Abstract
Lyme disease is the most common vector-borne disease in the United States and Europe, caused by a tick-borne spirochete, Borrelia burgdorferi. Life cycle alternation between arthropod and mammals enhanced B. burgdorferi to adapt to two diverse niches. Although B. burgdorferi infection in these reservoir hosts appears asymptomatic, infection in human can typically cause inflammation in the skin, nervous system, musculoskeletal system and heart. In this review, we discuss the basic molecular characteristics and cell biology of B. burgdorferi and provide an overview of spirochete-induced activation of innate and adaptive immunity, resulting in particular immunopathology. Advancing understanding of the immune evasion mechanisms of B. burgdorferi provides important implications for ongoing research and clinical practice of Lyme disease.
REFERENCES
1). Steere AC, Hardin JA, Malawista SE. Erythema chronicum migrans and Lyme arthritis: cryoimmunoglobulins and clinical activity of skin and joints. Science. 1977; 196:1121–2.


2). Hu LT. In the clinic. Lyme disease. Ann Intern Med. 2012; 157:ITC2–2-ITC2-16.
3). Radolf JD, Caimano MJ, Stevenson B, Hu LT. Of ticks, mice and men: understanding the dual-host lifestyle of Lyme disease spirochaetes. Nat Rev Microbiol. 2012; 10:87–99.


4). Burgdorfer W, Barbour AG, Hayes SF, Benach JL, Grunwaldt E, Davis JP. Lyme disease-a tick-borne spirochetosis? Science. 1982; 216:1317–9.


5). Fraser CM, Casjens S, Huang WM, Sutton GG, Clayton R, Lathigra R, et al. Genomic sequence of a Lyme disease spirochaete, Borrelia burgdorferi. Nature. 1997; 390:580–6.
6). Casjens S, Palmer N, van Vugt R, Huang WM, Stevenson B, Rosa P, et al. A bacterial genome in flux: the twelve linear and nine circular extrachromosomal DNAs in an infectious isolate of the Lyme disease spirochete Borrelia burgdorferi. Mol Microbiol. 2000; 35:490–516.
7). Rosa PA, Tilly K, Stewart PE. The burgeoning molecular genetics of the Lyme disease spirochaete. Nat Rev Microbiol. 2005; 3:129–43.


8). Schwan TG, Piesman J, Golde WT, Dolan MC, Rosa PA. Induction of an outer surface protein on Borrelia burgdorferi during tick feeding. Proc Natl Acad Sci U S A. 1995; 92:2909–13.
9). Ohnishi J, Piesman J, de Silva AM. Antigenic and genetic heterogeneity of Borrelia burgdorferi populations transmitted by ticks. Proc Natl Acad Sci U S A. 2001; 98:670–5.
10). Ramamoorthi N, Narasimhan S, Pal U, Bao F, Yang XF, Fish D, et al. The Lyme disease agent exploits a tick protein to infect the mammalian host. Nature. 2005; 436:573–7.


11). Grimm D, Tilly K, Byram R, Stewart PE, Krum JG, Bueschel DM, et al. Outer-surface protein C of the Lyme disease spirochete: a protein induced in ticks for infection of mammals. Proc Natl Acad Sci U S A. 2004; 101:3142–7.


