Abstract
Eosinophils are multifunctional leukocytes that reside in several tissues, most abundantly in the small intestinal lamina propria under the steady state. To date, the phenotypic and functional characteristics of small intestinal eosinophils have remained poorly understood. In this study, we found that proliferation of ovalbumin (OVA)-specific CD4+ T cells isolated from the mesenteric lymph nodes of eosinophil-deficient ΔdblGATA mice were decreased relative to wild-type mice after oral immunization with OVA and cholera toxin (CT), the typical mucosal adjuvant that induces CD4+ T cell-dependent responses. ΔdblGATA mice showed reduced mucosal secretion of OVA-specific IgA and IgG1 while maintaining a systemic level of anti-OVA IgG1 upon oral immunization with OVA and CT. These findings suggest that eosinophils might have a role in the modulation of T cell-mediated immune responses including mucosal antibody responses in the gastrointestinal tract following oral immunization.
REFERENCES
2). Jung Y, Rothenberg ME. Roles and regulation of gastrointestinal eosinophils in immunity and disease. J Immunol. 2014; 193:999–1005.


3). Rothenberg ME. Eosinophilic gastrointestinal disorders (EGID). J Allergy Clin Immunol. 2004; 113:11–28.


4). Yang D, Chen Q, Su SB, Zhang P, Kurosaka K, Caspi RR, et al. Eosinophil-derived neurotoxin acts as an alarmin to activate the TLR2-MyD88 signal pathway in dendritic cells and enhances Th2 immune responses. J Exp Med. 2008; 205:79–90.


5). Hirasawa R, Shimizu R, Takahashi S, Osawa M, Takayanagi S, Kato Y, et al. Essential and instructive roles of GATA factors in eosinophil development. J Exp Med. 2002; 195:1379–86.


6). Yu C, Cantor AB, Yang H, Browne C, Wells RA, Fujiwara Y, et al. Targeted deletion of a high-affinity GATA-binding site in the GATA-1 promoter leads to selective loss of the eosinophil lineage in vivo. J Exp Med. 2002; 195:1387–95.
7). Mishra A, Hogan SP, Lee JJ, Foster PS, Rothenberg ME. Fundamental signals that regulate eosinophil homing to the gastrointestinal tract. J Clin Invest. 1999; 103:1719–27.


8). Carlens J, Wahl B, Ballmaier M, Bulfone-Paus S, Förster R, Pabst O. Common gamma-chain-dependent signals confer selective survival of eosinophils in the murine small intestine. J Immunol. 2009; 183:5600–7.
9). Verjan Garcia N, Umemoto E, Saito Y, Yamasaki M, Hata E, Matozaki T, et al. SIRPalpha/CD172a Regulates Eosinophil Homeostasis. J Immunol. 2011; 187:2268–77.
10). Mowat AM. Anatomical basis of tolerance and immunity to intestinal antigens. Nat Rev Immunol. 2003; 3:331–41.


11). Fagarasan S, Kawamoto S, Kanagawa O, Suzuki K. Adaptive immune regulation in the gut: T cell-dependent and T cell-independent IgA synthesis. Annu Rev Immunol. 2010; 28:243–73.


12). Elson CO, Ealding W. Generalized systemic and mucosal immunity in mice after mucosal stimulation with cholera toxin. J Immunol. 1984; 132:2736–41.
13). Lycke N, Holmgren J. Strong adjuvant properties of cholera toxin on gut mucosal immune responses to orally presented antigens. Immunology. 1986; 59:301–8.
14). Bergqvist P, Gärdby E, Stensson A, Bemark M, Lycke NY. Gut IgA class switch recombination in the absence of CD40 does not occur in the lamina propria and is independent of germinal centers. J Immunol. 2006; 177:7772–83.


15). Jung Y, Wen T, Mingler MK, Caldwell JM, Wang YH, Chaplin DD, et al. IL-1beta in eosinophil-mediated small intestinal homeostasis and IgA production. Mucosal Immunol. 2015; 8:930–42.
16). Westlund J, Livingston M, Fahlén-Yrlid L, Oldenborg PA, Yrlid U. CD47-deficient mice have decreased production of intestinal IgA following oral immunization but a maintained capacity to induce oral tolerance. Immunology. 2012; 135:236–44.


