza vaccine comprising 15 µg of purified haemagluttinin (HA) surface antigen subunits from each of the three WHO-recommended strains formulated with MF59C.1 (containing in each 0.5 ml dose: squalene 9.75 mg, polysorbate 80 1.175 mg, sorbitan trioleate 1.175 mg, sodium citrate 0.66 mg, citric acid 0.04 mg and water for injection) [13]. Antibiotics (kanamycin and neomycin sulfate), formaldehyde and cetyltrimethylammonium bromide are used during the manufacturing process but thimerosal is not, so the vaccine is classified as thimerosal-free. Fluad contains no other preservatives.
Fluad is presented in a pre-filled syringe containing a single 0.5 mL dose to be administered intramuscularly. The vaccine is indicated for adults 65 years and older in more than 35 countries, including in Korea, and more than 65 million doses have been distributed since 1997. Within areas of Canada, Fluad is preferentially recommended over IIV for adults 65 years and older. The basis for this preferential recommendation is based on a body of data summarized below [14].
Numerous studies have shown that antibody responses of older adults to IIV are lower when compared with responses in younger adults [8-10]. It was to address this gap that IIVa3 was developed and licensed for use in adults over 65 years old. Trials conducted in the course of that registration and after licensure directly compared antibody responses to Fluad with an otherwise identical nonadjuvanted IIV counterpart. In general, those trials have shown that the geometric mean ratios (GMR) of hemagglutinin inhibition (HI) titer responses to IIVa compared with IIV are -1.5 fold higher, depending on the strain [12, 15]. Representative results are shown in Figure 2, with GMRs of IIVa:IIV above one indicating higher responses for IIVa.
As immune responses and so vaccine effectiveness (VE) continue progressively decline with advancing age beyond the conventional threshold of 65 years, it is pertinent that the comparatively higher response of IIVa has been shown to be maintained in ≥ 75 year olds, as demonstrated in a randomized trial comparing an nonadjuvanted split vaccine (Vaxigrip® Aventis Pasteur MSD, Lyon, France) with Fluad (Fig. 3) [16]. In these 'older elderly' subjects, both seroprotection and seroconversion rates were higher in the adjuvanted than non-adjuvanted vaccine recipients.
A recent study suggested that vaccine efficacy was diminished in persons who had been vaccinated in the previous season [17], although the consistency of this effect and its underlying immunological mechanisms remain to be elucidated [18]. However, as older adults in Korea are highly compliant with annual seasonal vaccination, it is noteworthy that in a meta-analysis of individuals receiving successive vaccinations across three seasons, the comparatively higher antibody responses to adjuvanted vaccine did not decline after repeated vaccination (Fig. 4) [12].
Antigenic mismatches between the virus strains contained in the vaccine and those that circulate during the seasonal epidemic can significantly reduce vaccine efficacy. Antigenic changes continually arise during ongoing evolution of the virus, due to point mutations in the HA gene of circulating strains or from intra-subtypic reassortant events, resulting in antigenic "drift" [19, 20]. A number of serological studies have shown that the antibody response to MF59-adjuvanted vaccine compared with nonadjuvanted vaccine is broadened, with antibody titers to heterovariant viruses that are quantitatively higher, whether measured by HI or by neutralization [14, 15]. Moreover, the adjuvanted antibody repertoire differs qualitatively, with an increased proportion of anti-HA antibodies directed at the HA1 and its receptor binding domain than to the antigenically less important stem region [21].
The results of a randomized study that demonstrated the reactivity of the adjuvanted antibody response to heterovariant strains that emerged years after the subjects were vaccinated illustrates the potential value of a broadened response. Older adult subjects were vaccinated with IIV or IIVa and their post-vaccination sera were stored. The sera were tested several years later against the A/Wyoming/3/2003 (H3N2) vaccine strain and against three H3N2 variants that had emerged 1-3 years in the interim [15]. Seroprotection levels are shown in Figure 5. The proportions of subjects receiving nonadjuvanted IIV who were seroprotected declined for strains that emerged 1, 2, and 3 years after vaccination, reflecting increasing vaccine mismatch due to antigenic drift. In sharp contrast, levels of seroprotection were maintained in IIVa recipients, to an extent that the adjuvanted 2003 vaccine would have met the European Committee for Medicinal Products for Human Use (CHMP) licensure criteria for seroprotection against the strains that emerged up to 3 years later. As the H3N2 subtype accounts for the greatest burden of hospitalizations and mortality of all the seasonal strains and viruses in that subtype also undergo a more rapid mutation and drift compared with seasonal H1N1 and B viruses, the greater level of seroprotection provided by IIVa against that subtype could have a commensurately greater impact on influenza-related morbidity and mortality.
The likelihood of antigenic mismatches between circulating strains and those designated in the WHO-recommended formulation may be greater in Korea than in other Northern Hemisphere locations such as the US [22]. Over a ten year period, the predominant strains and severity of the seasonal outbreak in Korea preceded observed patterns in the US by one year, resulting in a more frequent mismatch of strains in the recommended formulation with those circulating in Korea. Thus, seasonal strains circulating in Korea, like those future emerging strains described above, potentially could be better covered by an adjuvanted than a nonadjuvanted vaccine. The earlier circulation in Korea of viruses that later appeared in the US conforms to the hypothesis that seasonal A subtype viruses emerge first in Asia, whence they subsequently are transferred to Europe, North America and Australia [20, 23]. This model suggests that an adjuvanted vaccine could be well-suited for use throughout Asia.
Influenza morbidity and mortality increase sharply with advancing age, reflected in hospitalization rates of >150/100,000 in adults over 65 years of age (Fig. 6) [2, 24, 25]. Individuals in that age group represent half of those who are hospitalized for influenza-related illnesses during seasonal outbreaks and 90% of deaths, and nearly all have underlying chronic illnesses that predispose them to influenza-related complications (Fig. 7) [2, 24, 26]. A further personal and societal burden of influenza in this age group is the loss of mobility and ability for independent living in survivors of the illness [27-29]. Among nursing home residents, the capacity to undertake a major functional activity was lost in nearly 10% of patients after they had recovered from the acute phase of illness. This little studied impact of influenza in the elderly is consistent with other observations showing reductions in activities of daily living with hospitalization for other medical illnesses, ranging from ~25% - 60%, with advancing age beyond 70 years [28, 29].
While the need to demonstrate efficacy of improved vaccines in the older adult population is well recognized, only observational studies can be done to measure VE because, as mentioned previously, older adults are recommended to be vaccinated and placebo-controlled trials would be considered unethical. Despite the inherent limitations of observational studies, they have the advantage of representing real-life circumstances and with progressive refinements, independent studies across countries and centers have provided consistent results. For example, recent studies in Korea and in Tennessee, US disclosed similar (71.4-72.6%) effectiveness of IIV3 in preventing influenza-related hospitalizations, albeit in different seasons. These observations point to the possibility that vaccination could be more effective in preventing influenza-related complications than milder illnesses [30, 31].
Observational studies have assessed the absolute effectiveness of IIVa in preventing influenza or its complications in the contexts of community-acquired disease and in long term care facilities (LTCF), and also its comparative effectiveness versus nonadjuvanted vaccine.
In two case-control studies in Valencia, Spain, older adults vaccinated with IIVa were shown to be less likely than unvaccinated controls to be hospitalized for acute coronary syndrome (ACS), cerebrovascular accident (CVA) and pneumonia, with reductions for ACS of 87%, for CVA of 93% and for pneumonia of 69% [32, 33]. A case-control study conducted in Italy during the 2010-11 season compared patients hospitalized with influenza or pneumonia and matched non-hospitalized persons and found a non-significant VE for IIVa3 of 87.8% (0-98.9%) (34). However, these studies relied on administrative databases in which cases would not always have been laboratory-confirmed, which has been identified as a methodological shortcoming of administrative data.
Another case-control study conducted in the 2010-11 season in the previously mentioned Valencia community used laboratory test-negative patients as controls [35]. Patients hospitalized for influenza-related illnesses were tested by RT PCR for various respiratory viruses and 74% of those with influenza were infected with an H1N1 virus. Adjusted VE in the general adult population who received nonadjuvanted vaccine was 54% (11-76%). A similar level of effectiveness was observed in adults > 65 years old, 59% (16-79%), who received either a virosomal or MF59-adjuvanted vaccine. Vaccine effectiveness was maintained in older adults with underlying disease, 54% (4-78%). As the effectiveness of IIV generally is lower in older adults than in young adults, these observations suggested that the use of adjuvanted vaccine in the older population may have compensated for the usually lower VE of nonadjuvanted IIV. Other observational studies conducted during the same 2010-11 season generally disclosed similar point estimates of VE against the A (H1N1) strain in the total population, 46-66% [17, 36-40]. In one study that reported age-specific VE for adults > 65 years old, conventional IIV was ineffective against all circulating strains; however, that observation also may have reflected vaccine mismatches to viruses in other subtypes [40].
