Abstract
The purpose of this study was to examine profile of bacteria contaminated in a dry sauna. Bacteria sampled from the floor of the dry sauna (75~80℃) were separated and identified by using a thermal gradient gel electrophoresis (TGGE) technique, and were cultivated using a defined medium at 50℃. Bacteria grown in the defined medium were identified based on 16S-rDNA sequence homology. The band number of DNA separated by TGGE was 15, indicating the species diversity of bacteria contaminating the dry sauna. Seven species of bacteria were successfully cultured on agar plate medium at 50℃, which represented a combination of thermophilic and thermoduric bacteria contaminating the dry sauna. The highest limit temperature for growth of the bacterial isolates was generally 50℃ when cultivated in a defined medium, but was raised to 60℃ when cultivated in a complex medium. Consequently, the bacteria grown at 50~60℃ are thermoduric or thermophilic, but others may not be.
Thermoduric bacteria are microorganisms capable of surviving the heating processes for pasteurization but can't grow to regenerate under thermal conditions. Some thermoduric bacteria can contaminate processed food, creating health hazards by means of their growth after pasteurizing processes (1, 2). The thermophilic bacteria not only can survive heating treatment but also generally grow under the thermal condition from 45 to 122℃ (3, 4), differentiating them from thermoduric bacteria (5~7). Thermophilic bacteria are rarely found as growing cells at mammalian temperature (8).
Hence, few manmade environments are suitable for the growth of thermophilic bacteria, but many may be contaminated by thermoduric bacteria. Dry saunas are maintained at elevated temperature, and due to the traffic of users, they are exposed to various microorganisms, which may be periodically removed by cleaning and disinfection (9). However, endospore-forming microorganisms may survive attacks by detergent, hot air, and disinfectants, considering that endospores are often highly resistant to chemical- and heat-treatment (10). Accordingly, endospore-forming bacteria may be thermoduric but are usually not thermophiles because their vegetative cells can't grow under thermal condition for thermophilic bacteria but endospore can survive thermal condition. Bacterial species belonging to the Bacillus genus are typical endospore-forming micro-organisms, and are generally found in natural and artificial environments (11~13). The Bacillus genus is widely distributed in the natural environment, such as in soil and water, and ubiquitously contaminates various foods such as fruit and vegetable products, nuts, cereals, rice, dried foods, spices, and dairy products. Hence, Bacillus is diffused to anywhere people migrate. Most bacteria living around humans may be spread through foods or through direct contact of the human body (14, 15).
In this study, the bacterial community randomly sampled from a dry sauna was analyzed using a thermal gradient gel electrophoresis technique (TGGE), and was also cultivated at 60℃, based on the temperature of pasteurization to verify thermophilic bacteria. The bacterial species detected in TGGE were compared with those grown at 60℃ in order to determine the relative number of species of thermophilic bacteria within the bacterial community.
A digital thermometer on the wall of the dry sauna indicated that the internal temperature was a constant 75~80℃. Bacteria were sampled from floor of the dry sauna using a sterilized cotton swab. Samples were collected at 4 o'clock in the morning when there were no users present. An aluminum foil wrapped cotton swab was opened immediately before sampling, and the floor was then deeply and broadly wiped with 6 cotton swabs per sample area. The sampling points were located at a 30 cm distance from each wall or wood bench and the diameter of sampling area was about 30 cm as shown in Fig. 1A. Of the 6 swabs taken, one was used for bacterial cultivation and 5 were used for DNA extraction. The part of the cotton swab used for bacterial sampling was put in a sterilized conical tube immediately after sampling, while the other end was removed as shown in Fig. 1B.
Samples were inoculated on a defined agar plate medium composed of 1 g yeast extract, 20 g agar, and 1 L tap water, and were cultivated at 50℃ for 72 h. All bacterial colonies were separately isolated and were cultivated in 50℃ in the defined broth medium (1 g yeast extract and 1 L tap water). The 16S-rDNA was amplified with genomic DNA extracted from the cells grown in the broth medium and were sequenced for identification based on GenBank database (http://www.ncbi.nlm.nih.gov). The grown bacteria were cultivated in the complex broth medium at 20, 30, 40, 50, 60, and 70℃ in order to determine the optimal growth temperature. Complex medium was composed of 3 g yeast extract, 3 g glucose, 3 g sodium lactate, 1.5 g K2HPO4, 0.01g CaCl2, 0.001 g MgSO4, 0.001 g MnSO4, and 1 L tap water. Bacterial growth was determined by optical density (OD) at 660 nm.
