Abstract
Backgrounds
Type I diabetes mellitus (T1DM), an autoimmune disease, is associated with insulin deficiency due to the death of -cells. Bone marrowderived mesenchymal stem cells (BM-MSCs) are capable of tissue repair and thus are a promising source of -cell surrogates.
Methods
In this study, the therapeutic potential of BM-MSCs as -cell replacements was analyzed both in vitro and in vivo. First, we used retinoic acid (RA) to induce rat BM-MSCs to differentiate into cells of endodermal/pancreatic lineage. Then, differentiated rat BM-MSCs were syngeneically injected under the renal capsule of rats.
Results
Analysis of gene expression revealed that rat BM-MSCs showed signs of early pancreatic development, and differentiated cells were qualitatively and quantitatively confirmed to produce insulin in vitro. In vivo study was performed for short-term (3 weeks) and long-term (8 weeks) period of time. Rats that were injected with differentiated MSCs exhibited a reduction in blood glucose levels throughout 8 weeks, and grafted cells survived in vivo for at least 3 weeks.
References
1). Calne R. Cell transplantation for diabetes. Philos Trans R Soc Lond B Biol Sci. 2005; 360:1769–74.


2). Ackermann AM, Gannon M. Molecular regulation of pancreatic beta-cell mass development, maintenance, and expansion. J Mol Endocrinol. 2007; 38:193–206.
3). Melloul D, Marshak S, Cerasi E. Regulation of pdx-1 gene expression. Diabetes. 2002; 51(Suppl 3):S320–5.


4). Guz Y, Montminy MR, Stein R, Leonard J, Gamer LW, Wright CV, et al. Expression of murine STF-1, a putative insulin gene transcription factor, in beta cells of pancreas, duodenal epithelium and pancreatic exocrine and endocrine progenitors during ontogeny. Development. 1995; 121:11–8.


5). Huang HP, Liu M, El-Hodiri HM, Chu K, Jamrich M, Tsai MJ. Regulation of the pancreatic islet-specific gene BETA2 (neuroD) by neurogenin 3. Mol Cell Biol. 2000; 20:3292–307.
6). Fuchs E, Segre JA. Stem cells: a new lease on life. Cell. 2000; 100:143–55.
7). Anzalone R, Lo Iacono M, Loria T, Di Stefano A, Giannuzzi P, Farina F, et al. Wharton's jelly mesenchymal stem cells as candidates for beta cells regeneration: extending the differentiative and immunomodulatory benefits of adult mesenchymal stem cells for the treatment of type 1 diabetes. Stem Cell Rev. 2011; 7:342–63.


8). Bacigalupo A, Valle M, Podesta M, Pitto A, Zocchi E, De Flora A, et al. T-cell suppression mediated by mesenchymal stem cells is deficient in patients with severe aplastic anemia. Exp Hematol. 2005; 33:819–27.


9). Jung EJ, Kim SC, Wee YM, Kim YH, Choi MY, Jeong SH, et al. Bone marrowderived mesenchymal stromal cells support rat pancreatic islet survival and insulin secretory function in vitro. Cytotherapy. 2011; 13:19–29.


10). Popp FC, Renner P, Eggenhofer E, Slowik P, Geissler EK, Piso P, et al. Mesenchymal stem cells as immunomodulators after liver transplantation. Liver Transpl. 2009; 15:1192–8.


11). Rasmusson I, Ringden O, Sundberg B, Le Blanc K. Mesenchymal stem cells inhibit lymphocyte proliferation by mitogens and alloantigens by different mechanisms. Exp Cell Res. 2005; 305:33–41.


12). Efrat S. Regulation of insulin secretion: insights from engineered beta-cell lines. Ann N Y Acad Sci. 2004; 1014:88–96.
13). Oh SH, Muzzonigro TM, Bae SH, LaPlante JM, Hatch HM, Petersen BE. Adult bone marrowderived cells trans-differentiating into insulin-producing cells for the treatment of type I diabetes. Lab Invest. 2004; 84:607–17.


14). Lee KD, Kuo TK, Whang-Peng J, Chung YF, Lin CT, Chou SH, et al. In vitro hepatic differentiation of human mesenchymal stem cells. Hepatology. 2004; 40:1275–84.


