Abstract
Background:
The stem cell-derived secretome has received considerable attention as an alternative to stem cells for therapeutic applications. However, establishing optimal culture conditions is key to obtaining appropriate secretome contents. Here, the optimal culturing environment for achieving a high-efficiency secretome was determined via hypoxic preconditioning of human adipose-derived stem cells (ASC).
Methods:
Normoxic conditioned media (NCM) and hypoxic conditioned media (HCM) were obtained after culturing human ASCs under normoxia (20% O2) or hypoxia (1% O2), respectively. Subsequently, both normal and thioacetamide-induced hepatotoxic hepatocytes were treated with NCM or HCM. In addition, partially hepatectomized mice were infused with control saline, NCM, and HCM. The effects on liver regeneration and serum transaminases levels were then compared.
Results:
Hypoxic preconditioning significantly increased mRNA expression of proinflammatory cytokines (interleukin-6 and tumor necrosis factor-) and growth factors (hepatocyte growth factor and vascular endothelial growth factor). In both normal and thioacetamide-induced hepatotoxic hepatocyte (alpha mouse liver 12 [AML12]) cell lines, HCM treatment resulted in the highest cell viability (122% and 95%, respectively), followed by NCM (111% and 78%, respectively). In addition, intravenous administration of HCM to partially hepatectomized mice resulted in substantially enhanced liver regeneration compared with the NCM group (P<0.05).
Conclusions:
Taken together, the secretome obtained from ASC with hypoxic preconditioning showed potential to alleviate liver damage both in vitro and in vivo. Hypoxic culture of ASC is expected to play an important role in regenerative medicine by inducing secretome production that is beneficial for improving liver regeneration.
REFERENCES
1). Kiuchi T., Kasahara M., Uryuhara K., Inomata Y., Uemoto S., Asonuma K, et al. Impact of graft size mismatching on graft prognosis in liver transplantation from living donors. Transplantation. 1999. 67:321–7.
2). Marcos A., Fisher RA., Ham JM., Shiffman ML., Sanyal AJ., Luketic VA, et al. Liver regeneration and function in donor and recipient after right lobe adult to adult living donor liver transplantation. Transplantation. 2000. 69:1375–9.
3). Banas A., Teratani T., Yamamoto Y., Tokuhara M., Takeshita F., Osaki M, et al. IFATS collection: in vivo therapeutic potential of human adipose tissue mesenchymal stem cells after transplantation into mice with liver injury. Stem Cells. 2008. 26:2705–12.


4). Kuo TK., Hung SP., Chuang CH., Chen CT., Shih YR., Fang SC, et al. Stem cell therapy for liver disease: parameters governing the success of using bone marrow mesenchymal stem cells. Gastroenterology. 2008. 134:2111–21.


5). Le Blanc K., Tammik C., Rosendahl K., Zetterberg E., Ringden O. HLA expression and immunologic properties of differentiated and undifferentiated mesenchymal stem cells. Exp Hematol. 2003. 31:890–6.
6). Trento C., Dazzi F. Mesenchymal stem cells and innate tolerance: biology and clinical applications. Swiss Med Wkly. 2010. 140:w13121.


7). Sgodda M., Aurich H., Kleist S., Aurich I., Konig S., Dollinger MM, et al. Hepatocyte differentiation of mesenchymal stem cells from rat peritoneal adipose tissue in vitro and in vivo. Exp Cell Res. 2007. 313:2875–86.


8). Assmus B., Honold J., Schachinger V., Britten MB., Fischer-Rasokat U., Lehmann R, et al. Transcoronary transplantation of progenitor cells after myocardial infarction. N Engl J Med. 2006. 355:1222–32.


9). Lee TJ., Bhang SH., Yang HS., La WG., Yoon HH., Shin JY, et al. Enhancement of long-term angiogenic efficacy of adipose stem cells by delivery of FGF2. Microvasc Res. 2012. 84:1–8.


10). Rubio D., Garcia S., Paz MF., De la Cueva T., Lopez-Fernandez LA., Lloyd AC, et al. Molecular characterization of spontaneous mesenchymal stem cell transformation. PLoS One. 2008. 3:e1398.


11). Rubio D., Garcia-Castro J., Martin MC., de la Fuente R., Cigudosa JC., Lloyd AC, et al. Spontaneous human adult stem cell transformation. Cancer Res. 2005. 65:3035–9.