Total DNA was directly extracted from the bacterial cells using a Genomic DNA Extraction Kit (Accuprep, Bioneer, Korea) according to the manufacturer's protocol. 16S ribosomal DNA was amplified via direct PCR using the chromosomal DNA template and 16S-rDNA specific universal primers as follows: forward 5'-GAGTTGGATCCTGGCTCAG-3' and reverse 5'-AAGGAGGGGATCCAGCC-3'. The PCR reaction mixture (50 µl) consisted of 2.5 U Tag polymerase, 250 µM each of dNTP, 10 mM Tris-HCl (pH 9.0), 40 mM KCl, 100 ng template, 50 pM primer, and 1.5 mM MgCl2. Amplification was conducted for 30 cycles of 1 min at 95℃, 1 min of annealing at 55℃, and 2 min of extension at 72℃ using a PCR machine (T Gradient model, Biometra, German).
The 16S-rDNA amplified from chromosomal DNA was employed as a template for TGGE sample preparation. A variable region of 16S-rDNA was amplified with forward primer (eubacteria, V3 region) 341f 5'-CCTACGGGAGGCAGCAG-3' and reverse primer (universal, V3 region) 518r 5'-ATTACCGCGGCTGCTGG-3'. A GC clamp (5'-CGCCCGCCGCGCGCGGCGGGCGGGGCGGGGGCACGGGGGGCCTACGGGAGGCAGCAG-3') was attached to the 5'-end of the GC341f primer (16). The procedures for PCR and DNA sequencing were the same as 16S-rDNA amplification conditions, except for an additional annealing temperature of 53℃. The TGGE system (Bio-Rad, Dcode™, Universal Mutation Detection System, USA) was operated in accordance with the manufacturer's instructions. Aliquots (45 µl) of the PCR products were electrophoresed in gels containing 8% acrylamide, 8 M urea, and 20% formamide with a 1.5 x TAE buffer (7.26 g/l Trisma, 1.815 g/l Acetatic acid, and 0.56 g/l Ethylenediaminetetraacetate) buffer system at a constant voltage of 100 V for 12.5 hr and then 40 V for 0.5 hr, applying a thermal gradient of 39 to 52℃. Prior to electrophoresis, the gels were equilibrated to the temperature gradient for 30 to 45 min.
DNA was extracted from the TGGE band and was purified with a DNA gel purification kit (Accuprep). The purified DNA was then amplified with the same primers and procedures used for TGGE sample preparation, except that the GC clamp was not attached to the forward primer. The amplified DNA was sequenced for identification of bacteria based on 16S-rDNA sequence homology.
TGGE patterns of bacterial community sampled from 4 points of floor in the dry sauna were a little different from each other, as shown in Fig. 2. The number of bacterial species contaminating the dry sauna, as identified by TGGE technique, was approximately 15, based on the difference of electrophoretic migration distance of the DNA. The bacterial community sampled from corners 1 and 4 were very similar each other, but differed significantly from those in corners 2 and 3. The diversity of bacterial species may be limited depending on the thermal and drying conditions within a specific area, and the bacterial community in dry sauna so frequently contaminated by users may be opportunistically changed.
All bacterial species separated by the TGGE technique and identified by the sequence homology were found in all sample locations, except for Virgibacillus sp., Tepidomonas sp., and Janthinobacterium sp., while, bacterial species belonging to the Bacillus genus were commonly detected in sampling point 1, 2, and 4 but not in sampling point 3, as shown in Table 1. Bacterial contamination of any location within the sauna may be randomly and opportunistically varied according to the bacterial contamination of individual users.
The general character of bacterial species sampled from the floor of the dry sauna was tabulated based on information of genus or species reported by other researchers. As shown in Table 2, the growth temperature of Bacillus licheniformis, Tepidomonas sp., and Pseudoxanthomonas taiwanensis is higher than 50℃, while Bacillus sp. and Virgibacillus sp. are endospore-forming bacteria. On the other hand, Janthinobacterium sp. and Stenotrophomonas sp. are not the endospore-forming ones and the thermophiles. Accordingly, Janthinobacterium sp. and Stenotrophomonas sp. are likely to simply contaminate the dry sauna through users without vitality. However, other species may maintain their survival by sporulation or physiological function, for they are thermally resistance (17~26).
Bacterial colonies were cultivated on defined agar plate medium at 50℃, and were then isolated and identified. The isolates were cultivated in the defined medium and complex medium at different temperatures from 20 to 70℃, as shown in Tables 3 and 4. The endospore-forming or thermophilic bacteria grew from 30 to 50℃.
Temperature range and optimal temperature for growth of endospore-forming or thermophilic bacteria was 30~50℃ and 40℃, respectively in the defined medium; however, the isolates grew at temperatures ranging from 30 to 60℃, and maximally grew at 40 and 50℃ in the complex medium. The endospore-forming bacteria Bacillus sp. and Virgibacillus sp. were detected by TGGE and commonly grew at 60℃ in the complex medium, while the non-endospore-forming thermophilic bacteria Tepidomonas sp., and Pseudoxanthomonas taiwanensis were also detected by TGGE and grew at 60℃ in the complex medium. However, Stenotrophomonas sp. and Janthinobacterium sp. were detected by TGGE but did not grow at 50℃, even in the complex medium. This result is a clue that Stenotrophomonas sp. and Janthinobacterium sp. are non-endospore-forming and non-thermophilic bacteria but may be simple contaminants without vitality.