A prerequisite of observational studies is the need to adjust for differences between unvaccinated and vaccinated individuals that might confound their risk for the outcome of interest, in this case, for influenza-related complications. However, even with an adjusted analysis, concerns may remain over unidentified confounders. Observational studies comparing the relative effectiveness of adjuvanted versus nonadjuvanted vaccine remove some of these concerns and also provide data needed to make policy decisions on the choice of vaccine for public health programs.
The incremental benefit of IIVa compared with IIV in older adults was addressed in three observational studies: the first, a study of LTCF residents compared clinically-diagnosed influenza-like illness (ILI) in recipients of adjuvanted and nonadjuvanted vaccine; the second, a large scale, multi-season study compared rates of pneumonia and influenza hospitalizations based on administrative data, and the third, a smaller case-control study using laboratory test negative controls reported prevention of PCR-confirmed influenza as the endpoint.
The LTCF study, conducted in the 1998-1999 influenza season, included 3,173 institution-dwelling but ambulatory persons with a mean age of 85 years (± 10 years), residing in 25 facilities in Udine and Pordenone, Italy [41]. Overall, 93% of residents were vaccinated with either nonadjuvanted (N = 1,478) or adjuvanted (N = 1,487) vaccine. The overall effectiveness of any vaccination in preventing clinically-diagnosed ILI was 54%, with a VE for the MF59-adjuvanted vaccine of 94% (47-100%), and for the plain vaccine, 24.5% (0-45%). The relative effectiveness of the adjuvanted vaccine was even higher in subjects with physician-diagnosed chronic respiratory or cardiovascular disease than in the general LTCF population.
The cohort study was conducted over three consecutive influenza seasons starting in 2006 in Northern Italy (Lombardy Influenza Vaccine Effectiveness [LIVE] study) and enrolled 107,661 people aged 65 years of age and older who contributed 170,988 person-seasons of observation [42]. The primary objective was to assess the relative occurrence of pneumonia and influenza hospitalizations in recipients of adjuvanted or nonadjuvanted vaccine. The Italian National Healthcare System enabled linkage of hospital administrative data and immunization records, while additional data on subject functional status was assessed with a specific questionnaire. Multiple adjustments were made to reduce confounding, including the introduction of a propensity score. Recipients of adjuvanted vaccine tended to be older and were more likely to be chronically ill than IIV recipients, which was reflected in a 1.17 fold higher hospitalization rate in the summer period prior to the seasonal influenza epidemic. However, as the window of analysis was focused on the peak weeks of influenza transmission (determined from national laboratory-based surveillance), the risk ratio switched toward a lower risk of hospitalizations in IIVa recipients [risk ratio of 0.75 (95% CI 0.57 to 0.98)], indicating that IIVa was potentially 25% more effective than IIV in preventing pneumonia and influenza hospitalizations (Fig. 8). Although the study relied on administrative data and was not based on laboratory-confirmed outcomes, the trend of increasing risk reductions as the analysis window was tightened to remove 'background' cases unrelated to influenza supports the specificity of the estimated effect.
Because influenza-related cardiac hospitalizations and outpatient illnesses were not examined as endpoints, the incremental benefit of a 25% lower hospitalization rate is likely to underestimate the entire potential advantage of adjvuanted over unadjuvanted vaccine in this population.
In addition to adjuvanting IIV, protection can be extended by adding an additional B strain to the current vaccine, resulting in a quadrivalent or IIV4 vaccine. Viruses in co-circulating Yamagata and Victoria genetic lineages (named after the Yamagata/16/1988 and Victoria/2/1987 reference strains) exhibit limited cross-reactivity in naïve animals and in young children [43]. Several quadrivalent seasonal vaccines (both live and inactivated) are licensed or are in development. An adjuvanted IIV4 also is under development; however, in the interim, it is worth comparing the hypothetical impacts of nonadjuvanted IIV4 with IIVa3 (Fluad) in preventing overall influenza hospitalizations.
Influenza B viruses from the two lineages co-circulate with viruses in the other subtypes in widely varying proportions during seasonal outbreaks. In a recent review of 10 influenza seasons in the United States, the uncovered B strain contributed, on average, 9.2% towards the annual number of cases overall, but proportions varied from 0 to 98% in individual years [44]. Adding a fourth strain to cover these infections was projected to prevent only an additional 1.4% hospitalizations and deaths, in part because of the modest efficacy of the non-adjuvanted vaccine. In contrast, the adjuvanted trivalent vaccine is likely to prevent more morbidity on average, because the adjuvant augments protection against the more virulent H3N2 infections that account for more hospitalizations and deaths than all other subtypes combined. In a review of influenza-related hospitalizations in the US from 1993-2008, the age-specific rate of hospitalizations was 3.5-fold higher for H3N2 infections than for influenza B infections in ≥ 65 year olds, and was 2.5-fold higher in the general population (Table 1) [25]. Thus, a hypothetical 25% incremental effectiveness of IIVa3 over nonadjuvanted IIV4 against H3N2 infections would result in a greater reduction of all influenza-related hospitalizations, especially in older adults. The relative impact of introducing IIV4 versus IIVa3 in the elderly depends on the local epidemiology of H3N2 and B infections in individual countries, including the age-specific rates of illness for the two subtypes and their relative dominance across seasons.
In addition, it is important to recognize that adults respond even to nonadjuvanted IIV3 vaccination with cross-lineage antibody responses, probably reflecting priming due to prior exposures to viruses in both lineages over a lifetime (Table 2). The identification of epitopes common to viruses in the two lineages helps to explain their antigenic relatedness [45]. This cross-reactivity was reflected in results of a VE study during the 2007-2008 season when the vaccine was lineage-mismatched to 98% of the influenza B viruses that circulated, yet the B-specific VE of nonadjuvanted IIV3 was 55%, despite the mismatch [46].
In a second comparative effectiveness study, the Fraser Health department (British Columbia, Canada) sponsored a case-control study in three health districts that used either adjuvanted or nonadjuvanted split vaccine during the 2011-12 influenza season [47]. Cases and controls were drawn from patients whose upper respiratory swab sample results were, respectively, PCR-positive or -negative for influenza virus (laboratory test-negative control design). The study population included both community-dwelling older adults and those who were residents of LTCF. The study sought to enroll subjects over 75 years of age and the mean age of participants was 83 years (± 0.5 years) and 57% were LTCF residents.
Although the 2011-12 influenza season was relatively mild, the adjuvanted vaccine had an effectiveness of 60% in preventing laboratory-confirmed influenza while nonadjuvanted vaccine was ineffective. In a sub-analysis of community-dwelling subjects only, the effectiveness of adjuvanted vaccine was 73%, P = 0.03, while again, no effectiveness was shown for nonadjuvanted vaccine. Other VE studies conducted that year also found IIV to be poorly effective overall and ineffective in adults > 65 years old [48-51]. The results of this study were sufficiently persuasive that the Vancouver Coastal health authority issued a preferential recommendation for Fluad to be used in older adults over the other available non-adjuvanted IIV available in that health care system [14]. The second year of this study is ongoing.
The safety of the adjuvanted seasonal vaccine has been evaluated in elderly subjects in both clinical trials and post-marketing surveillance programs [10, 52, 53]. Together, this experience indicates that the vaccine's overall safety profile is similar to that of non-adjuvanted split-virion or subunit vaccines. The adjuvanted vaccine is transiently more locally reactogenic but is well tolerated.
A meta-analysis of safety data from > 10,000 elderly individuals vaccinated with IIVa3 in clinical trials demonstrated that the vaccine was well tolerated by older adult recipients, even after revaccination in subsequent influenza seasons [10]. Only local reactions such as pain, erythema and induration were reported significantly more frequently in individuals receiving IIVa3 compared with those receiving nonadjuvanted IIV, but the severity of the adverse events (AE) was mild or moderate in the great majority of cases and they were short-lived. Similarly, systemic reactions were infrequent and transient, ranging from < 1-8% for the adjuvanted vaccine and < 1-4% for nonadjuvanted comparator vaccines. Fever was not prominent among adjuvanted vaccine recipients. Although myalgias were reported more frequently in adjuvanted vaccine recipients, it is uncertain if subjects clearly differentiated local from generalized muscular pain. A similar pattern of slightly increased but clinically insignificant reactogencity of the adjuvanted compared to nonadjuvanted pandemic vaccine was noted in several clinical trials, including in Korea [52].