12). Horwitz EM., Prockop DJ., Fitzpatrick LA., Koo WW., Gordon PL., Neel M, et al. Transplantability and therapeutic effects of bone marrow-derived mesenchymal cells in children with osteogenesis imperfecta. Nat Med. 1999. 5:309–13.


13). Orlic D., Kajstura J., Chimenti S., Limana F., Jakoniuk I., Quaini F, et al. Mobilized bone marrow cells repair the infarcted heart, improving function and survival. Proc Natl Acad Sci U S A. 2001. 98:10344–9.


14). Ivanovic Z., Belloc F., Faucher JL., Cipolleschi MG., Praloran V., Dello Sbarba P. Hypoxia maintains and interleukin-3 reduces the pre-colony-forming cell potential of dividing CD34(+) murine bone marrow cells. Exp Hematol. 2002. 30:67–73.


15). Liu L., Yu Q., Lin J., Lai X., Cao W., Du K, et al. Hypoxia-inducible factor-1alpha is essential for hypoxia-induced mesenchymal stem cell mobilization into the peripheral blood. Stem Cells Dev. 2011. 20:1961–71.
16). Rosova I., Dao M., Capoccia B., Link D., Nolta JA. Hypoxic preconditioning results in increased motility and improved therapeutic potential of human mesenchymal stem cells. Stem Cells. 2008. 26:2173–82.
17). Lee SM., Lee SC., Kim SJ. Contribution of human adipose tissue-derived stem cells and the secretome to the skin allograft survival in mice. J Surg Res. 2014. 188:280–9.


18). Greene AK., Puder M. Partial hepatectomy in the mouse: technique and perioperative management. J Invest Surg. 2003. 16:99–102.


19). Lee SK., Lee SC., Kim SJ. A novel cell-free strategy for promoting mouse liver regeneration: utilization of a conditioned medium from adipose-derived stem cells. Hepatol Int. 2015. 9:310–20.


20). Zhang W., Liu HT. MAPK signal pathways in the regulation of cell proliferation in mammalian cells. Cell Res. 2002. 12:9–18.


21). Liu L., Gao J., Yuan Y., Chang Q., Liao Y., Lu F. Hypoxia preconditioned human adipose derived mesenchymal stem cells enhance angiogenic potential via secretion of increased VEGF and bFGF. Cell Biol Int. 2013. 37:551–60.


22). Thangarajah H., Vial IN., Chang E., El-Ftesi S., Januszyk M., Chang EI, et al. IFATS collection: Adipose stromal cells adopt a proangiogenic phenotype under the influence of hypoxia. Stem Cells. 2009. 27:266–74.


23). Yang DC., Yang MH., Tsai CC., Huang TF., Chen YH., Hung SC. Hypoxia inhibits osteogenesis in human mesenchymal stem cells through direct regulation of RUNX2 by TWIST. PLoS One. 2011. 6:e23965.


24). Han KH., Kim AK., Kim MH., Kim DH., Go HN., Kim DI. Enhancement of angiogenic effects by hypoxia-preconditioned human umbilical cord-derived mesenchymal stem cells in a mouse model of hindlimb ischemia. Cell Biol Int. 2016. 40:27–35.


25). Jin G., Qiu G., Wu D., Hu Y., Qiao P., Fan C, et al. Allogeneic bone marrow-derived mesenchymal stem cells attenuate hepatic ischemia-reperfusion injury by suppressing oxidative stress and inhibiting apoptosis in rats. Int J Mol Med. 2013. 31:1395–401.


26). Kanazawa H., Fujimoto Y., Teratani T., Iwasaki J., Kasahara N., Negishi K, et al. Bone marrow-derived mesenchymal stem cells ameliorate hepatic ischemia reperfusion injury in a rat model. PLoS One. 2011. 6:e19195.


27). Vassilopoulos G., Wang PR., Russell DW. Transplanted bone marrow regenerates liver by cell fusion. Nature. 2003. 422:901–4.


28). Ishikawa T., Banas A., Hagiwara K., Iwaguro H., Ochiya T. Stem cells for hepatic regeneration: the role of adipose tissue derived mesenchymal stem cells. Curr Stem Cell Res Ther. 2010. 5:182–9.


29). Gnecchi M., He H., Noiseux N., Liang OD., Zhang L., Morello F, et al. Evidence supporting paracrine hypothesis for Akt-modified mesenchymal stem cell-mediated cardiac protection and functional improvement. FASEB J. 2006. 20:661–9.