Dry saunas are a popular convenient facility which is routinely used for health care and relaxation in many countries. The temperature of a dry sauna is between 70~80℃ but is not a cause to scald users because the hot and dry air induces physiologically sweating but is not enough to increase temperature of human body. The bacterial cells contaminating users' bodies may not be directly exposed to hot and dry air which is enough for sterilization of non-endospore-forming and non-thermophilic bacteria, but some of them may be separated from the users' body through sweating or through direct contact with the sauna. The non-endospore-forming and non-thermophilic bacteria exposed to hot and dry conditions may be physiologically deactivated. Both endospore-forming bacteria (thermoduric bacteria) and thermophilic bacteria may survive on the sauna floor because endospores are strongly resistant against heating and drying, and thermophilic bacteria may maintain their metabolic function with nutrients contained in the users' sweat (27~29). Hence, both endospore-forming bacteria and thermophilic bacteria were cultured at 50~60℃ and were detected by TGGE, however, non-endospore-forming and non-thermophilic bacteria were also detected by TGGE, but not grown at 50~60℃ in complex medium.
DNA bands separated by TGGE technique were totally 15 but diversity of bacterial species was 9, which may be significantly lower than that inhabiting in natural environment. The relatively low diversity of bacterial species in the sauna may be caused by limitation in the process for bacterial sampling from the sauna and DNA extraction from bacterial samples. On the other hand, the defined medium used for bacterial isolation from the sauna may be a cause to limit bacterial diversity. Practically, cause of the limitations may be solved by improvement of sampling method, DNA extraction process, and bacterial cultivation condition. We have plan to precisely analyze diversity of bacteria contaminated in the sauna by increase of sampling frequency, application of upgraded DNA extraction system, and employment of various culture media.
The physiological function for heat resistance of thermophilic bacteria may depend upon the nutritional conditions present at higher than optimal temperature. The relatively rich nutrients of complex medium can, in some cases, allow bacterial cells to generate the physiological energy required to cope with disadvantageous conditions, such as those at 60℃ for mesophilic bacteria. Endospore-forming bacteria and thermophilic bacteria commonly grew in complex medium at 60℃, which is evidence that nutrients contained in user's sweat are used as a nutritional source for bacterial growth and sporulation within the dry sauna (30).
The dry sauna provides for the growth of thermophilic bacteria and the sporulation of endospore-forming bacteria, and also for contamination of both thermophilic and thermoduric bacteria by contact with various users. Bacterial contamination opportunities within a sauna are likely dependent upon the cleaning frequency. Daily cleaning in 24 h-operating sauna is also not an effective way to decrease contamination, considering that users may cross contaminate the sauna while cleaning is taking place. Various bacteria contaminating the human body, including those which are capable of causing opportunistic food poisoning, or human pathogens, may be continuously contaminating dry saunas in proportion to user numbers (31); however, most of bacteria contaminating the dry sauna can't be a cause for food poisoning or infection disease because all of users wash their body after using the sauna.
Conclusively, dry saunas may be an artificial environment for the opportunistic growth of various thermophilic or endospore-forming bacteria. Culture systems which are suitable for thermophilic bacteria are rarely contaminated by mesophilic bacteria which are generally distributed in the temperate region.
Figures and Tables
![]() | Figure 1Location of sampling area in floor of dry sauna (A) and sampling method of bacteria contaminated in floor of dry sauna (B). |
![]() | Figure 2Thermal gradient gel electrophoresis (TGGE) profiles of bacterial communities isolated from floor in 4 corners of sauna. The left figure is a real picture and the right figure is a black-and white picture that was duplicated from the real picture to mark band number. |
Table 1
Bacterial species identified based on sequence homology of 16S-rDNA that was separated by thermal gradient gel electrophoresis (TGGE) of DNA extracted from the bacterial community sampled from a sauna.
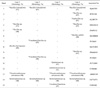
Table 2
General character of bacterial genus or species identified based on sequence homology of DNA extracted from thermal gradient gel electrophoresis (TGGE) gel.
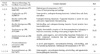
References
1. Walsh C, Meade J, McGill K, Fanning S. The biodiversity of thermoduric bacteria isolated from whey. J Food Saf. 2012. 32:255–261.


