Abstract
A neutralization-resistant mutant of Newcastle disease virus (NDV) Kr005 strain belonging to class II genotype VII was generated using a neutralizing monoclonal antibody and its biological effects were assessed. The mutant showed single amino acid substitution (E to K) at position 347 of the hemagglutinin-neuraminidase (HN) protein (E347K mutant). The E347K mutant exhibited marked rounding of the cells and few syncytia in infected chicken embryofibroblast (CEF) cells. The hemadsorption and neuraminidase activities of the E347K mutant of the wild-type virus were 118% and 166%, respectively. The mutant produced a rapid elution pattern whereas the wild type had a slow elution pattern. Growth kinetics studies showed that the E347K mutant produced an 80-times higher yield of extracellular virus in CEF cells compared with the wild-type virus. The time-course virus titer showed a marked increase in mutant-infected cells from 6 h to 12 h post infection (pi), which was consistent with the titer pattern time-course for NA activity. The E347K mutant virus showed a slight decrease in virulence compared to the wild-type virus, but there was no change in pathotype when measured by in vivo pathogenicity testing. These results suggest that an E347K mutation in HN protein might be associated with increased NA activity and subsequent enhancement of virus release from infected cells without change in viral pathotype.
Newcastle disease virus (NDV) is the causative agent of the devastating Newcastle disease (ND), which causes substantial economic losses in the poultry industry. The virus is a single-stranded RNA virus that belongs to the genus Avulavirus in the family Paramyxoviridae (1, 2). The viral RNA genome encodes at least six proteins, i.e., nucleocapsid protein, phosphoprotein, matrix protein, fusion protein (F), hemagglutinin-neuraminidase (HN), and large polymerase.
The HN protein is one of two envelope glycoproteins and it is a multifunctional molecule (3~6). The HN protein recognizes sialic acid-containing receptors on cell surfaces, which promote fusion activity via the HN-F interaction. The HN protein allows the virus to penetrate the cell surface, while it also acts as a neuraminidase during the release of viral progeny from the infected cell. The HN protein contains at least five neutralizing antigenic sites including site 23 (residues 193 to 211), site 1/14 (residues 345 to 352), site 12 (residue 494), site 2/12 (residues 513 to 521), and site 2 (residue 569) (7). Of these sites, site 1/14 is known to be the major linear epitope of HN protein (8). Recently, many field NDVs have been found to carry an E347K mutation in site 1/14 of the HN protein in domestic fowl farms throughout China and Korea where extensive ND vaccination has been implemented (9~11). It was postulated that antigenic variants of the E347K mutation might have emerged as a result of host immune pressure to vaccination (11~17). Despite the importance of the antigenic variation in residue 347, the effects of the E347K mutation on HN protein biology still remain unclear.
In this study, we generated an E347K mutant virus that escaped from a neutralizing monoclonal antibody (mAb), 10F11, and determined the effects of the mutation on the biological properties of HN protein in NDV.
The virulent NDV strain Kr005 (18) was used as the wild-type virus to generate a neutralization escape mutant. The Kr005 virus was propagated in 9 to10-day-old embryonated specific pathogen-free (SPF) chicken eggs until use. MAb 10F11 (IgG1, kappa) bound to the HN protein of NDV was produced at our laboratory from Balb/c mice immunized with recombinant HN protein of NDV, which was expressed by a recombinant baculovirus (19) using the previously described protocol (20). In preliminary work, the 10F11 antibody had virus-neutralizing activity and inhibited hemagglutination of NDV Kr005 strain (see Table 1). The supernatant collected from 10F11 antibody-secreting hybridoma cells was clarified by low speed centrifugation, and stored at -20℃ until use. Chicken NDV antiserum (to NDV Kr005 strain) and specific pathogen free (SPF) chicken serum were used as positive and negative controls, respectively.
Primary CEF cells were infected with NDV (Kr005 strain) with a multiplicity of infection (MOI) of approximately 1 for 1 h at 37℃, and then incubated for 4 days at 37℃ in the presence of 10F11 antibody (HI titer of 64) in M199 medium supplemented with 2% calf serum and an antibiotic-antimycotic solution. Control cells were inoculated in parallel using mock-neutralized virus. The passaging of harvested virus into fresh primary CEF cells was repeated using the same method until the marked cytopathic effect (CPE) was visible. After the final passage, cytopathic viruses were purified using a plaque assay with primary CEF cells, before their propagation in SPF chicken eggs.