Spontaneous adverse events (AE) and serious AE (SAE) reports submitted to Novartis' pharmacovigilance were analysed over an interval in which an estimated 27-32 million doses of Fluad had been distributed [53]. That numerator-only analysis did not point to unusual rates for specified AEs of note, including Guillain-Barre syndrome and related neurological syndromes with a potential autoimmune etiology.
More detailed safety data are available from observations actively collected in clinical trials. Safety data were pooled from 64 clinical trials involving MF59-adjuvanted seasonal and pandemic influenza vaccines, comparing recipients of adjuvanted [(+) MF59] or nonadjuvanted [(-) MF59] vaccine counterparts. Safety outcomes were analyzed in the overall population and in subjects aged ≥ 65 years in all clinical trials, and separately for controlled trials only [54]. Data from 20,447 (+) MF59 and 7,526 (-) MF59 subjects were included. Overall, (+) MF59 subjects had lower risks than (-) MF59 subjects of experiencing any unsolicited AE (26.8% vs 39.2%; adjusted risk ratio [ARR] 0.65; 95% CI 0.60 to 0.70). All unsolicited AE, the new occurrence of chronic disease, cardiovascular disease, SAE, including hospitalizations and deaths, also were compared in MF59 adjuvanted-vaccine and nonajduvanted vaccine recipients (Fig. 10) [54]. The risk ratio of those events were similar or, in the case of all unsolicited AEs, new onset of chronic disease and cardiovascular disease, were lower in adjuvanted vaccine recipients compared to controls. The latter suggests an unanticipated observation that the adjuvanted vaccine may have helped protect against those outcomes, consistent with studies showing improved clinical effectiveness of the adjuvanted vaccine. Not shown on the figure, reports of autoimmune disease in the two groups did not differ in the two groups, with a risk ratio of 0.59 (0.23 to 1.55) [54].
Concern has been raised over the safety of adjuvanted vaccines to patients with autoimmune disease, although, for MF59-adjuvanted vaccines, the available data, though limited, have not suggested a risk. Among the > 65 million persons who have received IIVa3 since its licensure in 1997, it is highly probable that some patients with autoimmune disease (especially rheumatoid arthritis) would have received the vaccine but no signal of disease exacerbation has been noted. Furthermore, in the LIVE study described above, hospital admissions for Guillain-Barré syndrome and other adverse events of special interest (AESI) occurred at similar frequencies in recipients of adjuvanted and non-adjuvanted vaccines (unpublished observation). Although more limited in the number of subjects under observation, as noted previously, no significant risk for autoimmune disease exacerbation or new onset of chronic disease was seen in an analysis of the MF59 clinical trial database. A single study has been published on use of IIVa3 in patients with an autoimmune disease viz. a study in 60 children (mean age -8 years) with juvenile rheumatoid arthritis (JRA) [55]. Although that study aimed to discover the utility of the adjuvant in overcoming suboptimal immune responses in patients on TNF-alpha inhibitor therapy, the safety follow-up of those subjects over a 4 month interval reported no clinical exacerbations (except in one patient who was noncompliant with therapy) and no change in laboratory markers of JRA activity.
Although not directly pertinent to the safety of seasonal IIVa3, database studies of cell- and egg-derived MF59 adjuvanted pandemic vaccine recipients compared to unvaccinated persons disclosed no difference in risk for various safety outcomes that included some with an autoimmune etiology [56, 57]. In addition, during the pandemic, reports of autoimmune disorders within EudraVigilance (Pharmacovigilance in the European Economic Area by European Medicines Agency) were similar for nonadjuvanted pandemic vaccine and adjuvanted formulations that included MF59-adjuvanted subunit pandemic vaccines [58]. These observations are supplemented by passive reports from the 107 million doses of MF59-adjuvanted monovalent H1N1 vaccine that had been distributed in the pandemic response, although the number of doses actually administered and their use in various age groups are unknown. An analysis of spontaneously reported adverse events showed no difference in the distribution of spontaneously reported neurological and other AESIs, including Guillain-Barré syndrome and other autoimmune neurological conditions, for the adjuvanted compared with nonadjuvanted pandemic vaccine [59, 60]. In addition, no notable adverse event signals were reported in Argentina where only MF59-adjuvanted pandemic vaccine was used in the public health response and 10 million doses had been administered with high coverage of 5-59 year olds with risk factors [61].
Special interest has focused on the increased risk for narcolepsy, which is suspected to have an autoimmune etiology, following immunization with pandemic vaccine adjuvanted with AS03, another oil-in-water emulsion adjuvant [62, 63]. That elevated risk was observed in children and not in older adults, nevertheless, the observation is the first linking an adjuvant with onset of an autoimmune disease. No signal of an increased risk has been demonstrated thus far in connection with MF59-adjuvanted pandemic vaccines [64]. One vaccine-associated case meeting a Brighton Collaboration level 2 definition of narcolepsy was reported through pharmacovigilance but it is difficult to evaluate the significance of a single case against the background occurrence of narcolepsy. Other studies relying on passive surveillance cited above also did not disclose any cases. Active surveillance to discover cases was conducted in the Netherlands, where the MF59-adjuvanted vaccine was administered to an estimated 654, 885 individuals aged 5-50 years of age with influenza risk factors, including an estimated 154, 622 children aged 5-19 years of age. No narcolepsy cases associated with the MF59-adjuvanted pandemic vaccine were found, after an active search for cases in 16 sleep disorder referral centers [65].
The failure to detect an increased risk of narcolepsy associated with MF59-adjuvanted pandemic vaccine could have several explanations besides a true lack of association, including differences between the AS03 and MF59 adjuvants, between the viral antigens in the respective vaccines, as well as other differences in vaccine constituents. The unknown but potentially limited number of children who received the MF59-adjuvanted vaccine may have limited the ability to detect cases through passive surveillance systems, as the attributable risk associated with AS03-adjuvanted vaccine ranged from 1/16,000 to 1/50,000 doses [59]. In addition, the role of the pandemic infection itself is unclear as it was suspected to be associated with an increased frequency of narcolepsy cases in Beijing, though no signal was detected in Korea [66-68].
MF59, an oil-in-water emulsion produced by microfluidization (MF), was the first adjuvant to be used in a licensed seasonal influenza vaccine [13, 69]. Squalene, the oil in MF59, is a naturally occurring hydrocarbon extracted from livers of the dog-shark, a small, non-endangered species. The preponderance of shark-derived squalene is used in cosmetics manufacturing while its use in vaccines constitutes a very small fraction by comparison. A single dose of MF59-adjuvanted vaccine given once a year contains 10 mg of squalene, while humans synthesize 1000 mg of squalene daily in the liver as an intermediate in the biosynthesis of cholesterol, and also consume 50-200 mg daily in a typical diet. The oil is microfluidized into droplets stabilized by a water-soluble emulsifier (polysorbate 80-also known as Tween 80), and an oil-soluble emulsifier (sorbitan trioleate, also known as Span 85) (Fig. 11). The size of the droplets, circa 160 nm mean diameter, approximates the size of a large virion (the mean diameter of an influenza virion is -100 nm). MF59 can be filter-sterilized, is freely miscible with aqueous solutions, and the material is stable for at least 5 years. The combination of the MF59 adjuvant emulsion and inactivated viral subunits constitutes the seasonal influenza vaccine Fluad.
MF59 enhances the immune response to vaccine antigens both quantitatively (higher levels) and qualitatively, with differences in the profile of the elicited antibody response [69, 70]. After intramuscular inoculation, MF59 stimulates an influx of inflammatory cells, including granulocytes as well as monocytes and macrophages to the injection site (Fig. 12). Their release of chemokines, including CCL2, 3, 4, and IL18, attract further waves of inflammatory cells, establishing a localized immunostimulatory environment. Co-administered antigen at the site is actively taken up by monocytes while MF59 also induces the chemokine receptor CCR7 and increases their differentiation into dendritic cells and their migration into draining lymph nodes where they trigger the adaptive immune response specific to the vaccine antigen.
The resulting proliferation of antigen presenting cells and helper T cells then augment a B cell response and ultimately, increased production of antibodies. Antibodies elicited by MF59-adjuvanted influenza HA are more avid than those produced to HA alone, as demonstrated in ELISAs employing properly folded antigen and a chaotropic wash, and in surface plasmon resonance studies in which slower off-rates reflected an increased strength of antibody binding to antigen [21].