12). Norgard MV, Arndt LL, Akins DR, Curetty LL, Harrich DA, Radolf JD. Activation of human monocytic cells by Treponema pallidum and Borrelia burgdorferi lipoproteins and synthetic lipopeptides proceeds via a pathway distinct from that of lipopolysaccharide but involves the transcriptional activator NF-kappa B. Infect Immun. 1996; 64:3845–52.
13). Norgard MV, Riley BS, Richardson JA, Radolf JD. Dermal inflammation elicited by synthetic analogs of Treponema pallidum and Borrelia burgdorferi lipoproteins. Infect Immun. 1995; 63:1507–15.
14). Morrison TB, Weis JH, Weis JJ. Borrelia burgdorferi outer surface protein A (OspA) activates and primes human neutrophils. J Immunol. 1997; 158:4838–45.
15). Ma Y, Seiler KP, Tai KF, Yang L, Woods M, Weis JJ. Outer surface lipoproteins of Borrelia burgdorferi stimulate nitric oxide production by the cytokine-inducible pathway. Infect Immun. 1994; 62:3663–71.
16). Ma Y, Weis JJ. Borrelia burgdorferi outer surface lipoproteins OspA and OspB possess B-cell mitogenic and cytokine-stimulatory properties. Infect Immun. 1993; 61:3843–53.
17). Wooten RM, Modur VR, McIntyre TM, Weis JJ. Borrelia burgdorferi outer membrane protein A induces nuclear translocation of nuclear factor-kappa B and inflammatory activation in human endothelial cells. J Immunol. 1996; 157:4584–90.
18). Hirschfeld M, Kirschning CJ, Schwandner R, Wesche H, Weis JH, Wooten RM, et al. Cutting edge: inflammatory signaling by Borrelia burgdorferi lipoproteins is mediated by toll-like receptor 2. J Immunol. 1999; 163:2382–6.
19). Bolz DD, Sundsbak RS, Ma Y, Akira S, Kirschning CJ, Zachary JF, et al. MyD88 plays a unique role in host defense but not arthritis development in Lyme disease. J Immunol. 2004; 173:2003–10.


20). Wang G, Ma Y, Buyuk A, McClain S, Weis JJ, Schwartz I. Impaired host defense to infection and Toll-like receptor 2-independent killing of Borrelia burgdorferi clinical isolates in TLR2-deficient C3H/HeJ mice. FEMS Microbiol Lett. 2004; 231:219–25.
21). Wang X, Ma Y, Weis JH, Zachary JF, Kirschning CJ, Weis JJ. Relative contributions of innate and acquired host responses to bacterial control and arthritis development in Lyme disease. Infect Immun. 2005; 73:657–60.


22). Behera AK, Hildebrand E, Bronson RT, Perides G, Uematsu S, Akira S, et al. MyD88 deficiency results in tissue-specific changes in cytokine induction and inflammation in interleukin-18-independent mice infected with Borrelia burgdorferi. Infect Immun. 2006; 74:1462–70.
23). Liu N, Montgomery RR, Barthold SW, Bockenstedt LK. Myeloid differentiation antigen 88 deficiency impairs pathogen clearance but does not alter inflammation in Borrelia burgdorferi-infected mice. Infect Immun. 2004; 72:3195–203.
24). Wooten RM, Ma Y, Yoder RA, Brown JP, Weis JH, Zachary JF, et al. Toll-like receptor 2 is required for innate, but not acquired, host defense to Borrelia burgdorferi. J Immunol. 2002; 168:348–55.
25). Cervantes JL, La Vake CJ, Weinerman B, Luu S, O'Connell C, Verardi PH, et al. Human TLR8 is activated upon recognition of Borrelia burgdorferi RNA in the phagosome of human monocytes. J Leukoc Biol. 2013; 94:1231–41.
26). Cervantes JL, Dunham-Ems SM, La Vake CJ, Petzke MM, Sahay B, Sellati TJ, et al. Phagosomal signaling by Borrelia burgdorferi in human monocytes involves Toll-like receptor (TLR) 2 and TLR8 cooperativity and TLR8-mediated induction of IFN-beta. Proc Natl Acad Sci U S A. 2011; 108:3683–8.
27). Petzke MM, Brooks A, Krupna MA, Mordue D, Schwartz I. Recognition of Borrelia burgdorferi, the Lyme disease spirochete, by TLR7 and TLR9 induces a type I IFN response by human immune cells. J Immunol. 2009; 183:5279–92.
28). Oosting M, Buffen K, Malireddi SR, Sturm P, Verschueren I, Koenders MI, et al. Murine Borrelia arthritis is highly dependent on ASC and caspase-1, but independent of NLRP3. Arthritis Res Ther. 2012; 14:R247.
29). Berende A, Oosting M, Kullberg BJ, Netea MG, Joosten LA. Activation of innate host defense mechanisms by Borrelia. Eur Cytokine Netw. 2010; 21:7–18.
30). Sterka D Jr, Marriott I. Characterization of nucleotide-binding oligomerization domain (NOD) protein expression in primary murine microglia. J Neuroimmunol. 2006; 179:65–75.