In the same manner, NDV Kr005 strain was passaged in primary CEF cells in the absence of mAb 10F11 for use as a wild-type control. The neutralization-resistance of escape mutant viruses to 10F11 antibody was then confirmed using a virus neutralization (VN) test and a hemagglutination inhibition (HI) test in 96-well microplates.
Viral genomic RNA was extracted from virus samples using a Viral Gene-spin Kit (iNtRON Biotech, Korea), according to the manufacturer's protocol. Complementary DNA was amplified using a one-step RT-PCR Kit (Bioneer, Korea), according to the manufacturer's instructions. The PCR primers used for the Nucleoprotein (NP), F, HN and L genes were shown in Table 1. The amplified PCR products were electrophoresed on 1% agarose gel. Slices of gel containing the DNA bands of the expected sizes were purified using a Qiaquick Gel Purification Kit (Qiagen). Purified DNA products were subjected to direct nucleotide sequencing using a Dye Terminator Cycle Sequencing Kit (Applied Biosystems), followed by analysis with an ABI PRISM version 377 DNA autosequencer (PE Applied Biosystems Inc., CA). The assembly of sequencing contigs and translation of the collated nucleotide sequences into deduced amino acid sequences was performed using the VectorNTI suite 10 program (Invitrogen, CA, USA). The sequencing data were aligned using the MegAlign program in the Lasergene package (DNASTAR Inc., Madison, WI, USA) and the CLUSTALW multiple alignment algorithm.
A hemadsorption (HAd) assay was performed using CEF cells in 96-well plates, according to a published procedure (21). For the elution test NDV viruses were incubated with chicken red blood cells for 1 h at 34℃ and hemagglutination (HA) titration assays were performed at 34℃ in 96-well plates as previously described (22). The neuraminidase (NA) activity of NDV was tested in 96-well ELISA plates (Costar, USA). The absorbance of red coloration was measured at a wavelength of 549 nm. The pathogenicity of NDV was measured by mean death time (MDT) in embryonated SPF chicken eggs and intracerebral pathogenicity index (ICPI) tests, which were previously developed by Alexander (23).
The growth kinetics of NDV was determined in primary CEF cells. Briefly, primary CEF cells were grown in six-well plates and infected using NDV at 1 MOI. After adsorption for 1 h, the infected cells were washed using phosphate-buffered saline (PBS) and the medium was replaced, before cultures were incubated at 37℃. At 6, 12, 24, 48, and 72 h pi, 100 µl of culture supernatant was collected and the viruses were stored at -70℃, before virus titration of CEF cells in 96-well plates. Virus titers of the samples were determined using a serial endpoint assay of CEF cells in 96-well plates, with four wells per virus per dilution. Titers were calculated as the 50% endpoint tissue culture infectious dose (TCID50/ml) using the Reed and Muench method (24). All tests were repeated three times.
NDV Kr005 strain was serially passaged in CEF cells in the presence of mAb 10F11. CPE was not apparent in the cell mono-layer during the first several passages. However, CPE developed rapidly and consistently in the cell monolayer after eight passages. Infected cells were rounded and granulated with few syncytia, which were small-sized in the cell monolayer (Fig. 1B). By contrast, the wild type Kr005-infected cells in the absence of 10F11 antibody developed large-sized syncytia during every passage in CEF cells (Fig. 1A). After 10 passages, the Kr005 viruses propagated in either presence or absence of the 10F11 antibody were purified by plaque assay and then used as mutant and wild-type viruses, respectively.
A virus neutralization test was used to test whether the E347K mutant virus could escape mAb 10F11. Table 2 shows that mutant virus escaped from mAb 10F11 (VN titer of <2), but not from anti-NDV chicken serum (VN titer of 16). The wild-type virus, which was collected after 10 passages in primary CEF cells in the absence of mAb 10F11, was neutralized by anti-NDV chicken serum (VN titer of 32) and mAb 10F11 (VN titer of 32). Both wild-type and mutant viruses were never neutralized by normal SPF chicken serum (VN titer of <2). This indicated that the mutant was an NDV escape mutant, which was resistant to neutralization by mAb 10F11. The HI test results were similar to the VN test. The HA activity of the wild type virus was inhibited by mAb 10F11 (HI titer of 32) and anti-NDV serum (HI titer of 32). However, the HA activity of the mutant virus was not inhibited by mAb 10F11 (HI titer of <2), whereas it was efficiently inhibited by anti-NDV chicken serum (HI titer of 32). The HA activity of the wild-type and mutant viruses were not affected by SPF chicken serum (HI titer of <2).