15). Seeberger KL, Eshpeter A, Rajotte RV, Korbutt GS. Epithelial cells within the human pancreas do not coexpress mesenchymal antigens: epithelial-mesenchymal transition is an artifact of cell culture. Lab Invest. 2009; 89:110–21.


16). Seo MJ, Suh SY, Bae YC, Jung JS. Differentiation of human adipose stromal cells into hepatic lineage in vitro and in vivo. Biochem Biophys Res Commun. 2005; 328:258–64.


17). Zanini C, Bruno S, Mandili G, Baci D, Cerutti F, Cenacchi G, et al. Differentiation of mesenchymal stem cells derived from pancreatic islets and bone marrow into islet-like cell phenotype. PLoS One. 2011; 6:e28175.


18). Zhou H, Ding S. Evolution of induced pluripotent stem cell technology. Curr Opin Hematol. 2010; 17:276–80.


19). Hori Y, Rulifson IC, Tsai BC, Heit JJ, Cahoy JD, Kim SK. Growth inhibitors promote differentiation of insulin-pro-ducing tissue from embryonic stem cells. Proc Natl Acad Sci U S A. 2002; 99:16105–10.


20). Kang HM, Kim J, Park S, Kim J, Kim H, Kim KS, et al. Insulin-secreting cells from human eyelid-derived stem cells alleviate type I diabetes in immunocompetent mice. Stem Cells. 2009; 27:1999–2008.


21). Tang DQ, Cao LZ, Burkhardt BR, Xia CQ, Litherland SA, Atkinson MA, et al. In vivo and in vitro characterization of insulin-producing cells obtained from murine bone marrow. Diabetes. 2004; 53:1721–32.


22). Shi Y, Hou L, Tang F, Jiang W, Wang P, Ding M, et al. Inducing embryonic stem cells to differentiate into pancreatic beta cells by a novel three-step approach with activin A and all-trans retinoic acid. Stem Cells. 2005; 23:656–62.
23). Delaspre F, Massumi M, Salido M, Soria B, Ravassard P, Savatier P, et al. Directed pancreatic acinar differentiation of mouse embryonic stem cells via embryonic signalling molecules and exocrine transcription factors. PLoS One. 2013; 8:e54243.


24). Cavallari G, Olivi E, Bianchi F, Neri F, Foroni L, Valente S, et al. Mesenchymal stem cells and islet cotransplantation in diabetic rats: improved islet graft revascularization and function by human adipose tissue-derived stem cells pre-conditioned with natural molecules. Cell Transplant. 2012; 21:2771–81.


25). Kim JH, Park SN, Suh H. Generation of insulin-producing human mesenchymal stem cells using recombinant ad-eno-associated virus. Yonsei Med J. 2007; 48:109–19.


26). Kim JH, Shin KH, Li TZ, Suh H. Potential of nucleofected human MSCs for insulin secretion. J Tissue Eng Regen Med. 2011; 5:761–9.


27). Otonkoski T, Beattie GM, Mally MI, Ricordi C, Hayek A. Nicotinamide is a potent inducer of endocrine differentiation in cultured human fetal pancreatic cells. J Clin Invest. 1993; 92:1459–66.


28). Sullivan GJ, Hay DC, Park IH, Fletcher J, Hannoun Z, Payne CM, et al. Generation of functional human hepatic endoderm from human induced pluripotent stem cells. Hepatology. 2010; 51:329–35.


29). Mfopou JK, Chen B, Sui L, Sermon K, Bouwens L. Recent advances and prospects in the differentiation of pancreatic cells from human embryonic stem cells. Diabetes. 2010; 59:2094–101.


30). Ostrom M, Loffler KA, Edfalk S, Selander L, Dahl U, Ricordi C, et al. Retinoic acid promotes the generation of pancreatic endocrine progenitor cells and their further differentiation into beta-cells. PLoS One. 2008; 3:e2841.
31). Li TZ, Kim JH, Cho HH, Lee HS, Kim KS, Lee SW, et al. Therapeutic potential of bone-marrowderived mesenchymal stem cells differentiated with growth-factor-free coculture method in liver-injured rats. Tissue Eng Part A. 2010; 16:2649–59.