2. Banykó J, Vyletelová M. Determining the source of Bacillus cereus and Bacillus licheniformis isolated from raw milk, pasteurised milk and yoghurt. Lett Appl Microbiol. 2009. 48:318–323.


3. Bott TL, Brock TD. Bacterial growth rates above 90 degrees C in Yellowstone hot springs. Science. 1969. 164:1411–1412.


4. Brock TD, Freeze H. Thermus aquaticus gen. n. and sp. n., a nonsporulating extreme thermophile. J Bacteriol. 1969. 98:289–297.


5. Brock TD, Boylen LK. Presence of thermophilic bacteria in laundry and domestic hot-water heaters. Appl Microbiol. 1973. 25:72–76.


6. Pask-Hughes R, Williama RA. Extremely thermophilic gram-negative bacteria from hot tap water. J Gen Microbiol. 1975. 88:321–328.


7. Oshima T, Imahori K. Description of Thermus thermophiles (Yoshida and Oshima) comb. Nov. A non-sporulating thermophilic bacterium from a Japanese thermal spa. Intern System Bacteriol. 1974. 24:102–112.


8. Ward J, Cockson A. Studies on a thermophilic bacillus: its isolation, properties, and temperature coefficient of growth. J Bacteriol. 1972. 112:1040–1042.


9. Metzger WJ, Patterson R, Fink J, Semerdjian R, Roberts M. Sauna-takers disease. Hypersensitivity pneumonitis due to contaminated water in a home sauna. JAMA. 1976. 236:2209–2211.


10. Scheldeman P, Pil A, Herman L, De Vos P, Heyndrickx M. Incidence and diversity of potentially highly heat-resistant spores isolated at dairy farms. Appl Environ Microbiol. 2005. 71:1480–1494.


11. Finley N, Fields ML. Heat activation and heat-induced dormancy of Bacillus stearothermophilus spores. Appl Microbiol. 1962. 10:231–236.