To determine the localization of the mutation that conferred resistance to the 10F11 antibody, the NP, F, HN and L genes of the mutant were sequenced and the sequences were compared with those of the wild-type virus. A single amino acid substitution mutation of E to K was found at residue 347 in the mutant HN protein, which was localized within a linear epitope known as antigenic site 1/14 (9, 11) (Table 3). The results indicated that amino acid residue E347 in the HN protein may be critical to the antibody 10F11 neutralization-resistance of the NDV escape mutant. Thus, the mutant was designated as an E347K mutant. However, the sequences of NP, F and L genes were identical to those of the wild-type virus (data not shown).
Biological tests were performed to determine whether the E347K mutation affected the biological function of NDV, i.e., HAd, NA, and HA elution, and the results were compared with those of the wild-type virus. The percentage biological (HAd and NA) activity of the E347K mutant virus was determined relative to that of the wild-type virus, which was considered to be 100% (Table 3). The HAd activity of the E347K mutant was 118% of the wild-type virus. The NA activity of the E347K mutant was 166% of the wild-type virus. The E347K mutant was eluted rapidly from chicken red blood cells, whereas the wild-type virus had a slow elution pattern. The pathotype of the wild-type and E347K mutant virus was determined by the MDT and ICPI tests (23). MDT values of wild-type and E347K mutant viruses were 49.6 h and 58.4 h, respectively. In other words, the E347K mutant virus took an average of 8.8 h longer than the wild-type virus did when the viruses kill chicken embryos (Table 2). The ICPI value in day-old chickens was 1.88 for wild-type virus, and 1.57 for E347K mutant virus. These results indicate that these viruses are classified as velogenic virus (≤60 hr for MDT and ≥1.5 for ICPI), according to the classification described by Alexander (23). When E347K mutant re-isolates from dead birds in the ICPI tests were sequenced for HN protein gene, no genetic variation on HN protein gene was found between challenge virus and its re-isolates.
The growth curve kinetics of extracellular E347K mutant and wild-type viruses were analyzed using CEF cells. The supernatant was collected from infected cells at the indicated time-points and virus titers were determined using CEF cells in 96-well plates (Fig. 2). Cells infected with each virus displayed accelerated virus release up to 12 h pi, before reaching their peak titer. However, the peak titer released from infected cells by the E347K mutant virus was approximately 80-times higher (108.2 TCID50/ml) than the wild-type virus (106.3 TCID50/ml). The E347K mutant virus released from infected cells showed a dramatic increase in the NA activity level from 6 h to 12 h pi. The wild-type extracellular virus had a relatively low and gradual increase in the NA activity level compared with the E347K mutant virus.
In this study, we investigated whether an E347K mutation in the HN protein affected the biological function of NDV. The biological effects of the E347K mutation were assessed using a mutant virus that escaped from mAb 10F11 neutralization. The mutant possessed an E347K mutation in the HN protein but no mutations in the F protein, indicating that the residue E347 in a linear epitope of the HN protein, known as an antigenic site 1/14, might be an important determinant for a neutralizing epitope recognized by the mAb 10F11.
The mAb 10F11 also blocked the HA activity of the wild-type virus but not that of the mutant virus, indicating that antigenic site 1/14 (especially E347) might have a role in receptor binding to chicken red blood cells. According to computational conformation modeling analysis, the amino acid residue at 347 forms part of a linear epitope (antigenic sites 1/14) stretching from residues 345 to 352, which is topographically localized in the fourth alpha-helical domain of the globular head region of HN (4, 22). Antigenic sites 1/14 are remote from the hydrophobic sialic acid-binding pocket of HN protein (4). The E347K mutation resulted in a reversal of the residual charge from negative to positive at residue 347, which may have affected the biological function or conformational structure. It is interesting that the main CPE produced by the mutant was cell rounding. The mutant produced few syncytia in infected cells compared with the wild-type virus. This suggests that the E347K mutation in the HN protein may have impaired its ability to promote syncytial formation. This is not surprising because the abolishment of syncytial formation due to a single mutation is reported in several other amino acid residues in the stalk and globular head regions of the HN protein (3, 25, 26). Nevertheless, the reduced fusogenic effect of an E347K mutation in the HN protein has never been reported.