31). Kumar H, Kawai T, Akira S. Pathogen recognition by the innate immune system. Int Rev Immunol. 2011; 30:16–34.


32). Sterka D Jr, Rati DM, Marriott I. Functional expression of NOD2, a novel pattern recognition receptor for bacterial motifs, in primary murine astrocytes. Glia. 2006; 53:322–30.


33). Petnicki-Ocwieja T, DeFrancesco AS, Chung E, Darcy CT, Bronson RT, Kobayashi KS, et al. Nod2 suppresses Borrelia burgdorferi mediated murine Lyme arthritis and carditis through the induction of tolerance. PloS One. 2011; 6:e17414.
34). Oosting M, Berende A, Sturm P, Ter Hofstede HJ, de Jong DJ, Kanneganti TD, et al. Recognition of Borrelia burgdorferi by NOD2 is central for the induction of an inflammatory reaction. J Infect Dis. 2010; 201:1849–58.
35). Oosting M, van de Veerdonk FL, Kanneganti TD, Sturm P, Verschueren I, Berende A, et al. Borrelia species induce inflammasome activation and IL-17 production through a caspase-1-dependent mechanism. Eur J Immunol. 2011; 41:172–81.
36). Liu N, Belperron AA, Booth CJ, Bockenstedt LK. The caspase 1 inflammasome is not required for control of murine Lyme borreliosis. Infect Immun. 2009; 77:3320–7.


37). Behera AK, Hildebrand E, Uematsu S, Akira S, Coburn J, Hu LT. Identification of a TLR-independent pathway for Borrelia burgdorferi-induced expression of matrix metalloproteinases and inflammatory mediators through binding to integrin alpha 3 beta 1. J Immunol. 2006; 177:657–64.
38). Coburn J, Magoun L, Bodary SC, Leong JM. Integrins alpha(v)beta3 and alpha5beta1 mediate attachment of lyme disease spirochetes to human cells. Infect Immun. 1998; 66:1946–52.
39). Coburn J, Leong JM, Erban JK. Integrin alpha IIb beta 3 mediates binding of the Lyme disease agent Borrelia burgdorferi to human platelets. Proc Natl Acad Sci U S A. 1993; 90:7059–63.
40). Coburn J, Barthold SW, Leong JM. Diverse Lyme disease spirochetes bind integrin alpha IIb beta 3 on human platelets. Infect Immun. 1994; 62:5559–67.


41). Coburn J, Leong JM, Erban JK. Integrin alpha IIb beta 3 mediates binding of the Lyme disease agent Borrelia burgdorferi to human platelets. Proc Natl Acad Sci U S A. 1993; 90:7059–63.
42). Marre ML, Petnicki-Ocwieja T, DeFrancesco AS, Darcy CT, Hu LT. Human integrin alpha(3)beta(1) regulates TLR2 recognition of lipopeptides from endosomal compartments. PLoS One. 2010; 5:e12871.
43). Barthold SW, Sidman CL, Smith AL. Lyme borreliosis in genetically resistant and susceptible mice with severe combined immunodeficiency. Am J Trop Med Hyg. 1992; 47:605–13.


44). Bockenstedt LK, Kang I, Chang C, Persing D, Hayday A, Barthold SW. CD4+ T helper 1 cells facilitate regression of murine Lyme carditis. Infect Immun. 2001; 69:5264–9.
45). McKisic MD, Redmond WL, Barthold SW. Cutting edge: T cell-mediated pathology in murine Lyme borreliosis. J Immunol. 2000; 164:6096–9.