The individual components of MF59, including squalene, are not in themselves adjuvants. An adjuvant effect is seen only with the microfluidized formulation comprising all components. Antigen and MF59 must be presented at the same site for an adjuvant effect to be seen and presented either at the same time or with MF59 presented first, allowing the adjuvant to establish the immunostimulatory environment of immune-reactive cells that leads to increased uptake and processing of antigen. It has been shown that no physicochemical linkage of antigen to MF59 occurs or is required for the adjuvant effect. The adjuvant and antigen are cleared rapidly and with independent kinetics from the injection site itself-90% of the MF59 and 75% of antigen remain at 6h, and 5% and 0.05%, respectively, at 120 h. At 3 h, most of the MF59 and antigen are in an extracellular location, but by 48 h, both are largely intracellular.
The injection site transcriptome following intramuscular injection of MF59 overlaps with but can be differentiated from and is enlarged compared to the collection of genes that are upregulated after alum or CpG injection [71]. They comprise a broad array of genes associated with immune activation e.g. those encoding chemokines, cytokines and their receptors, adhesion molecules associated with leucocyte migration, and others associated with antigen presentation. Importantly, within the lymph node, MF59 by itself does not produce similar gene activation in an antigen-independent manner, in contrast to control immune potentiators (e.g. TLR7 agonist) that are themselves immunostimulatory. From a clinical safety perspective, the absence of generalized MF59-induced immune activation within the lymph node is desirable.
The specific cellular membrane effects, intracellular events and molecular targets of MF59 are still under investigation. Requirement for activation of the Nalp3 inflammasome that underlies other inflammatory reactions has been excluded while the adaptor molecule, MyD88, has been defined to be necessary for MF59-stimulated signalling [72].
Influenza is a leading cause of serious morbidity and mortality in older adults and also causes significant disability in surviving elderly patients. The proportion of the Korean population that is elderly is currently among the highest in the world and continues to grow. Routine influenza vaccine coverage among the older adult population in Korea is remarkably high, so improving protection against influenza-related complications in this age group necessitates the use of more effective vaccines. The effectiveness of conventional non-adjuvanted inactivated influenza vaccine in older adults - ~50%, is limited, but can be improved by the addition of the oil-in-water emulsion adjuvant, MF59. Recent observational studies in elderly adults have (1) estimated that the adjuvanted vaccine (Fluad) provided significant protection against influenza-related hospitalization, similar to that achieved by a nonadjuvanted vaccine in young adults; (2) that the adjuvanted vaccine was 60-70% effective against PCR-confirmed illness in a population > 75 years of age, during a season when nonadjuvanted vaccine was ineffective; and (3) in a large-scale study across three influenza seasons, that the adjuvanted vaccine may further reduced influenza and pneumonia hospitalizations by an estimated 25% above those prevented by nonadjuvanted vaccine. Although not immediately obvious, IIVa3 may prevent more morbidity than IIV4 because the former's incremental prevention of seasonally dominant H3N2-associated hospitalizations is likely to be greater than the small reduction in influenza B hospitalizations provided by nonadjuvanted quadrivalent vaccine.
These clinical outcomes are consistent with the higher antibody levels elicited by the MF59-adjuvanted vaccine compared to nonadjuvanted vaccine. HI antibody titers in response to the adjuvanted vaccine are higher and in addition, are more broadly reactive to antigenically mismatched strains. The latter property may be of even greater importance in Korea and other Asian countries than elsewhere because influenza A viruses have been observed to emerge first in Asia before they are transferred to the other regions, resulting in a greater chance of mismatch between vaccine strains and locally circulating strains.
The MF59-adjuvanted seasonal vaccine was first licensed in 1997 for adults over 65 years of age and more than 65 million doses have been distributed in more than 30 countries (Fluad is licensed for adults > 60 years old in some countries). Although the vaccine is somewhat more reactogenic than conventional nonadjuvanted inactivated vaccine, there has been no indication of increased safety risks. Use of the MF59-adjuvanted A/H1N1pdm09 vaccine in a much broader age range did not result in the emergence of novel safety signals in these populations, including an increased risk for onset of narcolepsy.
It is unknown if the composition and mechanism of action of MF59 is connected with the apparent absence of a narcolepsy risk for the MF59-adjuvanted pandemic vaccines. The adjuvant consists of microfluidized squalene oil, held by two surfactants in 160 nanometer diameter droplets within a citrate buffer. That emulsion increases antibody responses to co-administered antigen by amplifying the influx of inflammatory cells to the injection site and increasing the differentiation of incoming monocytes to antigen presenting cells and their migration to local lymph nodes. An amplified antibody response follows increased T and B cell activation within the lymph node, but importantly, the emulsion does not directly and generally activate the lymph node as an immunostimulant.
Figures and Tables
![]() | Figure 2Comparative hemagglutination inhibition antibody responses to MF59-adjuvanted and nonadjuvanted inactivated influenza vaccine in older adults, by subtype.
Immune responses vary according to strain and season.
|
![]() | Figure 3Seroprotection (SP) and seroconversion (SC) in adults ≥ 75 year old, by serotype. Inactivated influenza vaccine (IIV3), adjuvanted IIV (IIVa3). |
![]() | Figure 4Postvaccination GMT ratio following subsequent yearly vaccination of a cohort of elderly subjects (≥ 65 years of age) (2). |
![]() | Figure 5Hemagglutination inhibition (HI) antibody responses to vaccine (A/Wyoming/3/03(H3N2) and H3N2 strains appearing in subsequent years in elderly adjuvanted and non-adjuvanted vaccine recipients-proportion with HI titers > 40.
Committee for Medicinal Products for Human Use (CHMP)
IIV3, trivalent inactivated influenza vaccine; IIVa3, MF59 adjuvanted trivalent inactivated influenza vaccine.
|
![]() | Figure 6Age-specific hospitalizations for influenza-related illness, US, by week, 2012-13 season (http://www.cdc.gov/flu/weekly/). |
![]() | Figure 7Influenza-related hospitalizatons, US, 2012-13 season by age group and underlying medical condition (http://www.cdc.gov/flu/weekly/). |
![]() | Figure 8Lombardy influenza effectiveness (LIVE) study.
Although influenza hospitalizations were not laboratory confirmed, reductions in hospitalizations among adjuvanted vaccine recipients increased and became significant as the analysis focused on peak weeks of influenza transmission, suggesting a specific effect on hospitalizations due to influenza.
IIV3, trivalent inactivated influenza vaccine; IIVa3, MF59 adjuvanted trivalent inactivated influenza vaccine.
|
![]() | Figure 9Solicited adverse events in older adults receiving MF59-adjuvanted or nonadjuvanted seasonal influenza vaccine over three consecutive years.
IIV3, trivalent inactivated influenza vaccine; IIVa3, MF59 adjuvanted trivalent inactivated influenza vaccine.
|
![]() | Figure 10Solicited, unsolicited and serious adverse events in subjects receiving MF59-adjuvanted or non-adjuvanted seasonal influenza vaccine by adverse event category with risk ratios. |
Table 1
Average annual influenza-related hospitalization rate (95% CI) per 100,000 person-years, U.S., by viral subtype, 1993-2008 [25]
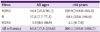
References
1. Lozano R, Naghavi M, Foreman K, Lim S, Shibuya K, Aboyans V, Abraham J, Adair T, Aggarwal R, Ahn SY, Alvarado M, Anderson HR, Anderson LM, Andrews KG, Atkinson C, Baddour LM, Barker-Collo S, Bartels DH, Bell ML, Benjamin EJ, Bennett D, Bhalla K, Bikbov B, Bin Abdulhak A, Birbeck G, Blyth F, Bolliger I, Boufous S, Bucello C, Burch M, Burney P, Carapetis J, Chen H, Chou D, Chugh SS, Coffeng LE, Colan SD, Colquhoun S, Colson KE, Condon J, Connor MD, Cooper LT, Corriere M, Cortinovis M, de Vaccaro KC, Couser W, Cowie BC, Criqui MH, Cross M, Dabhadkar KC, Dahodwala N, De Leo D, Degenhardt L, Delossantos A, Denenberg J, Des Jarlais DC, Dharmaratne SD, Dorsey ER, Driscoll T, Duber H, Ebel B, Erwin PJ, Espindola P, Ezzati M, Feigin V, Flaxman AD, Forouzanfar MH, Fowkes FG, Franklin R, Fransen M, Freeman MK, Gabriel SE, Gakidou E, Gaspari F, Gillum RF, Gonzalez-Medina D, Halasa YA, Haring D, Harrison JE, Havmoeller R, Hay RJ, Hoen B, Hotez PJ, Hoy D, Jacobsen KH, James SL, Jasrasaria R, Jayaraman S, Johns N, Karthikeyan G, Kassebaum N, Keren A, Khoo JP, Knowlton LM, Kobusingye O, Koranteng A, Krishnamurthi R, Lipnick M, Lipshultz SE, Ohno SL, Mabweijano J, MacIntyre MF, Mallinger L, March L, Marks GB, Marks R, Matsumori A, Matzopoulos R, Mayosi BM, McAnulty JH, McDermott MM, McGrath J, Mensah GA, Merriman TR, Michaud C, Miller M, Miller TR, Mock C, Mocumbi AO, Mokdad AA, Moran A, Mulholland K, Nair MN, Naldi L, Narayan KM, Nasseri K, Norman P, O'Donnell M, Omer SB, Ortblad K, Osborne R, Ozgediz D, Pahari B, Pandian JD, Rivero AP, Padilla RP, Perez-Ruiz F, Perico N, Phillips D, Pierce K, Pope CA 3rd, Porrini E, Pourmalek F, Raju M, Ranganathan D, Rehm JT, Rein DB, Remuzzi G, Rivara FP, Roberts T, De León FR, Rosenfeld LC, Rushton L, Sacco RL, Salomon JA, Sampson U, Sanman E, Schwebel DC, Segui-Gomez M, Shepard DS, Singh D, Singleton J, Sliwa K, Smith E, Steer A, Taylor JA, Thomas B, Tleyjeh IM, Towbin JA, Truelsen T, Undurraga EA, Venketasubramanian N, Vijayakumar L, Vos T, Wagner GR, Wang M, Wang W, Watt K, Weinstock MA, Weintraub R, Wilkinson JD, Woolf AD, Wulf S, Yeh PH, Yip P, Zabetian A, Zheng ZJ, Lopez AD, Murray CJ, AlMazroa MA, Memish ZA. Global and regional mortality from 235 causes of death for 20 age groups in 1990 and 2010: a systematic analysis for the Global Burden of Disease Study 2010. Lancet. 2012. 380:2095–2128.