Paramyxovirus-induced syncytia are formed by the membrane fusion of infected cells and neighboring cells via HN and F proteins expressed on the surface of infected cells, although the relationship between HN-F interaction and syncytial formation is still poorly understood (26~30). Thus, the loss of syncytium formation of the mutant virus might be a result of decreased fusion activity (important for cell fusion) or increased neuraminidase activity (involved in progeny virus release from budded infected cells). The structure of the F protein in the E347K mutant virus was not likely to have been affected because no amino acid mutations were found in the F protein of the E347K mutant virus.
Mutation in some residues on HN protein such as residues 123 and 526 is known to affect the pathotype of NDV in chickens (31, 32), although F protein cleavage site has been considered to be a primary determinant of virulence (6, 33). The E347K mutant virus of this study showed a slight decrease in virulence compared to the wild-type virus, but with no change in viral pathotype. This suggests that the E347K mutation in HN protein may have little influence in determining viral pathotype.
Interestingly, the growth curve kinetics (Fig. 2) of the E347K mutant virus showed that it produced an 80-times greater yield of extracellular virus in CEF cells compared with the wild-type virus (Fig. 2). The NA activity was markedly increased in the E347K mutant-infected cells from 6 h to 12 h pi, which was consistent with the pattern of virus titer release from infected cells. The E347K mutant virus had a higher NA activity and a more rapid elution pattern than the wild-type virus (Table 2), indicating that the E347K mutant virus may be released more efficiently from infected cells by neuraminidase than the wild-type. Here we could not rule out the possibility of mutations in other viral genes that might result in an alteration of polymerase complex itself during virus passages in CEF cells and in higher yield of released virus in CEF cells (34). However, no genetic mutation was observed in the NP and L genes of the E347K mutant, which are involved in the formation of polymerase complex during virus replication (34). This indicates that the E347K mutation in the HN protein by mAb 10F11 might not be affected by alteration of the NP and L proteins. Collectively, these observations support the hypothesis concerning the role of the E347K mutation in infected cells: the E347K mutation may increase NA activity, which releases progeny virus particles from infected cells more efficiently, before the formation of syncytia, ultimately resulting in a higher yield of extra-cellular virus.
Figures and Tables
Figure 1
Cytopathic effects induced by wild-type and E347K mutant viruses at 48 dpi in CEF cells. (A) wild type Kr005-infected cells; (B) E347K mutant-infected cells; (C) mock-infected control. E347K mutant virus was generated after 10 passages of wild-type NDV Kr005 strain in the primary CEF cells in the presence of mAb 10F11. Arrow represents cytopathic effects. E347K mutant-infected cells were rounded and granulated with few small-sized few syncytia while the wild type Kr005- infected cells developed large-sized syncytia in primary CEF cells.
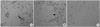
Figure 2
Growth curve kinetics (A) and time-course neuraminidase activity (B) of extracellular viruses released from CEF cells infected with E347K mutant or wild-type viruses. Wild-type and E347K mutant viruses were generated after 10 passages of NDV Kr005 strain in the primary CEF cells in the absence or presence of mAb 10F11, respectively. The E347K mutant virus showed higher growth and enhanced NA activity in primary CEF cells compared to the wild-type virus.
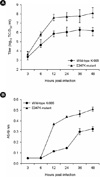
References
1. De leeuw O, Peeters B. Complete nucleotide sequence of Newcastle disease virus: evidence for the existence of a new genus within the subfamily Paramyxovirinae. J Gen Virol. 1999. 80:131–136.