17). Yamamoto M, Rennert P, McGhee JR, Kweon MN, Yamamoto S, Dohi T, et al. Alternate mucosal immune system: organized Peyer's patches are not required for IgA responses in the gastrointestinal tract. J Immunol. 2000; 164:5184–91.


18). Orr N, Robin G, Cohen D, Arnon R, Lowell GH. Immunogenicity and efficacy of oral or intranasal Shigella flexneri 2a and Shigella sonnei proteosome-lipopolysaccharide vaccines in animal models. Infect Immun. 1993; 61:2390–5.


19). Seiffert M, Cant C, Chen Z, Rappold I, Brugger W, Kanz L, et al. Human signal-regulatory protein is expressed on normal, but not on subsets of leukemic myeloid cells and mediates cellular adhesion involving its counterreceptor CD47. Blood. 1999; 94:3633–43.


20). Xu-Amano J, Kiyono H, Jackson RJ, Staats HF, Fujihashi K, Burrows PD, et al. Helper T cell subsets for immunoglobulin A responses: oral immunization with tetanus toxoid and cholera toxin as adjuvant selectively induces Th2 cells in mucosa associated tissues. J Exp Med. 1993; 178:1309–20.


21). Cho KA, Suh JW, Sohn JH, Park JW, Lee H, Kang JL, et al. IL-33 induces Th17-mediated airway inflammation via mast cells in ovalbumin-challenged mice. Am J Physiol Lung Cell Mol Physiol. 2012; 302:L429–40.


22). Chu VT, Beller A, Rausch S, Strandmark J, Zänker M, Arbach O, et al. Eosinophils promote generation and maintenance of immunoglobulin-A-expressing plasma cells and contribute to gut immune homeostasis. Immunity. 2014; 40:582–93.


23). Wen T, Mingler MK, Blanchard C, Wahl B, Pabst O, Rothenberg ME. The pan-B cell marker CD22 is expressed on gastrointestinal eosinophils and negatively regulates tissue eosinophilia. J Immunol. 2012; 188:1075–82.


24). Macpherson AJ, McCoy KD, Johansen FE, Brandtzaeg P. The immune geography of IgA induction and function. Mucosal Immunol. 2008; 1:11–22.


25). Cerutti A, Rescigno M. The biology of intestinal immunoglobulin A responses. Immunity. 2008; 28:740–50.


26). Cazac BB, Roes J. TGF-beta receptor controls B cell responsiveness and induction of IgA in vivo. Immunity. 2000; 13:443–51.
27). Mishra A, Hogan SP, Brandt EB, Rothenberg ME. Peyer's patch eosinophils: identification, characterization, and regulation by mucosal allergen exposure, interleukin-5, and eotaxin. Blood. 2000; 96:1538–44.


28). Uematsu S, Fujimoto K, Jang MH, Yang BG, Jung YJ, Nishiyama M, et al. Regulation of humoral and cellular gut immunity by lamina propria dendritic cells expressing Toll-like receptor 5. Nat Immunol. 2008; 9:769–76.


29). Mora JR, Iwata M, Eksteen B, Song SY, Junt T, Senman B, et al. Generation of gut-homing IgA-secreting B cells by intestinal dendritic cells. Science. 2006; 314:1157–60.


30). Fagarasan S, Kinoshita K, Muramatsu M, Ikuta K, Honjo T. In situ class switching and differentiation to IgA-producing cells in the gut lamina propria. Nature. 2001; 413:639–43.


31). Yuvaraj S, Dijkstra G, Burgerhof JG, Dammers PM, Stoel M, Visser A, et al. Evidence for local expansion of IgA plasma cell precursors in human ileum. J Immunol. 2009; 183:4871–8.


32). He B, Raab-Traub N, Casali P, Cerutti A. EBV-encoded latent membrane protein 1 cooperates with BAFF/BLyS and APRIL to induce T cell-independent Ig heavy chain class switching. J Immunol. 2003; 171:5215–24.