30). Haynesworth SE., Baber MA., Caplan AI. Cytokine expression by human marrow-derived mesenchymal progenitor cells in vitro: effects of dexamethasone and IL-1 alpha. J Cell Physiol. 1996. 166:585–92.
31). Bai L., Lennon DP., Caplan AI., DeChant A., Hecker J., Kranso J, et al. Hepatocyte growth factor mediates mesenchymal stem cell-induced recovery in multiple sclerosis models. Nat Neurosci. 2012. 15:862–70.


32). Ranganath SH., Levy O., Inamdar MS., Karp JM. Harnessing the mesenchymal stem cell secretome for the treatment of cardiovascular disease. Cell Stem Cell. 2012. 10:244–58.


Fig. 1.
Effect of hypoxia on the expression of cytokines and growth factor in adipose-derived stem cells (ASCs). (A) Real-time quantitative polymerase chain reaction analysis of hypoxia preconditioning ASCs. (B) Western blotting of ASCs under normoxia and hypoxia. (C) Enzyme-linked immunosorbent assay analysis for measure secreted interleukin-6 (IL-6) levels in normoxic and hypoxic condition of ASC. Values represent mean±SD of three independent experiments. Abbreviations: GAPDH, glyceraldehyde 3-phosphate dehydrogenase; TNF-, tumor necrosis factor ; HGF, hepatocyte growth factor; VEGF, vascular endothelial growth factor; p-Erk, phosphorylated extracellular signal-regulated kinase; Erk, extracellular signal-regulated kinase; p-STAT3, phosphorylated signal transducer and activator of transcription 3; STAT3, signal transducer and activator of transcription 3. aP<0.05.
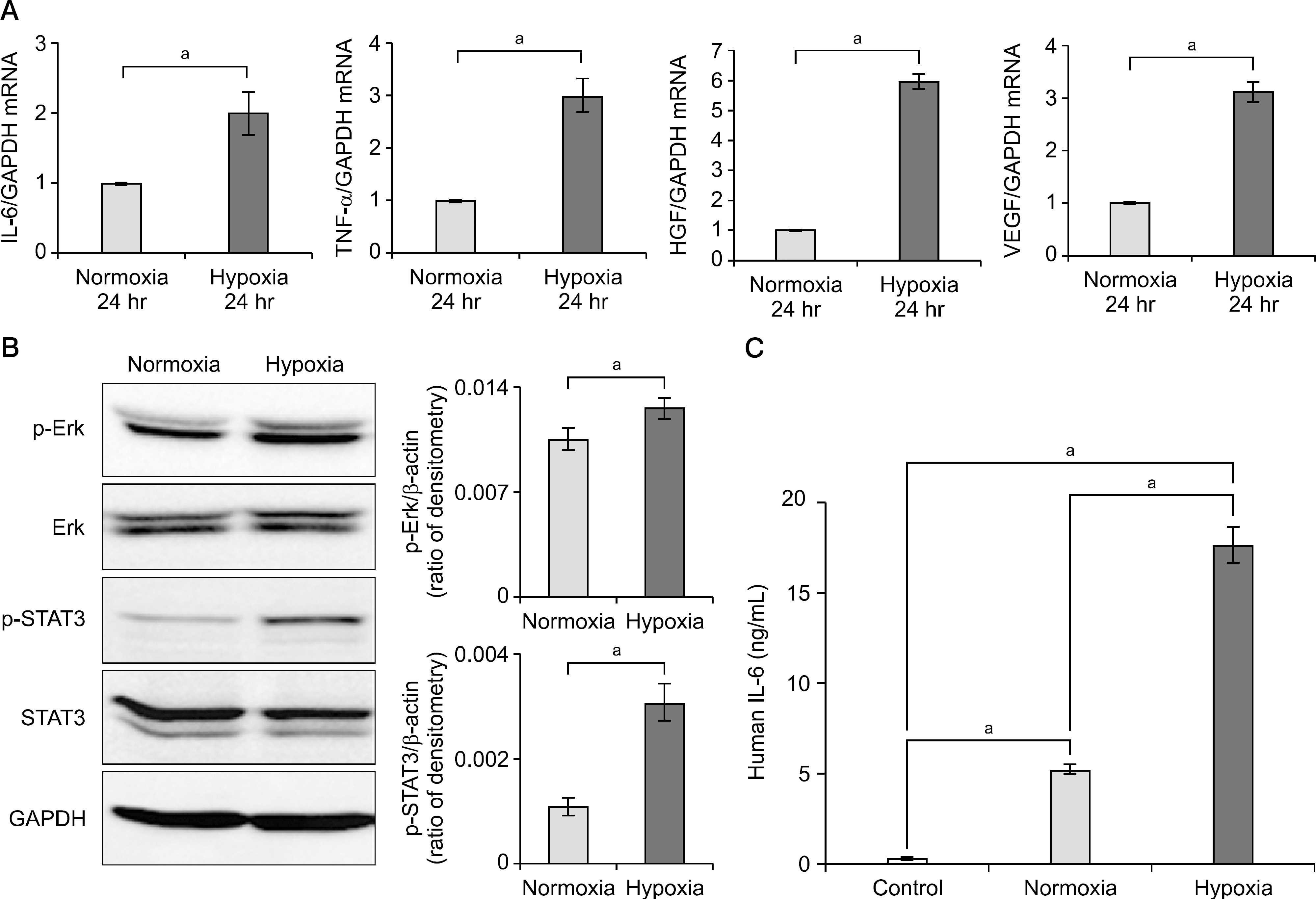
Fig. 2.
Effect of hypoxic conditioned media (HCM) on mouse hepatocyte (alpha mouse liver 12 [AML12]) cells. (A) Cell viability assay showing dose-dependent effects of the hepatotoxic molecule thioacetamide (TAA) on mouse hepatocyte AML12 proliferation. (B) Western blotting of AML12 cells under series concentration of