46). Ruderman EM, Kerr JS, Telford SR 3rd, Spielman A, Glimcher LH, Gravallese EM. Early murine Lyme carditis has a macrophage predominance and is independent of major histocompatibility complex class II-CD4+ T cell interactions. J Infect Dis. 1995; 171:362–70.


47). Schaible UE, Gay S, Museteanu C, Kramer MD, Zimmer G, Eichmann K, et al. Lyme borreliosis in the severe combined immunodeficiency (scid) mouse manifests predominantly in the joints, heart, and liver. Am J Pathol. 1990; 137:811–20.
48). McKisic MD, Redmond WL, Barthold SW. Cutting edge: T cell-medicated pathology in murine Lyme borreliosis. J Immunol. 2000; 164:6096–9.
49). Gross DM, Steere AC, Huber BT. T helper 1 response is dominant and localized to the synovial fluid in patients with Lyme arthritis. J Immunol. 1998; 160:1022–8.
50). Keane-Myers A, Nickell SP. Role of IL-4 and IFN-gamma in modulation of immunity to Borrelia burgdorferi in mice. J Immunol. 1995; 155:2020–8.
51). Matyniak JE, Reiner SL. T helper phenotype and genetic susceptibility in experimental Lyme disease. J Exp Med. 1995; 181:1251–4.


52). Yssel H, Shanafelt MC, Soderberg C, Schneider PV, Anzola J, Peltz G. Borrelia burgdorferi activates a T helper type 1-like T cell subset in Lyme arthritis. J Exp Med. 1991; 174:593–601.
53). Potter MR, Noben-Trauth N, Weis JH, Teuscher C, Weis JJ. Interleukin-4 (IL-4) and IL-13 signaling pathways do not regulate Borrelia burgdorferi-induced arthritis in mice: IgG1 is not required for host control of tissue spirochetes. Infect Immun. 2000; 68:5603–9.
54). Kinjo Y, Tupin E, Wu D, Fujio M, Garcia-Navarro R, Benhnia MR, et al. Natural killer T cells recognize diacylglycerol antigens from pathogenic bacteria. Nat Immunol. 2006; 7:978–86.


55). Tupin E, Benhnia MR, Kinjo Y, Patsey R, Lena CJ, Haller MC, et al. NKT cells prevent chronic joint inflammation after infection with Borrelia burgdorferi. Proc Natl Acad Sci U S A. 2008; 105:19863–8.
56). Codolo G, Amedei A, Steere AC, Papinutto E, Cappon A, Polenghi A, et al. Borrelia burgdorferi NapA-driven Th17 cell inflammation in lyme arthritis. Arthritis Rheum. 2008; 58:3609–17.
57). Connolly SE, Benach JL. The versatile roles of antibodies in Borrelia infections. Nat Rev Microbiol. 2005; 3:411–20.
58). Belperron AA, Dailey CM, Booth CJ, Bockenstedt LK. Marginal zone B-cell depletion impairs murine host defense against Borrelia burgdorferi infection. Infect Immun. 2007; 75:3354–60.
59). Tunev SS, Hastey CJ, Hodzic E, Feng S, Barthold SW, Baumgarth N. Lymphoadenopathy during lyme borreliosis is caused by spirochete migration-induced specific B cell activation. PLoS Pathog. 2011; 7:e1002066.


60). Moody KD, Barthold SW. Lyme borreliosis in laboratory mice. Lab Anim Sci. 1998; 48:168–71.
61). Barthold SW, Beck DS, Hansen GM, Terwilliger GA, Moody KD. Lyme borreliosis in selected strains and ages of laboratory mice. J Infect Dis. 1990; 162:133–8.