32). Shen CN, Marguerie A, Chien CY, Dickson C, Slack JM, Tosh D. All-trans retinoic acid suppresses exocrine differentiation and branching morphogenesis in the embryonic pancreas. Differentiation. 2007; 75:62–74.


33). Peck AB, Ramiya V. In vitro-generation of surrogate islets from adult stem cells. Transpl Immunol. 2004; 12:259–72.


34). Sumi S, Gu Y, Hiura A, Inoue K. Stem cells and regenerative medicine for diabetes mellitus. Pancreas. 2004; 29:e85–9.


Fig. 1.
Fluorescence-activated cell sorting analysis for characterization of rat mesenchymal stem cells (MSCs) at passage 3. Abbreviation: IgG, immunoglobulin G.
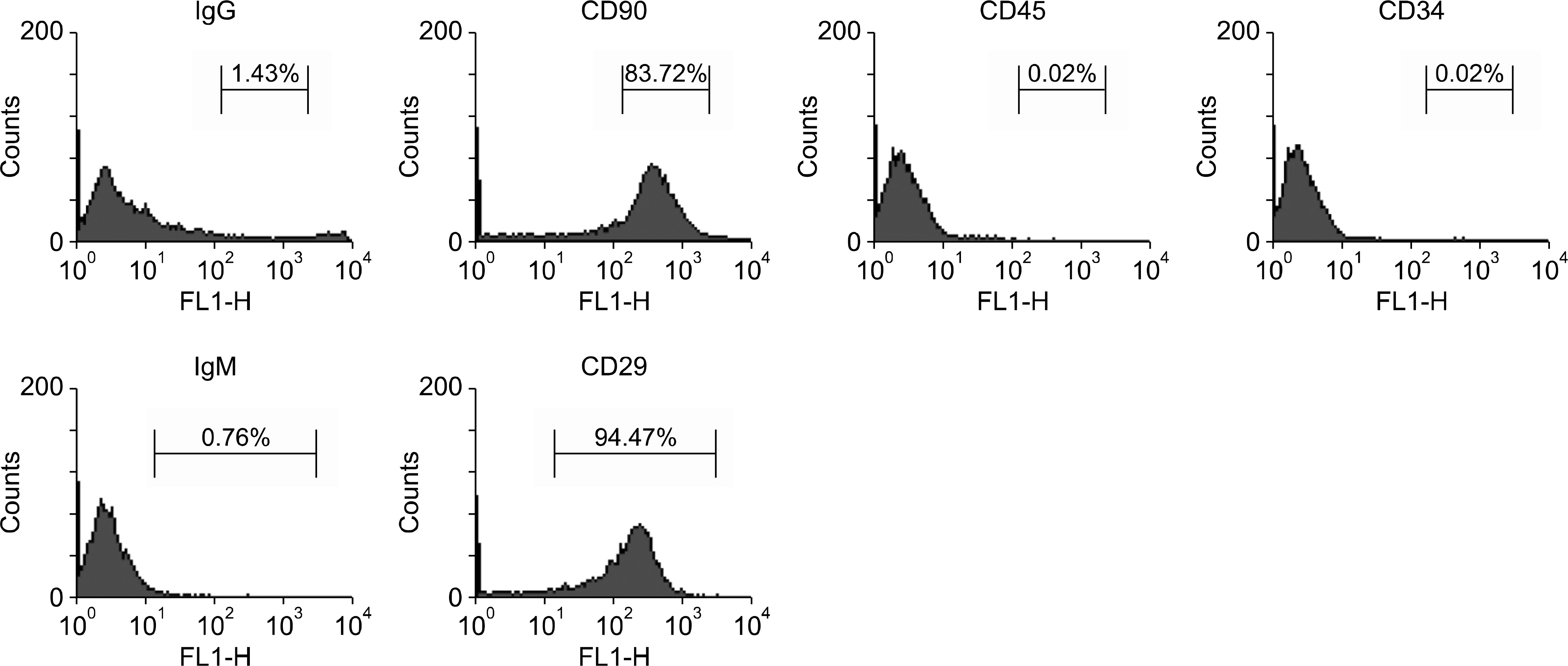
Fig. 2.
Morphological changes of differentiated rat bone marrowderived mesenchymal stem cells. (A, B) Week 1. (C, D) Week 2. (E, F) Week 3 (A, C, E: ×40; B, D, F: ×100).