2. Mayo MA. A summary of taxonomic changes recently approved by ICTV. Arch Virol. 2002. 147:1655–1663.


3. Connaris H, Takimoto T, Russell R, Crennell S, Moustafa I, Portner A, et al. Probing the sialic acid binding site of the hemagglutinin-neuraminidase of Newcastle disease virus: identification of key amino acids involved in cell binding, catalysis, and fusion. J Virol. 2002. 76:1816–1824.


4. Crennell S, Takimoto T, Portner A, Taylor G. Crystal structure of the multifunctional paramyxovirus hemagglutinin-neuraminidase. Nat Struct Biol. 2000. 7:1068–1074.
5. Palermo LM, Porotto M, Greengard O, Moscona A. Fusion promotion by a paramyxovirus hemagglutinin-neuraminidase protein: pH modulation of receptor avidity of binding sites I and II. J Virol. 2007. 81:9152.


6. Panda A, Huang Z, Elankumaran S, Rockemann DD, Samal SK. Role of fusion protein cleavage site in the virulence of Newcastle disease virus. Microb Pathog. 2004. 36:1–10.


7. Ke GM, Yu SW, Ho CH, Chu PY, Ke LY, Lin KH, et al. Characterization of newly emerging Newcastle disease viruses isolated during 2002-2008 in Taiwan. Virus Res. 2010. 147:247–257.


8. Iorio RM, Syddall RJ, Sheehan JP, Bratt MA, Glickman RL, Riel AM. Neutralization map of the hemagglutinin-neuraminidase glycoprotein of Newcastle disease virus: domains recognized by monoclonal antibodies that prevent receptor recognition. J Virol. 1991. 65:4999–5006.


9. Cho SH, Kwon HJ, Kim TE, Kim JH, Yoo HS, Kim SJ. Variation of a Newcastle disease virus hemagglutinin-neuraminidase linear epitope. J Clin Microbiol. 2008. 46:1541–1544.


10. Gu M, Liu W, Xu L, Cao Y, Yao C, Hu S, et al. Positive selection in the hemagglutinin-neuraminidase gene of Newcastle disease virus and its effect on vaccine efficacy. Virol J. 2011. 8:150.


11. Hu S, Wang T, Liu Y, Meng C, Wang X, Wu Y, et al. Identification of a variable epitope on the Newcastle disease virus hemagglutinin-neuraminidase protein. Vet Microbiol. 2010. 140:92–97.


12. Huang Y, Wan HQ, Liu HQ, Wu YT, Liu XF. Genomic sequence of an isolate of Newcastle disease virus isolated from an outbreak in geese: a novel six nucleotide insertion in the non-coding region of the nucleoprotein gene. Brief Report. Arch Virol. 2004. 149:1445–1457.
13. Liang R, Cao DJ, Li JQ, Chen J, Guo X, Zhuang FF, et al. Newcastle disease outbreaks in western China were caused by the genotypes VIIa and VIII. Vet Microbiol. 2002. 87:193–203.


14. Lin MY, Liu HJ, Ke GM. Genetic and antigenic analysis of Newcastle disease viruses from recent outbreaks in Taiwan. Avian Pathol. 2003. 32:345–350.


15. Miller PJ, King DJ, Afonso CL, Suarez DL. Antigenic differences among Newcastle disease virus strains of different genotypes used in vaccine formulation affect viral shedding after a virulent challenge. Vaccine. 2007. 25:7238–7246.