TAA. (C) Relative densities of proliferating cell nuclear antigen (PCNA) according to TAA concentrations. (D) Effect of HCM on TAA-treated or untreated AML12 cells viabilities. (E) Effect of HCM on TAA-treated AML12 cells. (F) Relative densities of PCNA in each group. Values represent mean±SD of three independent experiments. Abbreviation: NCM, normoxic conditioned media. aP<0.05.
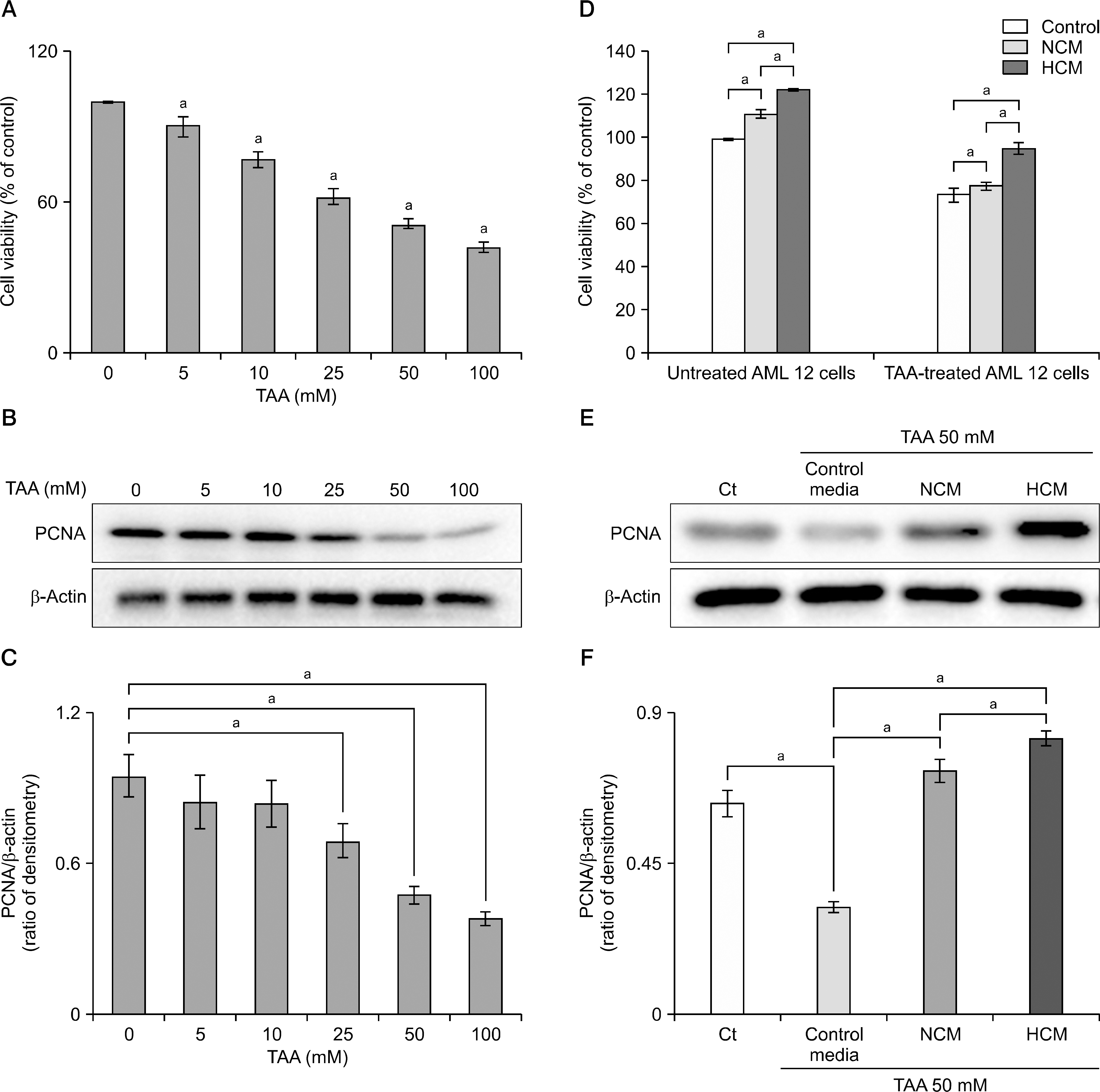
Fig. 3.
Hypoxic conditioned media (HCM) effects on inflammation in the liver of partially hepatectomized mice. (A) Enzyme-linked immunosorbent assay (ELISA) analysis for the serum levels of interleukin-6 (IL-6) in each group at 1, 2, 3 days after infusion. (B) ELISA analysis for the serum levels of tumor necrosis factor (TNF-) in each group at 1, 2, 3 days after infusion. In the experiment presented in (A) and (B), each group included 20 mice at each time point (80 mice in total). Values represent mean±SD. Abbreviation: NCM, normoxic conditioned media. aP<0.05.