33). Litinskiy MB, Nardelli B, Hilbert DM, He B, Schaffer A, Casali P, et al. DCs induce CD40-independent immunoglobulin class switching through BLyS and APRIL. Nat Immunol. 2002; 3:822–9.


34). Xu W, He B, Chiu A, Chadburn A, Shan M, Buldys M, et al. Epithelial cells trigger frontline immunoglobulin class switching through a pathway regulated by the inhibitor SLPI. Nat Immunol. 2007; 8:294–303.


35). Chu VT, Fröhlich A, Steinhauser G, Scheel T, Roch T, Fillatreau S, et al. Eosinophils are required for the maintenance of plasma cells in the bone marrow. Nat Immunol. 2011; 12:151–9.


36). Schaffeler MP, Brokenshire JS, Snider DP. Detection of precursor Th cells in mesenteric lymph nodes after oral immunization with protein antigen and cholera toxin. Int Immunol. 1997; 9:1555–62.


37). Demeure CE, Yang LP, Byun DG, Ishihara H, Vezzio N, Delespesse G. Human naive CD4 T cells produce interleukin-4 at priming and acquire a Th2 phenotype upon repetitive stimulations in neutral conditions. Eur J Immunol. 1995; 25:2722–5.


38). Seder RA, Gazzinelli R, Sher A, Paul WE. Interleukin 12 acts directly on CD4+ T cells to enhance priming for interferon gamma production and diminishes interleukin 4 inhibition of such priming. Proc Natl Acad Sci U S A. 1993; 90:10188–92.
Figure 1.
Phenotypic characteristics of leukocytes isolated from the small intestinal lamina propria (LP) of wild-type (WT) and ΔdblGATA mice. (A) Small intestinal LP cells of WT and ΔdblGATA mice were stained with anti-CD11b and anti-CD172a, anti-SiglecF, anti-CCR3, or anti-IL-5Rα, then analyzed by flow cytometry. The R1 gate represents the medium to high side scatter (SSC) subset, and the R2 and R3 gates correspond to the mononuclear cell fractions of WT and ΔdblGATA mice, respectively. FSC indicates a forward light scatter. (B) The R1 and R2 of the LP cells of WT mice and the R3 of ΔdblGATA intestinal LP cells were analyzed for the expression of various cell-surface molecules. (C and D) Isolated blood (C) and bone marrow cells (D) were stained with anti-CCR3 and anti-SiglecF and analyzed by flow cytometry. A granulocyte population with medium to high SSC