Fig. 3.
Reverse-transcriptase polymerase chain reaction of rat bone marrowderived mesenchymal stem cells (rBM-MSCs). Lanes 1 to 3: differentiated rBM-MSCs from week 1 to 3. Abbreviations: FoxA2, forkhead box A2; PDX-1, pancreatic-duodenal homeobox 1; Ngn3, neurogenin 3; Pax4, paired box gene 4; GAPDH, glyceraldehyde 3-phosphate dehydrogenase.
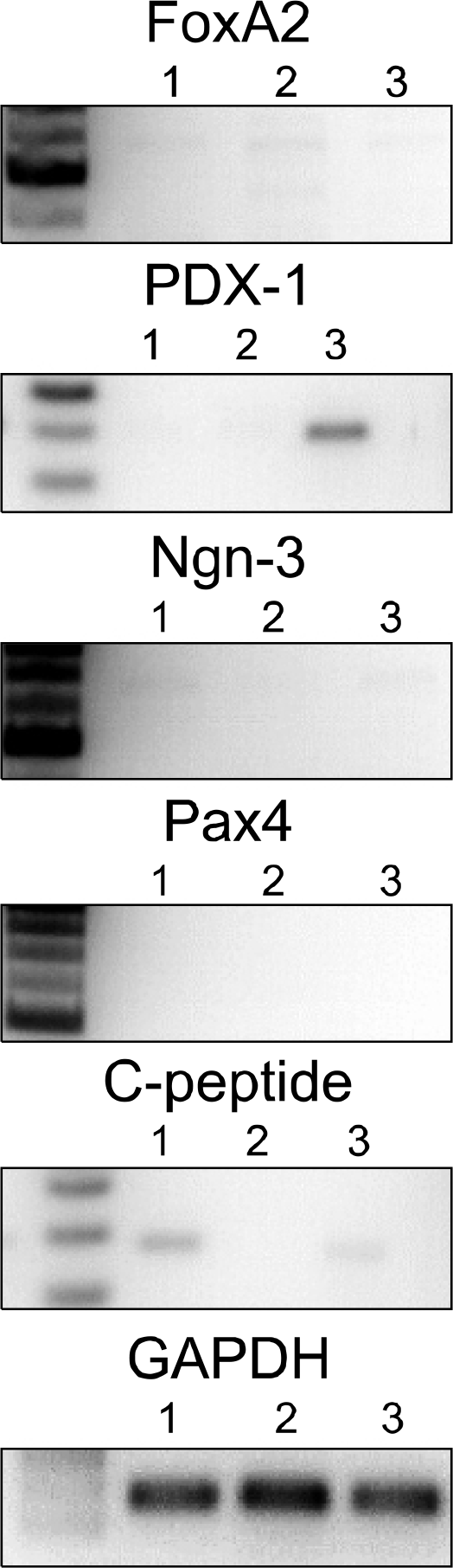
Fig. 4.
Immunocytochemistry of differentiated rat bone marrowderived mesenchymal stem cells at week 3. (A) Insulin (green). (B) 4’, 6-diamidino-2-phenyindole (DAPI, blue). (C) Light microscopic image. (D) Merged insulin and DAPI images. (E) Merged insulin, DAPI, and light microscopic images (magnification, ×100; scale bar=200 m).

