Abstract
Vibrio vulnificus, a gram-negative halophilic marine bacterium and opportunistic human pathogen, must withstand various environmental changes, especially simultaneous changes in temperature and salinity, from 25℃/2.5% to 37℃/0.9% (SCTS) upon entering the human body. In our previous study, SCTS stimulated vvpE expression even in the background of a mutation in luxS encoding LuxS enzyme for the biosynthesis of quorum-sensing (QS) signal molecule autoinducer-2 (AI-2), suggesting that the A1-2-mediated QS system is partially involved in the SCTS-mediated change of vvpE expression. In this study, we examined the effects of the QS master regulator SmcR on SCTS-mediated changes in vvpE expression and extracellular VvpE production. SCTS stimulated V. vulnificus growth, but with no increase in maximal growth levels. The SCTS-mediated prolongation of the stationary growth phase resulted in a significant increase in growth phase-dependent smcR and vvpE expressions. A mutation in smcR seriously repressed vvpE expression, but had no significant effect on V. vulnificus growth. However, the smcR mutation only partially attenuates SCTS-mediated changes in vvpE expression. These results indicate that SCTS stimulates the expressions of smcR and vvpE by stimulating V. vulnificus growth, and that SmcR is only partially involved in SCTS-mediated changes in vvpE expression.
Vibrio vulnificus, a gram-negative halophilic marine bacterium, is an opportunistic pathogen that causes rapidly progressing fatal septicemia and necrotizing wound infections, preferentially in susceptible patients with hepatic diseases, hemochromatosis, heavy alcohol drinking habits, and other immunocompromised conditions (1).
V. vulnificus produces a metalloprotease known as VvpE. Purified VvpE exhibits a variety of biological activities including hemorrhagic damage, dermonecrosis, enhanced vascular permeability, and edema, and is lethal to mice (1). Although the pathogenetic role of VvpE remains enigmatic (2), vvpE expression or VvpE production is a useful tool for studying how V. vulnificus senses and responds to diverse environmental signals, since it is the best known phenotype directly controlled by three global regulators, RpoS (the stationary sigma factor responsible for general stress response), SmcR (the master regulator of quorum sensing [QS] systems responsible for bacterial density-dependent gene expression), and Crp (cAMP-receptor protein responsible for catabolite repression) (3, 4). In addition, extracellular VvpE production occurs via the type II general secretion system (GSS), the activity of which is influenced by environmental factors (5~7).
The precise role of QS is still a matter of debate. Nevertheless, QS probably plays a vital role in environmental response and gives each bacterium a survival advantage (8). V. vulnificus possesses two QS systems. First, autoinducer (AI)-2 signaling is finally transferred to the master regulator SmcR (a LuxR homolog) via LuxP, LuxQ, LuxU, LuxO, and LuxT (9). SmcR regulates vvpE expression at the transcription level by directly binding to the vvpE promoter in V. vulnificus (3). Second, another QS signal molecule acylated homoserine lactone (AHL: AI-1) was recently identified in V. vulnificus, although no known AHL-related gene was detected (10). AHL signaling is also likely to be finally transferred to SmcR in V. vulnificus, as in V. harveyi (11). The presence of the two QS systems implies that they can cooperate, and that smcR mutation that blocks both AI-2 signaling and AHL signaling is more suitable for the complete disruption of QS than luxS mutation, which blocks only AI-2 signaling.
V. vulnificus inhabits sea water and can infect the human body, and these two environments differ in many respects. As a result, V. vulnificus must overcome and survive diverse environmental changes to establish successful human infections. Virulence expression is a survival strategy of pathogenic bacteria (12), and V. vulnificus is capable of sensing and responding to diverse environmental changes by modulating the expressions of the virulence factors required for survival (13, 14). Many environmental factors including temperature, salinity, iron, and oxygen levels can affect vvpE expression (15). In particular, a temperature of 25℃ and a salinity of 2.5% mimics estuarine condition in the summer, and 37℃ and 0.9% the human body condition. Previous studies have suggested that V. vulnificus might sense and respond to temperature and salinity changes by modulating vvpE expression via AI-2-QS system (15, 16). In our previous study (17), a simultaneous change of temperature and salinity from 25℃/2.5% to 37℃/0.9% (SCTS) stimulated vvpE expression even in the background of a mutation in luxS encoding LuxS enzyme, which is required for AI-2 biosynthesis (18). These findings suggest that the A1-2-QS system is only partially involved in the SCTS-mediated modulation of vvpE expression. This obscurity regarding the role played by the AI-2-QS system may be because the luxS mutation disrupts only AI-2 signaling, and this may result in an increase in AHL signaling. Accordingly, in an attempt at obtaining unequivocal evidence regarding the involvements of the QS systems, we examined the effect of a smcR mutation that can simultaneously block AI-2 and AHL signaling, on the SCTS-mediated modulation of vvpE expression and extracellular VvpE production under the same condition as in our previous study (17).