2. Monto AS, Ansaldi F, Aspinall R, McElhaney JE, Montaño LF, Nichol KL, Puig-Barberà J, Schmitt J, Stephenson I. Influenza control in the 21st century: Optimizing protection of older adults. Vaccine. 2009. 27:5043–5053.


3. Statistics Bureau. Statistical Handbook of Japan 2012. Accessed 1 November 2012. Available at: http://www.stat.go.jp/english/data/handbook/.
4. Cai L, Uchiyama H, Yanagisawa S, Kamae I. Cost-effectiveness analysis of influenza and pneumococcal vaccinations among elderly people in Japan. Kobe J Med Sci. 2006. 52:97–109.
5. Osterholm MT, Kelley NS, Sommer A, Belongia EA. Efficacy and effectiveness of influenza vaccines: a systematic review and meta-analysis. Lancet Infect Dis. 2012. 12:36–44.


6. Simonsen L, Taylor RJ, Viboud C, Miller MA, Jackson LA. Mortality benefits of influenza vaccination in elderly people: an ongoing controversy. Lancet Infect Dis. 2007. 7:658–666.


7. Thijs C, Beyer WE, Govaert PM, Sprenger MJ, Dinant GJ, Knottnerus A. Mortality benefits of influenza vaccination in elderly people. Lancet Infect Dis. 2008. 8:460–461. author reply 463-5.