12. Hill WM, Fields ML. Factors affecting growth and interaction of rough and smooth variants of Bacillus stearothermophilus. I. Oxygen tension and temperature. J Food Sci. 1967. 32:458–462.


13. O'Brien RT, Campbell LL Jr. The nutritional requirements for germination and outgrowth of spores and vegetative cell growth of some aerobic spore forming bacteria. J Bacteriol. 1957. 73:522–525.
14. Martin PA, Travers RS. Worldwide abundance and distribution of Bacillus thuringiensis isolates. Appl Environ Microbiol. 1989. 55:2437–2442.


15. Stefanic P, Mandic-Mulec I. Social interactions and distribution of Bacillus subtilis pherotypes at microscale. J Bacteriol. 2009. 191:1756–1764.


16. Lee SJ, Lee YW, Chung J, Lee JK, Lee JY, Jahng D, et al. Reuse of low concentrated electronic wastewater using selected microbe immobilised cell system. Water Sci Technol. 2008. 57:1191–1197.


17. Salkinoja-Salonen MS, Vuorio R, Andersson MA, Kämpfer P, Andersson MC, Honkanen-Buzalski T, et al. Toxigenic Strains of Bacillus licheniformis Related to Food Poisoning. Appl Environ Microbiol. 1999. 65:4637–4645.


18. An SY, Asahara M, Goto K, Kasai H, Yokota A. Virgibacillus halophilus sp. nov., spore-forming bacterial isolated from soil in Japan. Int J Syst Evol Microbiol. 2007. 57:1607–1611.


19. Earl AM, Losick R, Kolter R. Ecology and genomics of Bacillus subtilis. Trends Microbiol. 2008. 16:269–275.
20. Dean DH. Biochemical genetics of the bacterial insectcontrol agent Bacillus thuringiensis: basic principles and prospects for genetic engineering. Biotechnol Genet Eng Rev. 1984. 2:341–363.


21. Moreira C, Rainey FA, Nobre MF, da Silva MT, da Costa MS. Tepidimonas ignava gen nov, sp nov, a new chemolithoheterotrophic and slightly thermophilic member of the beta-Proteobacteria. Int J Syst Evol Microbiol. 2000. 50:735–742.


22. Mountain BW, Benning LG, Boerema JA. Experimental studies on New Zealand hot spring sinters: rates of growth and textural development. Can J Earth Sci. 2003. 40:1643–1667.


23. Kämpfer P, Falsen E, Busse HJ. Reclassification of Pseudomonas mephitica claydon and hammer 1939 as a later heterotypic synonym of Janthinobacterium lividum (Eisenberg 1891) De Ley et al. 1978. Int J Syst Evol Microbiol. 2008. 58:136–138.
24. Stead DE. Grouping of plant-pathogenic and some other Pseudomonas spp. by using cellular fatty acid profiles. IJSEM. 1992. 42:281–295.


25. Chen MY, Tsay SS, Chen KY, Shi YC, Lin YT, Lin GH. Pseudoxanthomonas taiwanensis sp. nov., a novel thermophilic, N2O-producing species isolated from hot springs. Int J Syst Evol Microbiol. 2002. 52:2155–2161.


26. Palleroni NJ, Bradbury JF. Stenotrophomonas, a new bacterial genus for Xanthomonas maltophilia (Hugh 1980) Swings et al. 1983. Int J Syst Bacteriol. 1993. 43:606–609.


27. Yokoya F, York GK. Effect of several environmental conditions on the "thermal death rate" of endospores of aerobic, thermophilic bacteria. Appl Microbiol. 1965. 13:993–999.


28. Nicholson WL, Munakata N, Horneck G, Melosh HJ, Setlow P. Resistance of Bacillus endospores to extreme terrestrial and extraterrestrial environments. Microbiol Mol Biol Rev. 2000. 64:548–572.


29. Lowe SE, Jain MK, Zeikus JG. Biology, ecology, and biotechnological applications of anaerobic bacteria adapted to environmental stresses in temperature, pH, salinity, or substrates. Microbiol Rev. 1993. 57:451–509.