62). Weis JJ, McCracken BA, Ma Y, Fairbairn D, Roper RJ, Morrison TB, et al. Identification of quantitative trait loci governing arthritis severity and humoral responses in the murine model of Lyme disease. J Immunol. 1999; 162:948–56.
63). Crandall H, Dunn DM, Ma Y, Wooten RM, Zachary JF, Weis JH, et al. Gene expression profiling reveals unique pathways associated with differential severity of Lyme arthritis. J Immunol. 2006; 177:7930–42.


64). Miller JC, Ma Y, Crandall H, Wang X, Weis JJ. Gene expression profiling provides insights into the pathways involved in inflammatory arthritis development: murine model of Lyme disease. Exp Mol Pathol. 2008; 85:20–7.


65). Brown JP, Zachary JF, Teuscher C, Weis JJ, Wooten RM. Dual role of interleukin-10 in murine Lyme disease: regulation of arthritis severity and host defense. Infect Immun. 1999; 67:5142–50.


66). Sellati TJ, Bouis DA, Caimano MJ, Feulner JA, Ayers C, Lien E, et al. Activation of human monocytic cells by Borrelia burgdorferi and Treponema pallidum is facilitated by CD14 and correlates with surface exposure of spirochetal lipoproteins. J Immunol. 1999; 163:2049–56.
67). Radolf JD, Arndt LL, Akins DR, Curetty LL, Levi ME, Shen Y, et al. Treponema pallidum and Borrelia burgdorferi lipoproteins and synthetic lipopeptides activate monocytes/macrophages. J Immunol. 1995; 154:2866–77.
68). Tatro JB, Romero LI, Beasley D, Steere AC, Reichlin S. Borrelia burgdorferi and Escherichia coli lipopoly-saccharides induce nitric oxide and interleukin-6 production in cultured rat brain cells. J Infect Dis. 1994; 169:1014–22.
69). Behera AK, Thorpe CM, Kidder JM, Smith W, Hildebrand E, Hu LT. Borrelia burgdorferi-induced expression of matrix metalloproteinases from human chondrocytes requires mitogen-activated protein kinase and Janus kinase/signal transducer and activator of transcription signaling pathways. Infect Immun. 2004; 72:2864–71.
70). Brown CR, Blaho VA, Loiacono CM. Susceptibility to experimental Lyme arthritis correlates with KC and monocyte chemoattractant protein-1 production in joints and requires neutrophil recruitment via CXCR2. J Immunol. 2003; 171:893–901.


71). Gebbia JA, Coleman JL, Benach JL. Selective induction of matrix metalloproteinases by Borrelia burgdorferi via toll-like receptor 2 in monocytes. J Infect Dis. 2004; 189:113–9.
72). Gebbia JA, Coleman JL, Benach JL. Borrelia spirochetes upregulate release and activation of matrix metalloproteinase gelatinase B (MMP-9) and collagenase 1 (MMP-1) in human cells. Infect Immun. 2001; 69:456–62.
73). Anguita J, Barthold SW, Persinski R, Hedrick MN, Huy CA, Davis RJ, et al. Murine Lyme arthritis development mediated by p38 mitogen-activated protein kinase activity. J Immunol. 2002; 168:6352–7.


74). Hedrick MN, Olson CM Jr, Conze DB, Bates TC, Rincón M, Anguita J. Control of Borrelia burgdorferi-specific CD4+-T-cell effector function by interleukin-12- and T-cell receptor-induced p38 mitogen-activated protein kinase activity. Infect Immun. 2006; 74:5713–7.
75). Brown CR, Blaho VA, Fritsche KL, Loiacono CM. Stat1 deficiency exacerbates carditis but not arthritis during experimental lyme borreliosis. J Interferon Cytokine Res. 2006; 26:390–9.