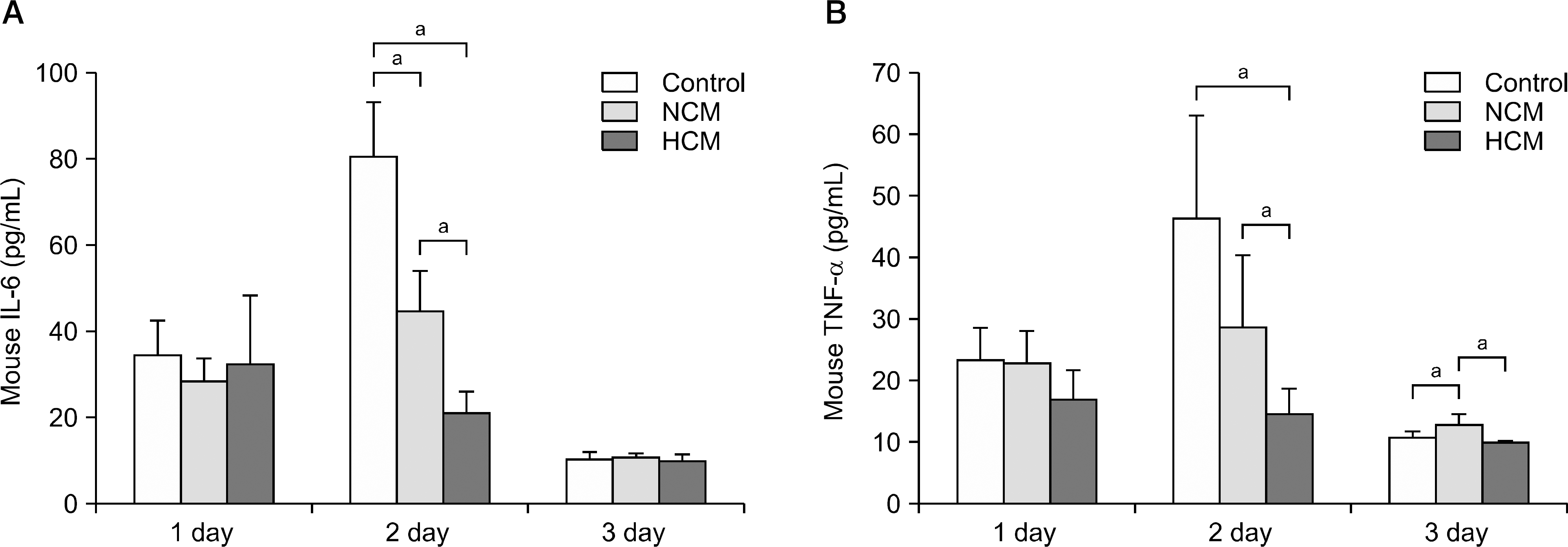
Fig. 4.
Hypoxic conditioned media (HCM) effects on liver regeneration in partially hepatectomized mice. (A, upper) Immunohistochemical stain of Ki67 (regeneration marker) in the liver specimens of each group at 1 day after infusion. (A, under) Percentage of Ki67 positive cells in each group at 1, 2, 3, and 7 days after infusion. (B, upper) Immunohistochemical stain of bromodeoxyuridine (BrdU; regeneration marker) in the liver specimens of each group at 1day after infusion. (B, under) Percentage of BrdU positive cells in each group at 1, 2, 3, and 7 days after infusion. (C, upper) Western blotting analysis for expression of proliferating cell nuclear antigen (PCNA; liver regeneration marker) and phosphorylation of signal transducer and activator of transcription 3 (STAT3) at 2 days after infusion. (C, under) Relative