8. Goodwin K, Viboud C, Simonsen L. Antibody response to influenza vaccination in the elderly: a quantitative review. Vaccine. 2006. 24:1159–1169.


9. Song JY, Cheong HJ, Hwang IS, Choi WS, Jo YM, Park DW, Cho GJ, Hwang TG, Kim WJ. Long-term immunogenicity of influenza vaccine among the elderly: Risk factors for poor immune response and persistence. Vaccine. 2010. 28:3929–3935.


10. Reber AJ, Chirkova T, Kim JH, Cao W, Biber R, Shay DK, Sambhara S. Immunosenescence and challenges of vaccination against influenza in the aging population. Aging Dis. 2012. 3:68–90.
11. Orsi A, Ansaldi F, de Florentiis D, Ceravolo A, Parodi V, Canepa P, Coppelli M, Icardi G, Durando P. Cross-protection against drifted influenza viruses: Options offered by adjuvanted and intradermal vaccines. Hum Vaccin Immunother. 2013. 9:[Epub ahead of print].
12. Podda A. The adjuvanted influenza vaccines with novel adjuvants: experience with the MF59-adjuvanted vaccine. Vaccine. 2001. 19:2673–2680.


13. O'Hagan DT, Ott GS, Nest GV, Rappuoli R, Giudice GD. The history of MF59(®) adjuvant: a phoenix that arose from the ashes. Expert Rev Vaccines. 2013. 12:13–30.
14. Vancouver Coastal Health. Physicians' Update - 1 October 2012. Accessed 10 October 2012. Available at: http://www.vch.ca/your_health/health_topics/communicable_diseases/for_health_professionals/physician_updates/physicians__update/.
15. Ansaldi F, Bacilieri S, Durando P, Sticchi L, Valle L, Montomoli E, Icardi G, Gasparini R, Crovari P. Cross-protection by MF59-adjuvanted influenza vaccine: neutralizing and haemagglutination-inhibiting antibody activity against A(H3N2) drifted influenza viruses. Vaccine. 2008. 26:1525–1529.


16. Squarcione S, Sgricia S, Biasio LR, Perinetti E. Comparison of the reactogenicity and immunogenicity of a split and a subunit-adjuvanted influenza vaccine in elderly subjects. Vaccine. 2003. 21:1268–1274.


17. Ohmit SE, Petrie JG, Malosh RE, Cowling BJ, Thompson MG, Shay DK, Monto AS. Influenza vaccine effectiveness in the community and the household. Clin Infect Dis. 2013. 56:1363–1369.


18. Song JY, Cheong HJ, Seo YB, Kim IS, Noh JY, Choi WS, Lee J, Jeong HW, Kee SY, Kim WJ. Long-term immunogenicity of the pandemic influenza A/H1N1 2009 vaccine among health care workers: influence of prior seasonal influenza vaccination. Clin Vaccine Immunol. 2013. 20:513–516.


19. Medina RA, García-Sastre A. Influenza A viruses: new research developments. Nat Rev Microbiol. 2011. 9:590–603.


20. Russell CA, Jones TC, Barr IG, Cox NJ, Garten RJ, Gregory V, Gust ID, Hampson AW, Hay AJ, Hurt AC, de Jong JC, Kelso A, Klimov AI, Kageyama T, Komadina N, Lapedes AS, Lin YP, Mosterin A, Obuchi M, Odagiri T, Osterhaus AD, Rimmelzwaan GF, Shaw MW, Skepner E, Stohr K, Tashiro M, Fouchier RA, Smith DJ. The global circulation of seasonal influenza A (H3N2) viruses. Science. 2008. 320:340–346.


21. Khurana S, Verma N, Yewdell JW, Hilbert AK, Castellino F, Lattanzi M, Del Giudice G, Rappuoli R, Golding H. MF59 adjuvant enhances diversity and affinity of antibody-mediated immune response to pandemic influenza vaccines. Sci Transl Med. 2011. 3:85ra48.


22. Kang S, Yang IS, Lee JY, Park Y, Oh HB, Kang C, Kim KH. Epidemiologic study of human influenza virus infection in South Korea from 1999 to 2007: origin and evolution of A/Fujian/411/2002-like strains. J Clin Microbiol. 2010. 48:2177–2185.


23. Cheng X, Tan Y, He M, Lam TT, Lu X, Viboud C, He J, Zhang S, Lu J, Wu C, Fang S, Wang X, Xie X, Ma H, Nelson MI, Kung HF, Holmes EC, Cheng J. Epidemiological dynamics and phylogeography of influenza virus in southern China. J Infect Dis. 2013. 207:106–114.


24. Centers for Disease Control and Prevention. FluView: 2012-2013 Influenza season week 13 ending March 30, 2013. Accessed 2 April 2013. Available at: http://www.cdc.gov/flu/weekly/.
25. Zhou H, Thompson WW, Viboud CG, Ringholz CM, Cheng PY, Steiner C, Abedi GR, Anderson LJ, Brammer L, Shay DK. Hospitalizations associated with influenza and respiratory syncytial virus in the United States, 1993-2008. Clin Infect Dis. 2012. 54:1427–1436.


26. Madjid M, Awan I, Ali M, Frazier L, Casscells W. Influenza and atherosclerosis: vaccination for cardiovascular disease prevention. Expert Opin Biol Ther. 2005. 5:91–96.


27. Barker WH, Borisute H, Cox C. A study of the impact of influenza on the functional status of frail older people. Arch Intern Med. 1998. 158:645–650.


28. Boyd CM, Landefeld CS, Counsell SR, Palmer RM, Fortinsky RH, Kresevic D, Burant C, Covinsky KE. Recovery of activities of daily living in older adults after hospitalization for acute medical illness. J Am Geriatr Soc. 2008. 56:2171–2179.


29. Covinsky KE, Palmer RM, Fortinsky RH, Counsell SR, Stewart AL, Kresevic D, Burant CJ, Landefeld CS. Loss of independence in activities of daily living in older adults hospitalized with medical illnesses: increased vulnerability with age. J Am Geriatr Soc. 2003. 51:451–458.