76). Montgomery RR, Nathanson MH, Malawista SE. The fate of Borrelia burgdorferi, the agent for Lyme disease, in mouse macrophages. Destruction, survival, recovery. J Immunol. 1993; 150:909–15.
77). Montgomery RR, Malawista SE. Entry of Borrelia burgdorferi into macrophages is end-on and leads to degradation in lysosomes. Infect Immun. 1996; 64:2867–72.
78). Montgomery RR, Lusitani D, de Boisfleury Chevance A, Malawista SE. Human phagocytic cells in the early innate immune response to Borrelia burgdorferi. J Infect Dis. 2002; 185:1773–9.
79). Montgomery RR, Nathanson MH, Malawista SE. Fc- and non-Fc-mediated phagocytosis of Borrelia burgdorferi by macrophages. J Infect Dis. 1994; 170:890–3.
80). Shin OS, Miller LS, Modlin RL, Akira S, Uematsu S, Hu LT. Downstream signals for MyD88-mediated phagocytosis of Borrelia burgdorferi can be initiated by TRIF and are dependent on PI3K. J Immunol. 2009; 183:491–8.
81). Shin OS, Isberg RR, Akira S, Uematsu S, Behera AK, Hu LT. Distinct roles for MyD88 and Toll-like receptors 2, 5, and 9 in phagocytosis of Borrelia burgdorferi and cytokine induction. Infect Immun. 2008; 76:2341–51.
82). Brown EL, Wooten RM, Johnson BJ, Iozzo RV, Smith A, Dolan MC, et al. Resistance to Lyme disease in decorin-deficient mice. J Clin Invest. 2001; 107:845–52.


83). Liang FT, Yan J, Mbow ML, Sviat SL, Gilmore RD, Mamula M, et al. Borrelia burgdorferi changes its surface antigenic expression in response to host immune responses. Infect Immun. 2004; 72:5759–67.
84). McDowell JV, Sung SY, Hu LT, Marconi RT. Evidence that the variable regions of the central domain of VlsE are antigenic during infection with lyme disease spirochetes. Infect Immun. 2002; 70:4196–203.


85). Gross DM, Forsthuber T, Tary-Lehmann M, Etling C, Ito K, Nagy ZA, et al. Identification of LFA-1 as a candidate autoantigen in treatment-resistant Lyme arthritis. Science. 1998; 281:703–6.