The bacterial strains, plasmids, and primers used in this study are listed in Table 1. Heart Infusion (HI; Becton-Dickinson (BD), Franklin Lakes, NJ, USA) broth and agar containing 2.5% sodium chloride were used to cultivate V. vulnificus strains. Luria-Bertani medium (BD) and Thiosulfate-Citrate-Bile-Sucrose medium (BD) with or without appropriate antibiotics were used to select and subculture recombinant strains. Antibiotics (BD) were used at the following concentrations; for Escherichia coli, ampicillin 50 µg/ml, kanamycin 50 µg/ml, tetracycline 12.5 µg/ml, and chloramphenicol 30 µg/ml; for V. vulnificus, ampicillin 20 µg/ml, kanamycin 200 µg/ml, tetracycline 2 µg/ml, and chloramphenicol 2 µg/ml. Unless otherwise stated, all reagents used were purchased from Sigma-Aldrich (St. Louis, MO, USA).
V. vulnificus strains were preconditioned by culturing in HI broth containing 2.5% NaCl at 25℃ overnight to adapt them to conditions mimicking their natural habitat. The preconditioned strains were inoculated into HI broth containing 0.9% NaCl or 2.5% NaCl at 1 × 106 cells/ml and cultured with vigorous shaking (220 rpm) at 37℃ or 25℃ for 24 h. Culture aliquots were withdrawn at appropriate times to measure bacterial growth and β-galactosidase activity. To measure extracellular VvpE production, culture supernatants were obtained from culture aliquots by centrifugation (10,000 × g for 5 min). Bacterial growth was measured by determining the optical densities of culture aliquots at a wavelength of 600 nm (OD600). β-Galactosidase activity in culture aliquots was measured using the Miller method (26).
Rabbit polyclonal anti-VvpE antibody was prepared and VvpE production was measured using Western blotting, as described in our previous study (5). In brief, equal volumes (20 µl) of culture supernatants were electrophoresed on 10% sodium dodecyl sulfate-polyacrylamide gels and then transferred to nitrocellulose membranes. Membranes were incubated with rabbit polyclonal anti-VvpE antibody and subsequently with anti-rabbit-IgG antibody conjugated with alkaline phosphatase. Finally, 5-bromo-4-chloro-3-indolyl-phosphate/ nitro blue tetrazolium solution was used for visualization. The amount of VvpE on Western blot was quantitatively analyzed using a densitometer program (UN-SCAN- IT gel Automatid Digitalizing System ver. 4.1, Silk Scientific Corporation).
V. vulnificus M06-24/O, a highly virulent clinical isolate (19), was used as the wild-type strain. A deletion mutant in smcR was constructed using the suicide vector pDM4 with a R6K origin (21). The 5' and 3' ends of smcR were amplified by polymerase-chain reaction (PCR) using the primers; smcR-up-1/2 and smcR-down-1/2. The resulting PCR products were used as the DNA template for the second crossover PCR using the primers; smcR-up-1 with a SmaI overhang and smcR-down-2 with a SpeI overhang. The resulting SmaI-SpeI fragment with deleted smcR was cloned into pDM4. The resulting plasmid was named pRC152, and transformed into E. coli SY327 λpir and SM10 λpir (20), and subsequently transferred to M06-24/O by conjugation. Finally, a stable transconjugant was selected on TCBS agar containing chloramphenicol and on HI agar containing 10% sucrose, and named RC174. The deletion mutation of smcR was confirmed by PCR using the primers smcR-up-1 and smcR-down-2.
To restore wild-type smcR in RC174 with mutated smcR, the HindIII-EcoRI fragment containing the promoter region and the open reading frame of smcR was amplified using the PCR primers; smcR-comp-1 with a HindIII overhang and smcR-comp-2 with a EcoRI overhang, and was subsequently subcloned into the broad host range vector pLAFR3II (18), which was prepared by subcloning the BamHI-BglII fragment containing the bla gene of pUTKm1 into a BglII site of pLAFR3 (24, 25). The resulting plasmid was named pRC158 and transferred into RC174 by triparental mating using the conjugative helper plasmid pRK2013 (24). Finally, a stable transconjugant was selected on TCBS agar containing ampicillin and tetracycline and named RC186. The presence of wild-type smcR on plasmid DNA was confirmed by PCR using the primers smcR-comp-1 and 2.