30. Seo YB, Hong KW, Kim IS, Choi WS, Baek JH, Lee J, Song JY, Lee JS, Cheong HJ, Kim WJ. Effectiveness of the influenza vaccine at preventing hospitalization due to acute lower respiratory infection and exacerbation of chronic cardiopulmonary disease in Korea during 2010-2011. Vaccine. 2013. 31:1426–1430.


31. Talbot HK, Zhu Y, Chen Q, Williams JV, Thompson MG, Griffin MR. Effectiveness of influenza vaccine for preventing laboratory-confirmed influenza hospitalizations in adults, 2011-2012 influenza season. Clin Infect Dis. 2013. 56:1774–1777.


32. Puig-Barberà J, Diez-Domingo J, Pérez Hoyos S, Belenguer Varea A, González Vidal D. Effectiveness of the MF59-adjuvanted influenza vaccine in preventing emergency admissions for pneumonia in the elderly over 64 years of age. Vaccine. 2004. 23:283–289.


33. Puig-Barberà J, Díez-Domingo J, Varea AB, Chavarri GS, Rodrigo JA, Hoyos SP, Vidal DG. Effectiveness of MF59-adjuvanted subunit influenza vaccine in preventing hospitalisations for cardiovascular disease, cerebrovascular disease and pneumonia in the elder ly. Vaccine. 2007. 25:7313–7321.


34. Gasparini R, Amicizia D, Lai PL, Rossi S, Panatto D. Effectiveness of adjuvanted seasonal influenza vaccines (Inflexal V® and Fluad®) in preventing hospitalization for influenza and pneumonia in the elderly: a matched case-control study. Hum Vaccin Immunother. 2013. 9:144–152.


35. Puig-Barberà J, Díez-Domingo J, Arnedo-Pena A, Ruiz-García M, Pérez-Vilar S, Micó-Esparza JL, Belenguer-Varea A, Carratalá-Munuera C, Gil-Guillén V, Schwarz-Chavarri H. Effectiveness of the 2010-2011 seasonal influenza vaccine in preventing confirmed influenza hospitalizations in adults: a case-case comparison, case-control study. Vaccine. 2012. 30:5714–5720.


36. Eick-Cost AA, Tastad KJ, Guerrero AC, Johns MC, Lee SE, Macintosh VH, Burke RL, Blazes DL, Russell KL, Sanchez JL. Effectiveness of seasonal influenza vaccines against influenza-associated illnesses among US military personnel in 2010-11: a case-cont rol approach. PLoS One. 2012. 7:e41435.
37. Kissling E, Valenciano M, Cohen JM, Oroszi B, Barret AS, Rizzo C, Stefanoff P, Nunes B, Pitigoi D, Larrauri A, Daviaud I, Horvath JK, O'Donnell J, Seyler T, Paradowska-Stankiewicz IA, Pechirra P, Ivanciuc AE, Jiménez-Jorge S, Savulescu C, Ciancio BC, Moren A. I-MOVE multi-centre case control study 2010-11: overall and stratified estimates of influenza vaccine effectiveness in Europe. PLoS One. 2011. 6:e27622.


38. Pebody RG, Andrews N, Fleming DM, McMenamin J, Cottrell S, Smyth B, Durnall H, Robertson C, Carman W, Ellis J, Sebastian-Pillai P, Zambon M, Kearns C, Moore C, Thomas DR, Watson JM. Age-specific vaccine effectiveness of seasonal 2010/2011 and pandemic influenza A(H1N1) 2009 vaccines in preventing influenza in the United Kingdom. Epidemiol Infect. 2012. 1–11.


39. Skowronski DM, Janjua NZ, De Serres G, Winter AL, Dickinson JA, Gardy JL, Gubbay J, Fonseca K, Charest H, Crowcroft NS, Fradet MD, Bastien N, Li Y, Krajden M, Sabaiduc S, Petric M. A sentinel platform to evaluate influenza vaccine effectiveness and new variant circulation, Canada 2010-2011 season. Clin Infect Dis. 2012. 55:332–342.


40. Treanor JJ, Talbot HK, Ohmit SE, Coleman LA, Thompson MG, Cheng PY, Petrie JG, Lofthus G, Meece JK, Williams JV, Berman L, Breese Hall C, Monto AS, Griffin MR, Belongia E, Shay DK. US Flu-VE Network. Effectiveness of seasonal influenza vaccines in the United States during a season with circulation of all three vaccine strains. Clin Infect Dis. 2012. 55:951–959.


41. Iob A, Brianti G, Zamparo E, Gallo T. Evidence of increased clinical protection of an MF59-adjuvant influenza vaccine compared to a non-adjuvant vaccine among elderly residents of long-term care facilities in Italy. Epidemiol Infect. 2005. 133:687–693.


42. Mannino S, Villa M, Apolone G, Weiss NS, Groth N, Aquino I, Boldori L, Caramaschi F, Gattinoni A, Malchiodi G, Rothman KJ. Effectiveness of adjuvanted influenza vaccination in elderly subjects in northern Italy. Am J Epidemiol. 2012. 176:527–533.


43. Levandowski RA, Regnery HL, Staton E, Burgess BG, Williams MS, Groothuis JR. Antibody responses to influenza B viruses in immunologically unprimed children. Pediatrics. 1991. 88:1031–1036.


44. Reed C, Meltzer MI, Finelli L, Fiore A. Public health impact of including two lineages of influenza B in a quadrivalent seasonal influenza vaccine. Vaccine. 2012. 30:1993–1998.


45. Dreyfus C, Laursen NS, Kwaks T, Zuijdgeest D, Khayat R, Ekiert DC, Lee JH, Metlagel Z, Bujny MV, Jongeneelen M, van der Vlugt R, Lamrani M, Korse HJ, Geelen E, Sahin Ö, Sieuwerts M, Brakenhoff JP, Vogels R, Li OT, Poon LL, Peiris M, Koudstaal W, Ward AB, Wilson IA, Goudsmit J, Friesen RH. Highly conserved protective epitopes on influenza B viruses. Science. 2012. 337:1343–1348.


46. Janjua NZ, Skowronski DM, De Serres G, Dickinson J, Crowcroft NS, Taylor M, Winter AL, Hottes TS, Fonseca K, Charest H, Drews SJ, Sabaiduc S, Bastien N, Li Y, Gardy JL, Petric M. Estimates of influenza vaccine effectiveness for 2007-2008 from Canada's sentinel surveillance system: cross-protection against major and minor variants. J Infect Dis. 2012. 205:1858–1868.


47. Konrad S. Influenza Comparative Vaccine Effectiveness Study. In : Annual meeting of 10th Canadian Immunization Conference; 2012 Dec 4; Vancouver, Canada.
48. Castilla J, Martínez-Baz I, Martínez-Artola V, Reina G, Pozo F, García Cenoz M, Guevara M, Morán J, Irisarri F, Arriazu M, Albéniz E, Ezpeleta C, Barricarte A. Primary Health Care Sentinel Network. Network for Influenza Surveillance in Hospitals of Navarre. Decline in influenza vaccine effectiveness with time after vaccination, Navarre, Spain, season 2011/12. Euro Surveill. 2013. 18:pii: 20388.