86). Trollmo C, Meyer AL, Steere AC, Hafler DA, Huber BT. Molecular mimicry in Lyme arthritis demonstrated at the single cell level: LFA-1 alpha L is a partial agonist for outer surface protein A-reactive T cells. J Immunol. 2001; 166:5286–91.
Figure 1.
Life cycle of Borrelia burgdorferi. Ixodes ticks have three life stages that require blood: larvae, nymphs, and adults. Because B. burgdorferi is not vertically transmitted by ticks to their offspring, larvae become infected when feeding on infected reservoir animals and transmit the infection to new animals during their next bloodmeal. Nymphal ticks appear to be responsible for the majority of human transmission in the eastern U.S.; the majority of human cases occur in late spring and summer when this stage is most commonly encountered. Many mammals and birds have been implicated as reservoirs for B. burgdorferi in the U.S., although in most of the sites where transmission is intense, white-footed mice appear to most frequently contribute to the spirochetal life cycle (3).
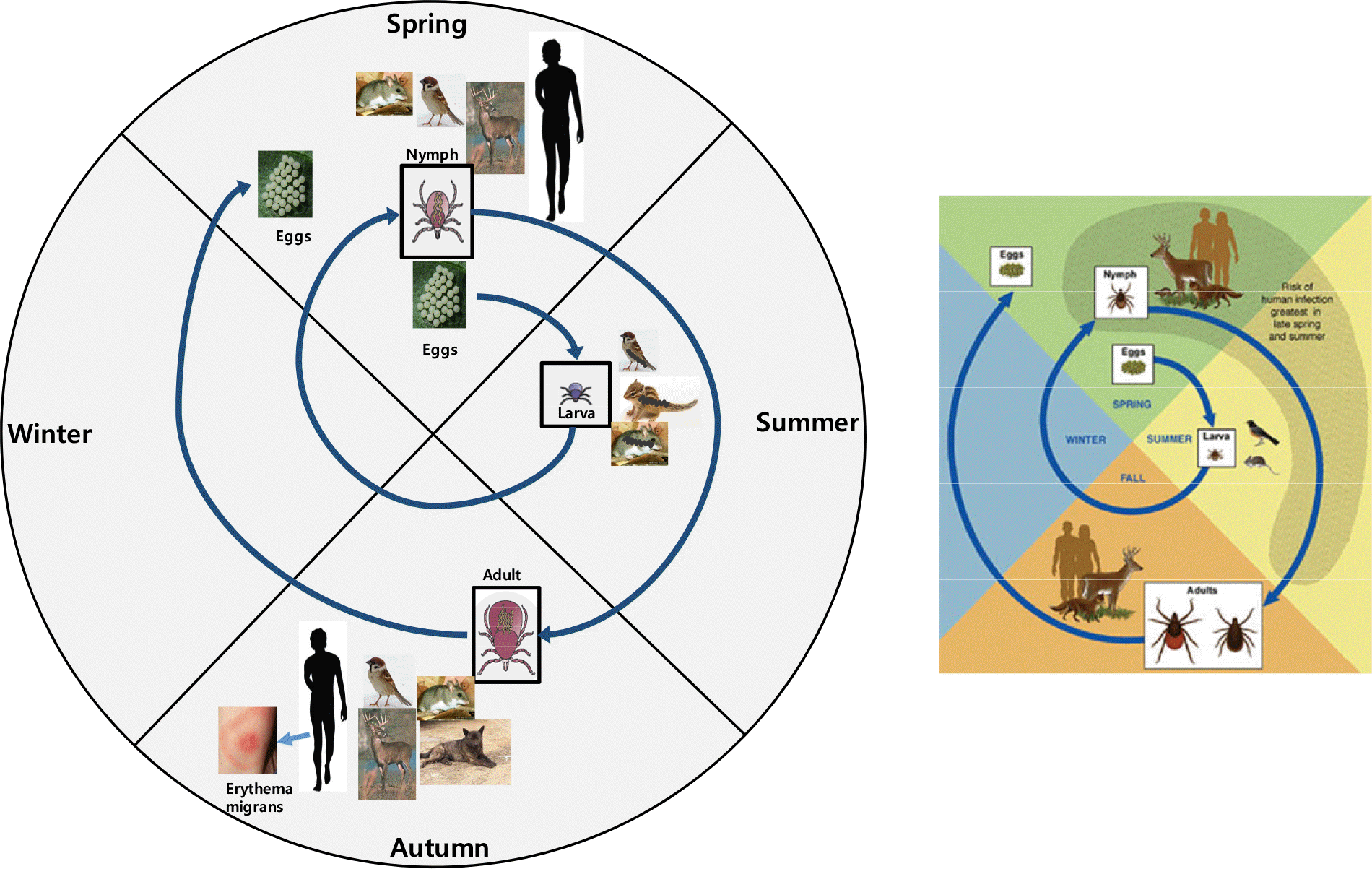
Figure 2.
Innate and adaptive immune activation in response to B. burgdorferi infection. B. burgdorferi are initially recognized by innate immune effector cells such as dendritic cells (DCs), neutrophils and macrophages; activation of these cells increases following internalization and degradation of spirochetes within phagolysosomes. DCs that have taken up spirochetes migrate to the lymph nodes, where they present processed B. burgdorferi antigens to T cells and B cells. While T cells enter the circulation and are recruited to the site of infection, B cells can be differentiated into plasma cells that can secrete specific antibodies that can kill B. burgdorferi via complement-dependent and -independent pathways. Production of pro-inflammatory cytokines by activated macrophages results in the recruitment of additional neutrophils, T cells, macrophages and DCs to the bite site, and eventually the development of erythema migrans (3).
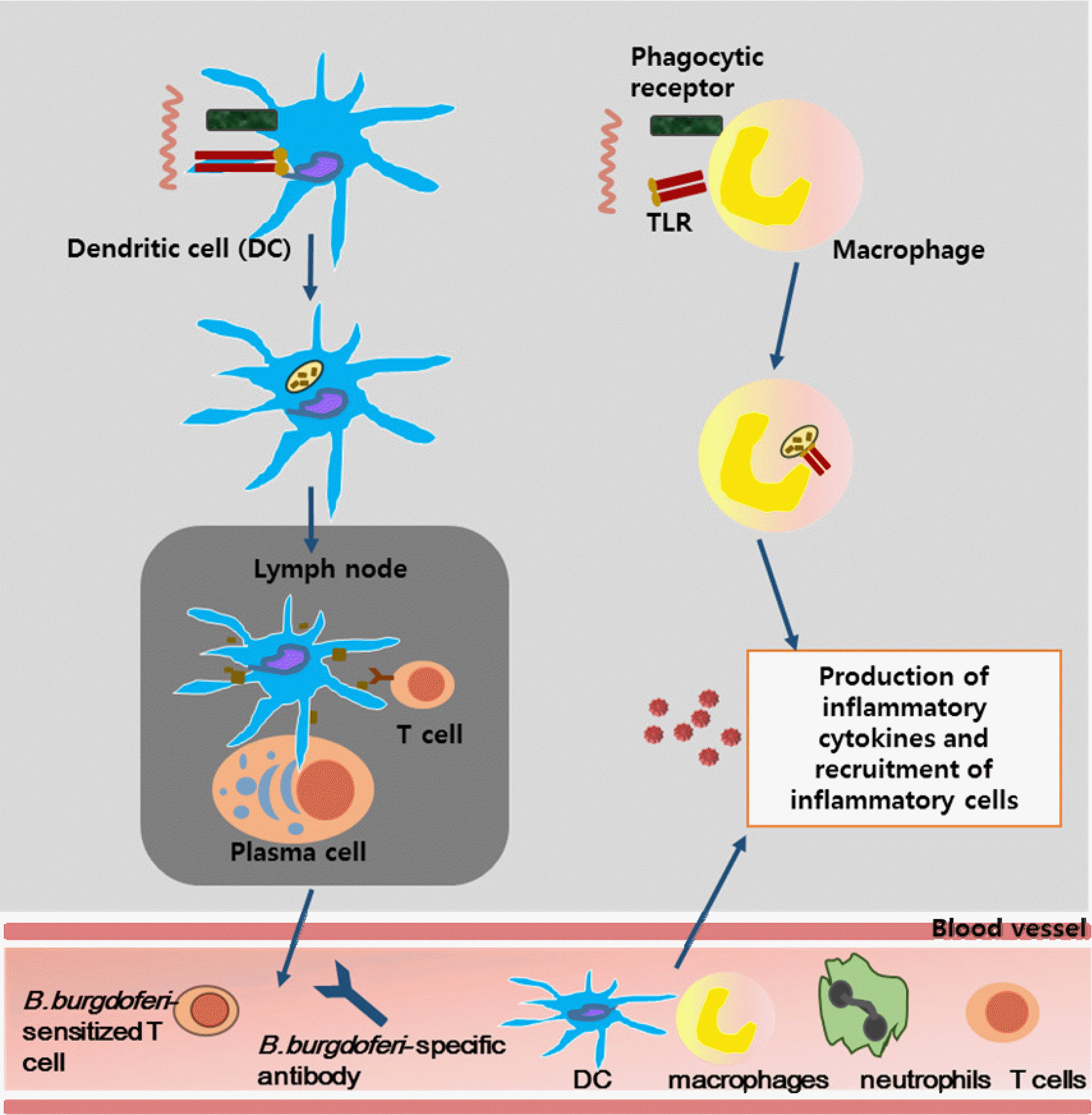