16. Tsai HJ, Chang KH, Tseng CH, Frost KM, Manvell RJ, Alexander DJ. Antigenic and genotypical characterization of Newcastle disease viruses isolated in Taiwan between 1969 and 1996. Vet Microbiol. 2004. 104:19–30.


17. Yu L, Wang Z, Jiang Y, Chang L, Kwang J. Characterization of newly emerging Newcastle disease virus isolates from the People's Republic of China and Taiwan. J Clin Microbiol. 2001. 39:3512–3519.


18. Lee YJ, Sung HW, Choi JG, Kim JH, Song CS. Molecular epidemiology of Newcastle disease viruses isolated in South Korea using sequencing of the fusion protein cleavage site region and phylogenetic relationships. Avian Pathol. 2004. 33:482–491.


19. Lee YJ, Sung HW, Choi JG, Lee EK, Yoon H, Kim JH, et al. Protection of chickens from Newcastle disease with a recombinant baculovirus subunit vaccine expressing the fusion and hemagglutinin neuraminidase proteins. J Vet Sci. 2008. 9:301–308.


20. Choi KS, Nah JJ, Ko YJ, Choi CU, Kim JH, Kang SY, et al. Characterization of antigenic sites on the rinderpest virus N protein using monoclonal antibodies. J Vet Sci. 2003. 4:57–65.


21. Tsunekun R, Ito H, Kida H, Otsuki K, Ito T. Increase in the neuraminidase activity of a nonpathogenic Newcastle disease virus isolate during passaging in chickens. J Vet Med Sci. 2010. 72:453–457.


22. Bousse TL, Taylor G, Krishnamurthy S, Portner A, Samal SK, Takimoto T. Biological significance of the second receptor binding site of Newcastle disease virus hemagglutinin-neuraminidase protein. J Virol. 2004. 78:13351–13355.


23. Alexander DJ. Purchase HG, Arp LH, Domermuth CH, Pearson JE, editors. Newcastle disease. A laboratory manual for the isolation and identification of avian pathogens. 1990. 3rd ed. American Association for Avian Pathologists;114–120.
24. Reed LH, Muench H. A simple method of estimating fifty percent endpoints. Am J Hyg. 1938. 27:493–497.
25. Iorio RM, Glickman RL. Fusion Mutants of Newcastle Disease Virus Selected with Monoclonal Antibodies to the Hemagglutinin-Neuraminidase. J Virol. 1992. 66:6626–6633.


26. Takimoto T, Taylor GL, Connaris HC, Crennell SJ, Portner A. Role of the hemagglutinin-neuraminidase protein in the mechanism of paramyxovirus-cell membrane fusion. J Virol. 2002. 76:13028–13033.


27. Gravel KA, Morrison TG. Interacting Domains of the HN and F Proteins of Newcastle Disease Virus. J Virol. 2003. 77:11040–11049.


28. Heminway BR, Yu Y, Galinski MS. Paramyxovirus mediated cell fusion requires co-expression of both the fusion and hemagglutinin-neuraminidase glycoproteins. Virus Res. 1994. 31:1–16.


29. Horvath CM, Paterson RG, Shaughnessy MA, Wood R, Lamb RA. Biological activity of paramyxovirus fusion proteins: factors influencing formation of syncytia. J Virol. 1992. 66:4564–4569.


30. Melanson VR, Iorio RM. Amino acid substitutions in the F-Specific domain in the stalk of the Newcastle disease virus HN protein modulate fusion and interfere with Its interaction with the F protein. J Virol. 2004. 78:13053–13061.


31. Khattar SK, Yan Y, Panda A, Collins PL, Samal SK. A Y526Q mutation in the Newcastle disease virus HN protein reduces its functional activities and attenuates virus replication and pathogenicity. J Virol. 2009. 83:7779–7782.


32. Römer-Oberdörfer A, Veits J, Werner O, Mettenleiter TC. Enhancement of pathogenicity of Newcastle disease virus by alteration of specific amino acid residues in the surface glycoproteins F and HN. Avian Dis. 2006. 50:259–263.