was gated and analyzed for their expression of CCR3 and SiglecF. Eosinophil populations (CCR3+ SiglecF+) were not observed in the blood or bone marrow of ΔdblGATA mice. The CCR3−SiglecF+ subset, observed only in the bone marrow of WT mice, might represent an immature type of eosinophil. The results shown are representative of three independent experiments.
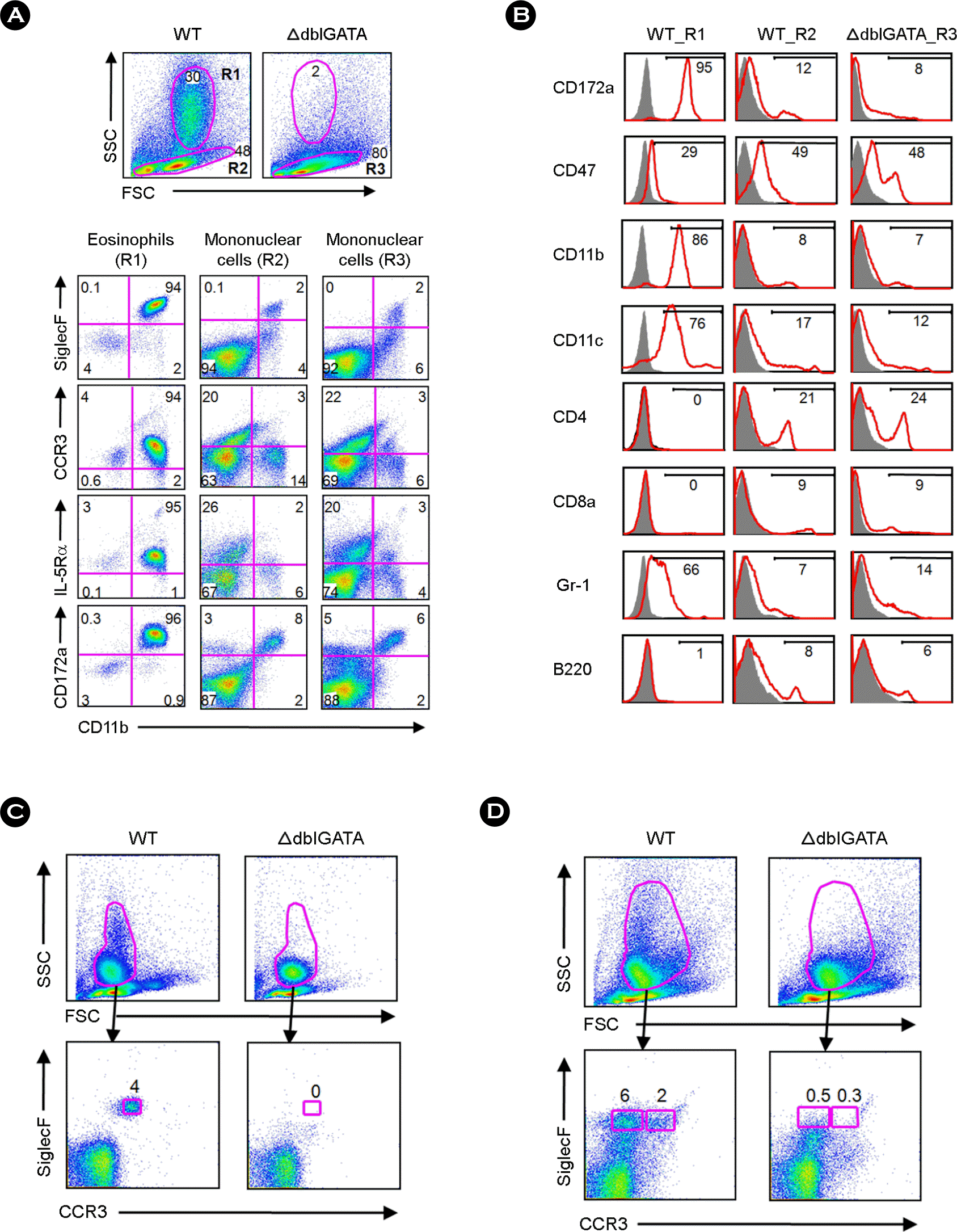
Figure 2.
Reduced proliferation of CD4+ T cells isolated from the mesenteric lymph node (MLN) of ΔdblGATA mice after oral immunization with ovalbumin (OVA) and cholera toxin (CT). (A and B) CD4+ T cells isolated from the MLN of WT or ΔdblGATA mice after oral immunization with PBS (control), OVA or OVA+CT were labeled with carboxyfluorescein diacetate succinimidyl ester (CFSE) and co-cultured with OVA-loaded APC for 72 h. Representative flow cytometry analysis of CD4+ CFSE+ cells (A). (B) CD4+ T cell proliferation presented as percentage of CD4+CFSE+ cells. (C and D) CD4+ T cells isolated from the MLN of WT or ΔdblGATA mice after oral immunization with PBS, OVA or OVA+CT