Fig. 5.
Insulin secretion from differentiated rat bone marrowderived mesenchymal stem cells (MSCs) measured by enzyme-linked Immunosorbent Assay at week 3 (n=9). a P<0.05.
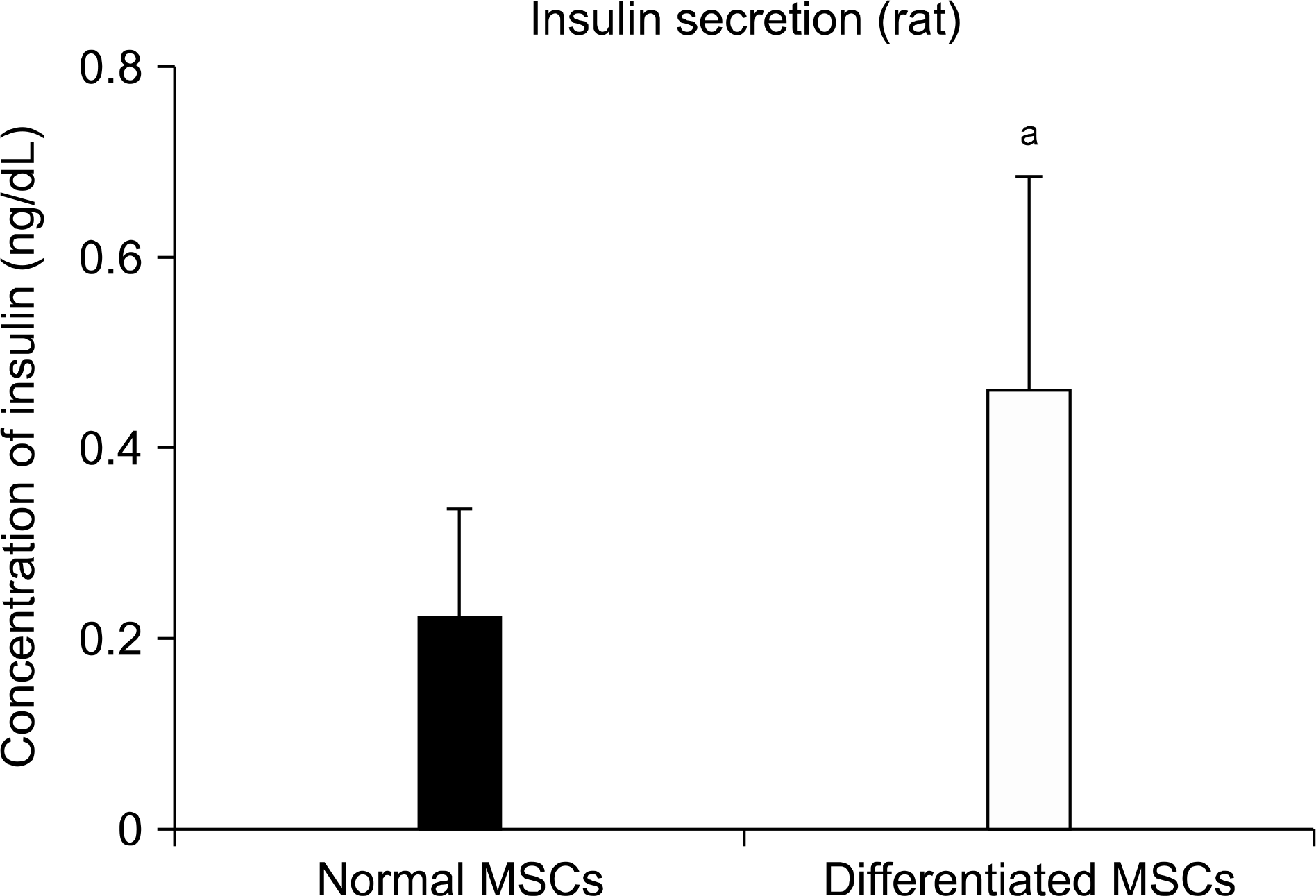
Fig. 6.
Blood glucose levels of rats observed for 3 (A, B) or 8 (C) weeks. (A) Positive control (n=4) and sham control (n=4). (B) Blood glucose level of normal mesenchymal stem cell (MSC)-injected (n=9) and differentiated MSC-injected (n=8) rats. (C) Positive control (n=4) and differentiated MSC-injected (n=8) rats. (D) Linear regression of blood glucose levels from MSC-injected (n=9) and differentiated MSC-injected (n=8) rats. Slope for MSC-injected group=−0.4935±0.5103; slope for differentiated MSC-injected group=−1.728±0.7725.