49. Kissling E, Valenciano M, Larrauri A, Oroszi B, Cohen JM, Nunes B, Pitigoi D, Rizzo C, Rebolledo J, Paradowska-Stankiewicz I, Jiménez-Jorge S, Horváth JK, Daviaud I, Guiomar R, Necula G, Bella A, O'Donnell J, Głuchowska M, Ciancio BC, Nicoll A, Moren A. Low and decreasing vaccine effectiveness against influenza A(H3) in 2011/12 among vaccination target groups in Europe: results from the I-MOVE multicentre case-control study. Euro Surveill. 2013. 18:pii: 20390.


50. MacIntosh VH, Tastad KJ, Eick-Cost AA. Mid-season influenza vaccine effectiveness 2011-2012: a Department of Defense Global, Laboratory-based, Influenza Surveillance System case-control study estimate. Vaccine. 2013. 31:1651–1655.


51. Pebody R, Andrews N, McMenamin J, Durnall H, Ellis J, Thompson CI, Robertson C, Cottrell S, Smyth B, Zambon M, Moore C, Fleming DM, Watson JM. Vaccine effectiveness of 2011/12 trivalent seasonal influenza vaccine in preventing laboratory-confirmed influenza in primary care in the United Kingdom: evidence of waning intra-seasonal protection. Euro Surveill. 2013. 18:pii: 20389.


52. Schultze V, D'Agosto V, Wack A, Novicki D, Zorn J, Hennig R. Safety of MF59 adjuvant. Vaccine. 2008. 26:3209–3222.


53. Pellegrini M, Nicolay U, Lindert K, Groth N, Della Cioppa G. MF59-adjuvanted versus non-adjuvanted influenza vaccines: integrated analysis from a large safety database. Vaccine. 2009. 27:6959–6965.


54. Cheong HJ, Song JY, Heo JY, Noh JY, Choi WS, Park DW, Wie SH, Kim WJ. Immunogenicity and safety of the influenza A/H1N1 2009 inactivated split-virus vaccine in young and older adults: MF59-adjuvanted vaccine versus nonadjuvanted vaccine. Clin Vaccine Immunol. 2011. 18:1358–1364.


55. Dell'Era L, Corona F, Daleno C, Scala A, Principi N, Esposito S. Immunogenicity, safety and tolerability of MF59-adjuvanted seasonal influenza vaccine in children with juvenile idiopathic arthritis. Vaccine. 2012. 30:936–940.
56. Moro ML, Nobilio L, Voci C, Di Mario S, Candela S, Magrini N. SaFoH1N1 working group. A population based cohort study to assess the safety of pandemic influenza vaccine Focetria in Emilia-Romagna region, Italy - part two. Vaccine. 2013. 31:1438–1446.


57. Candela S, Pergolizzi S, Ragni P, Cavuto S, Nobilio L, Di Mario S, Dragosevic V, Groth N, Magrini N. SaFoH1N1 working group. An early (3-6 weeks) active surveillance study to assess the safety of pandemic influenza vaccine Focetria® in a province of Emilia-Romagna region, Italy - part one. Vaccine. 2013. 31:1431–1437.


58. Isai A, Durand J, Le Meur S, Hidalgo-Simon A, Kurz X. Autoimmune disorders after immunisation with Influenza A/H1N1 vaccines with and without adjuvant: EudraVigilance data and literature review. Vaccine. 2012. 30:7123–7129.


59. Banzhoff A, Haertel S, Praus M. Passive surveillance of adverse events of an MF59-adjuvanted H1N1v vaccine during the pandemic mass vaccinations. Hum Vaccin. 2011. 7:539–548.


60. Moro ML, Nobilio L, Voci C, Di Mario S, Candela S, Magrini N. SaFoH1N1 working group. A population based cohort study to assess the safety of pandemic influenza vaccine Focetria in Emilia-Romagna region, Italy - part two. Vaccine. 2013. 31:1438–1446.


61. Biscayart C. Argentina's integral prevention of influenza: a successful experience of an emerging country. In : Annual meeting of Influenza vaccines for the world; 2012 Oct 10; Valencia, Spain.
62. Nohynek H, Jokinen J, Partinen M, Vaarala O, Kirjavainen T, Sundman J, Himanen SL, Hublin C, Julkunen I, Olsén P, Saarenpää-Heikkilä O, Kilpi T. AS03 adjuvanted AH1N1 vaccine associated with an abrupt increase in the incidence of childhood narcolepsy in Finland. PLoS One. 2012. 7:e33536.


63. Miller E, Andrews N, Stellitano L, Stowe J, Winstone AM, Shneerson J, Verity C. Risk of narcolepsy in children and young people receiving AS03 adjuvanted pandemic A/H1N1 2009 influenza vaccine: retrospective analysis. BMJ. 2013. 346:f794.


64. Tsai TF, Crucitti A, Nacci P, Nicolay U, Della Cioppa G, Ferguson J, Clemens R. Explorations of clinical trials and pharmacovigilance databases of MF59®-adjuvanted influenza vaccines for associated cases of narcolepsy. Scand J Infect Dis. 2011. 43:702–706.


65. Tsai TF, Del Giudice G, Crucitti A, Weil J, Narasimhan V. Is the adjuvant solely to blame? BMJ. 2013. 346:f2375.


66. Choe YJ, Bae GR, Lee DH. No association between influenza A(H1N1)pdm09 vaccination and narcolepsy in South Korea: an ecological study. Vaccine. 2012. 30:7439–7442.


67. Han F, Lin L, Li J, Dong XS, Mignot E. Decreased incidence of childhood narcolepsy 2 years after the 2009 H1N1 winter flu pandemic. Ann Neurol. 2012. [Epub ahead of print].


68. Han F, Lin L, Warby SC, Faraco J, Li J, Dong SX, An P, Zhao L, Wang LH, Li QY, Yan H, Gao ZC, Yuan Y, Strohl KP, Mignot E. Narcolepsy onset is seasonal and increased following the 2009 H1N1 pandemic in China. Ann Neurol. 2011. 70:410–417.


69. O'Hagan DT, Ott GS, De Gregorio E, Seubert A. The mechanism of action of MF59 - an innately attractive adjuvant formulation. Vaccine. 2012. 30:4341–4348.
70. Calabro S, Tortoli M, Baudner BC, Pacitto A, Cortese M, O'Hagan DT, De Gregorio E, Seubert A, Wack A. Vaccine adjuvants alum and MF59 induce rapid recruitment of neutrophils and monocytes that participate in antigen transport to draining lymph nodes. Vaccine. 2011. 29:1812–1823.


71. Mosca F, Tritto E, Muzzi A, Monaci E, Bagnoli F, Iavarone C, O'Hagan D, Rappuoli R, De Gregorio E. Molecular and cellular signatures of human vaccine adjuvants. Proc Natl Acad Sci U S A. 2008. 105:10501–10506.


72. Seubert A, Calabro S, Santini L, Galli B, Genovese A, Valentini S, Aprea S, Colaprico A, D'Oro U, Giuliani MM, Pallaoro M, Pizza M, O'Hagan DT, Wack A, Rappuoli R, De Gregorio E. Adjuvanticity of the oil-in-water emulsion MF59 is independent of Nlrp3 inflammasome but requires the adaptor protein MyD88. Proc Natl Acad Sci U S A. 2011. 108:11169–11174.