were labeled with CFSE and cultured for 72 h with anti-CD3 and anti-CD28 antibodies. Representative flow cytometry analysis of CD4+ CFSE+ cells (C). (D) CD4+ T cell proliferation presented as a percentage of CD4+ CFSE+ cells. Data are mean ± s.e.m. values. ∗ p < 0.05, ∗∗∗ p < 0.001 (Student's t-test).
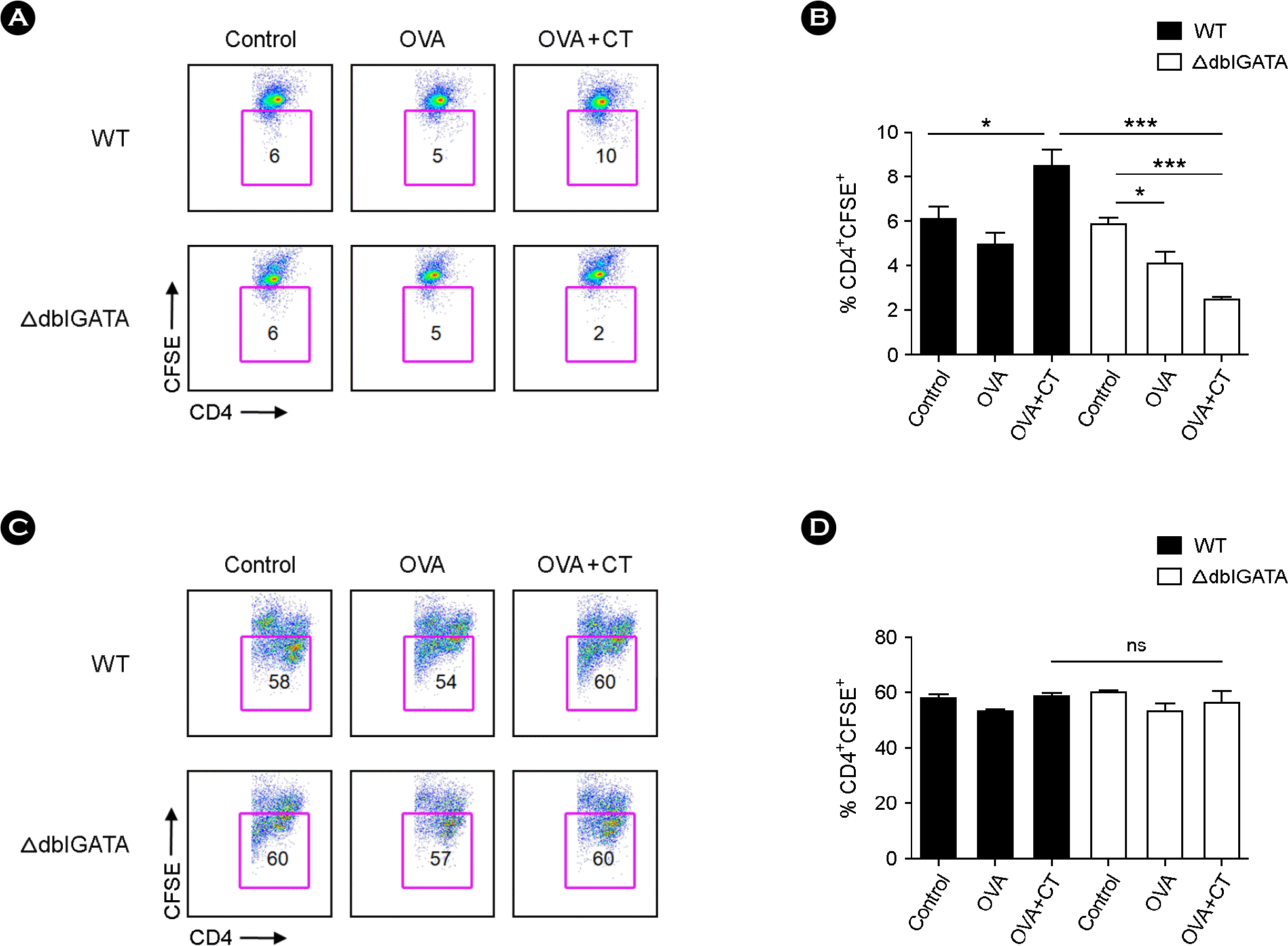
Figure 3.
OVA-specific intestinal IgA and IgG1 are reduced in ΔdblGATA mice orally immunized with OVA plus CT. (A) The frequencies of IgA+ cells in the Peyer's patch (PP) and small intestinal LP of WT and ΔdblGATA mice after oral immunization with PBS (control) or OVA+CT. The results shown are representative of three independent experiments. (B) OVA-specific IgA and IgG1 titers in serum and intestinal wash of WT and ΔdblGATA mice after oral immunization with PBS (control), OVA or OVA+CT. Each group consists of at least three individual mice. The absorbance at 450 nm represents antibody titers. Data are mean ± s.e.m. values. ∗ p < 0.05, ∗∗ p < 0.01, ∗∗∗ p < 0.001 (Student's t-test).
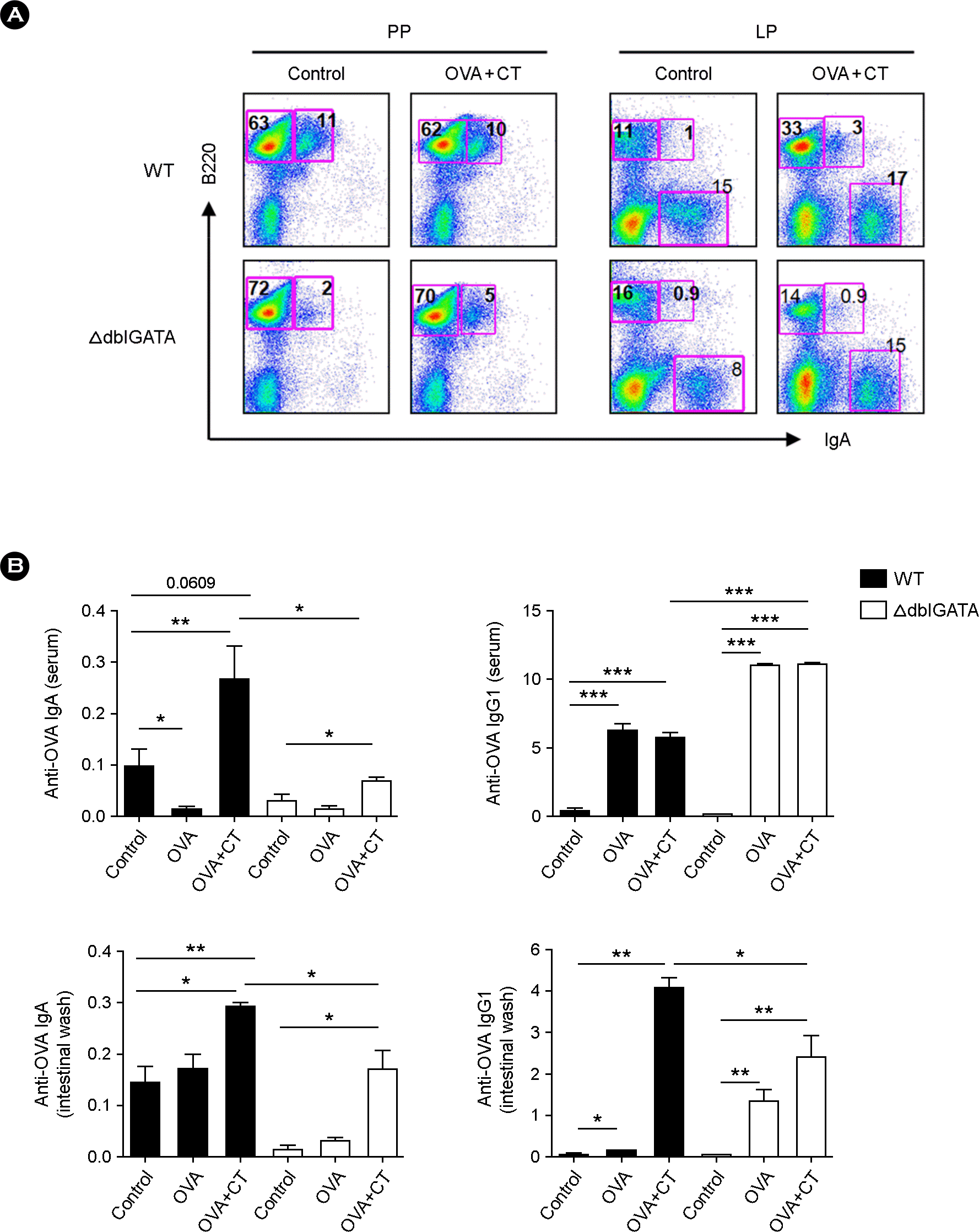