densities of these markers in each group. (D) Comparison of liver weight to body weight of each group at 7 days after infusion. In the experiment presented in (A) to (D), each group included 20 mice at each time point (80 mice in total). Values represent mean±SD. Abbreviations: NCM, normoxic conditioned media; p-STAT3, phosphorylated signal transducer and activator of transcription 3. aP<0.05.
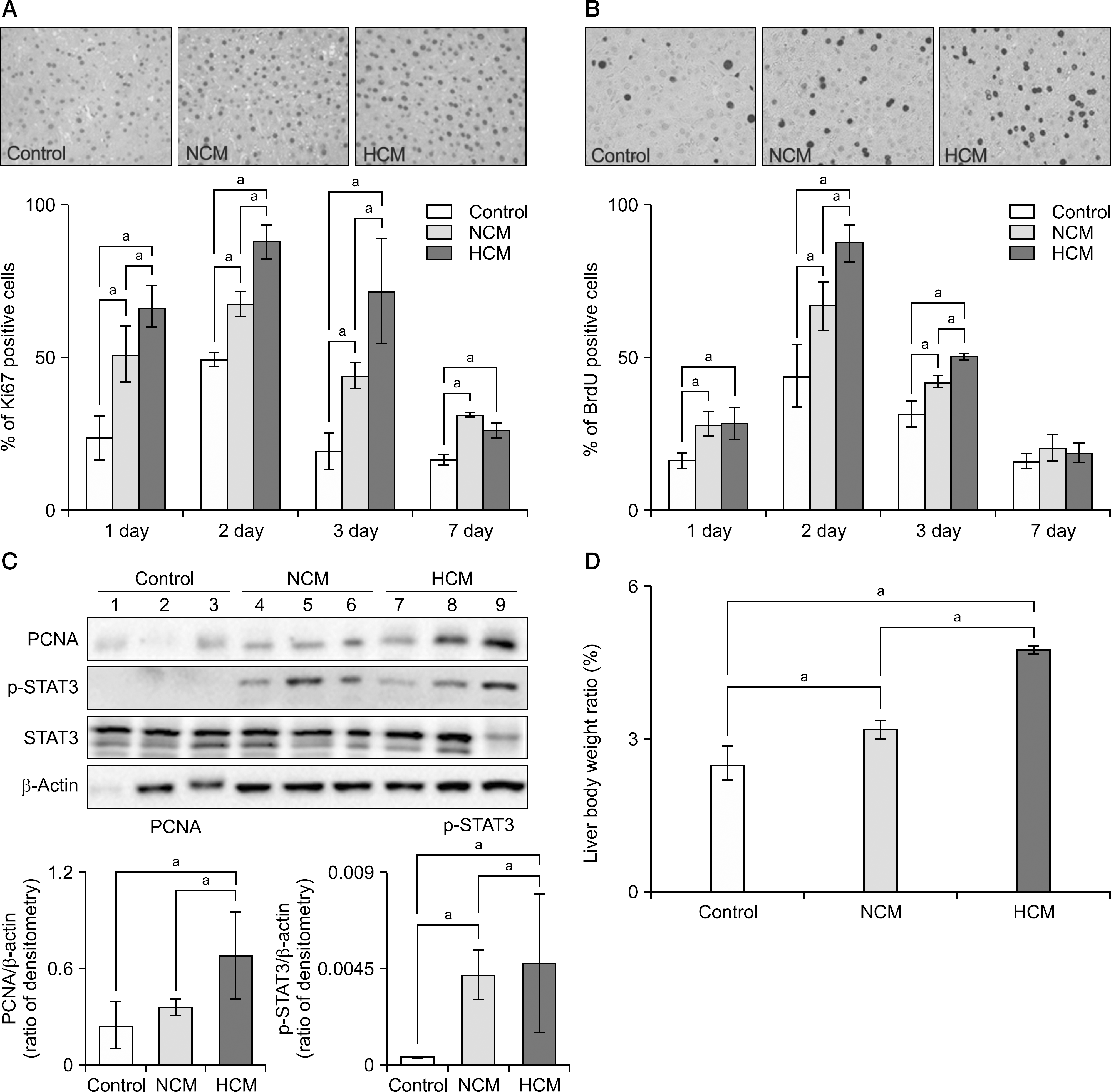
Fig. 5.
Hypoxic conditioned media (HCM) effects on hepatic function in partially hepatectomized mice. (A) Serology tests of aspartate transaminase (AST) in each group. (B) Serology tests of alanine transaminase (ALT) in each group. In the experiment presented in (A) and (B), in the serologic tests, each group included 5 mice (20 mice in total). Values represent mean±SD. Abbreviation: NCM, normoxic conditioned media. aP<0.05.
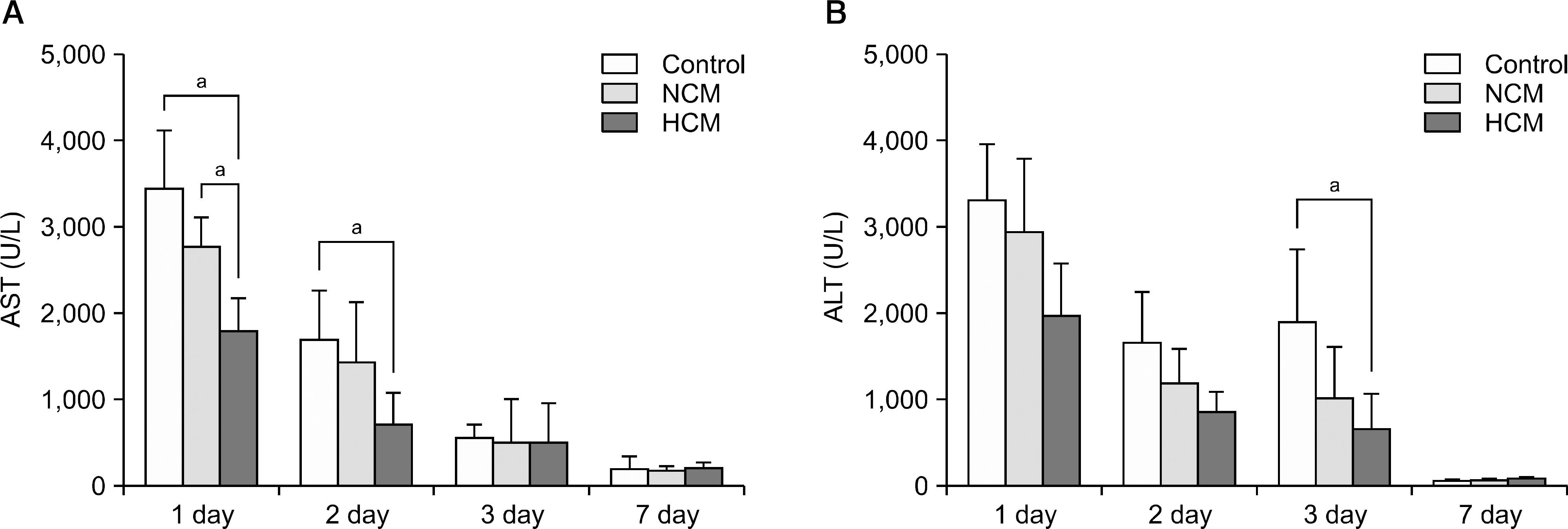