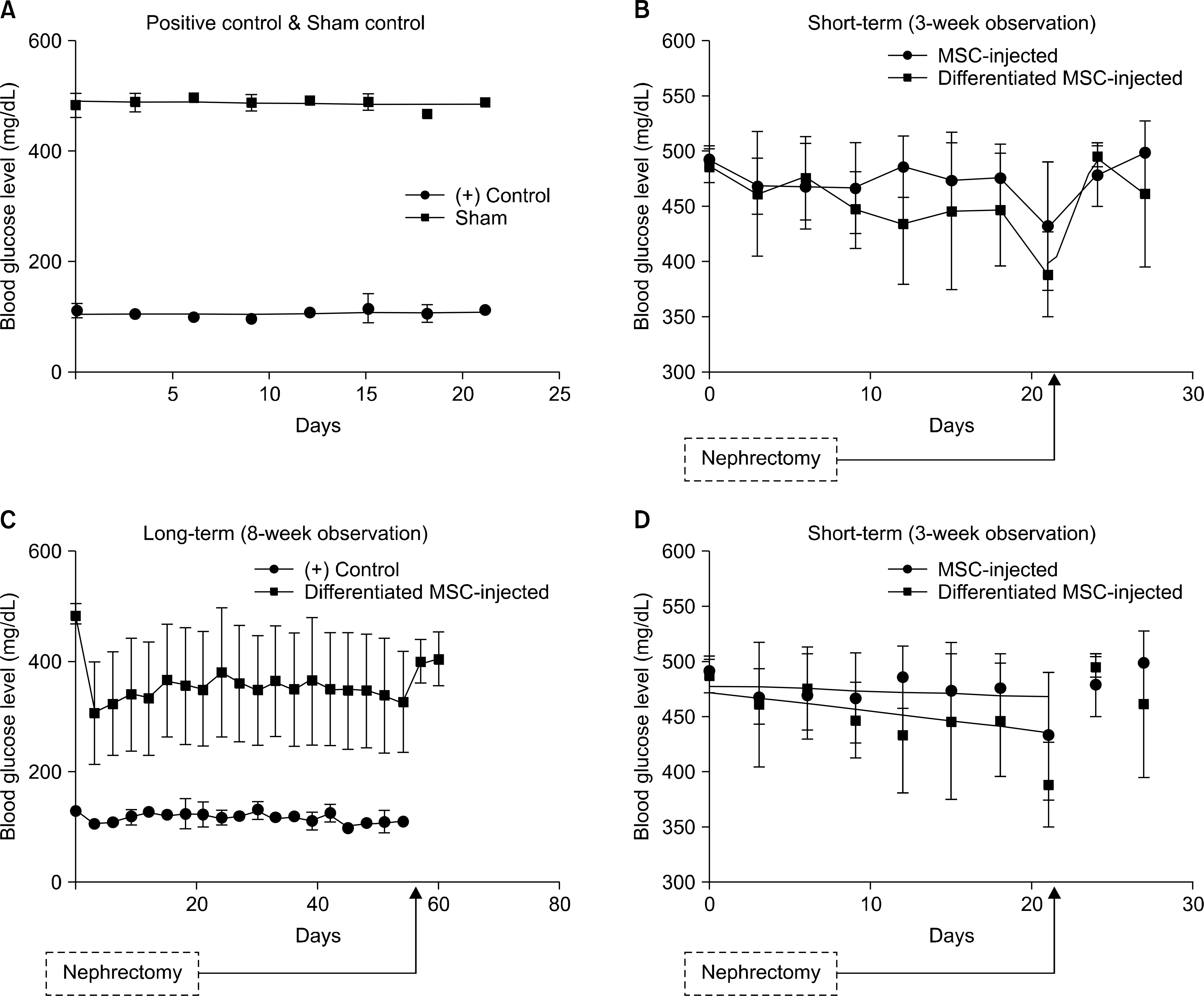
Fig. 7.
3,3’-Diaminobenzidine-stained tissue. (A) Cells located along the inner surface of the tissue membrane. (B) Cell that migrated a short distance into the tissue (marked by yellow arrowhead) (magnification: ×100; scale bar=200 m).

Fig. 8.
PKH26-labeled cells in kidney capsule (marked by yellow arrowheads). (A, B) Cell located along the inner surface of the tissue membrane. (C) Cells that migrated a short distance into the tissue (magnification: ×100; scale bar=200 m).

Table 1.
Primers for rat bone marrowderived mesenchymal stem cells