V. vulnificus CMM2101 with a lacZ mutation (18), which was originated from M06-24/O, was used as the wild-type strain for the construction of transcriptional reporter strains. V. vulnificus CMM2106 with the PvvpE::lacZ transcription fusion was constructed as described previously (18). V. vulnificus RC164 was constructed by introducing a smcR mutation into V. vulnificus CMM2106 with the PvvpE::lacZ transcription fusion, as described above. The merozygotic PsmcR::lacZ transcription reporter was constructed as follows. A 926-bp BamHI-HindIII fragment containing the promoter region of smcR was amplified using the PCR primers smcR-rep-F with BamHI overhang and smcR-rep-R with HindIII overhang, and the resulting PCR product was subcloned into pQF52 (22). From the resulting plasmid pRC162, the BamHI-ScaI fragment containing the PsmcR::lacZ fragment was isolated and subcloned into pDM4 (21). The resulting plasmid pRC164 was transformed into E. coli SY327 λpir and SM10 λpir (20), and transferred to CMM2101 by conjugation. Eventually, a transconjugant (RC196) was selected on TCBS agar containing chloramphenicol. The presence of the PsmcR::lacZ fusion in RC196 was confirmed by β-galactosidase assay (26).
The effect of SCTS on smcR expression was determined using RC196 with the merozygotic PsmcR::lacZ transcriptional fusion. SCTS stimulated and advanced RC196 growth with no significant difference in the maximal growth level, and subsequently prolonged the duration of the stationary growth phase in 24 h-batch culture (Fig. 1A). The pattern of smcR expression was growth phase- and bacterial density-dependent (Fig. 1B and 1C): smcR expression was initiated at low bacterial densities, gradually increased during the exponential growth phase, and thereafter steeply increased at high bacterial densities or during the stationary growth phase. SCTS significantly increased smcR expression levels on a per-cell basis. Overall, these results indicate that SCTS can increase total QS activity by facilitating smcR expression and V. vulnificus growth.
The effects of SCTS on vvpE expression in the background of wild-type or mutated smcR were determined using the two PvvpE::lacZ transcriptional reporter strains, CMM2106 with wild-type smcR and RC164 with mutated smcR. SCTS stimulated and advanced the growths of the two strains, and prolonged the duration of the stationary growth phase (Fig. 2A and 2D). No significant difference in the growth levels of the two strains was observed, indicating that SmcR does not affect V. vulnificus growth. In CMM2106, the pattern of vvpE expression was growth phase- and bacterial density-dependent (Fig. 2B and 2C): vvpE expression was initiated at low bacterial densities, gradually increased during the exponential growth phase, and thereafter steeply increased at high bacterial densities or during the stationary growth phase. SCTS did not increase vvpE expression levels on a per cell basis during the exponential growth phase. However, SCTS significantly increased vvpE expression levels once the growth of CMM2106 arrived at the stationary growth phase. Accordingly, SCTS increased total vvpE expression levels, and prolonged the duration of the stationary growth phase in 24-h batch culture. In RC164, vvpE expression was severely repressed, and the pattern of vvpE expression was not growth phase-dependent: vvpE expression increased during the exponential growth phase, but not during the stationary growth phase. Nevertheless, SCTS also increased vvpE expression levels in RC164. However, when compared quantitatively, the SCTS-mediated increase in vvpE expression was approximately 4-fold in CMM2106 and approximately 2-fold in RC164. These results indicate that SCTS facilitates growth phase-dependent vvpE expression by stimulating V. vulnificus growth, and that SmcR partially affects SCTS-mediated changes in vvpE expression.
The effects of SCTS on extracellular VvpE production in the background of wild-type smcR, mutated smcR, and in trans complemented smcR were determined using the three strains, M06-24/O with wild-type smcR, RC174 with mutated smcR, and RC186 with in trans complemented smcR. SCTS stimulated and advanced the growth of all three strains, and prolonged the duration of the stationary growth phase (Fig. 3A). The smcR mutation or in trans complementation had no significant effect on V. vulnificus growth. In M06-24/O, SCTS advanced and stimulated extracellular VvpE production, and this was consistent with growth levels (Fig. 3B). SCTS or the smcR mutation did not affect the pattern of extracellular VvpE production: 35 kDa-VvpE was the main form under all conditions. At 25℃/2.5%, extracellular VvpE production was first observed at 10~11 h after culture initiation, and at 37℃/0.9%, extracellular VvpE production was first observed at 4 or 5 h after culture initiation. The smcR mutation in RC174 lowered and delayed extracellular VvpE production, and these changes were recovered by the in trans smcR complementation in RC186. The SCTS-mediated change in extracellular VvpE production appeared to be slightly attenuated but was still observed in RC174 with the smcR mutation: extracellular VvpE production was observed from 12 h at 25℃/2.5% and from 8 h at 37℃/0.9%. When quantitative analyzed using densitometry (Fig. 3C), the SCTS-mediated change in extracellular VvpE production was also still observed in RC174 with the smcR mutation. These results indicate that SCTS facilitates extracellular VvpE production, stimulating V. vulnificus growth, and that SmcR is not the main factor responsible for SCTS-mediated changes in extracellular VvpE production.
The present study shows that SCTS stimulates the expressions of smcR and vvpE by stimulating V. vulnificus growth, and that SmcR is only partially involved in SCTS-mediated changes in vvpE expression.
Virulence expression is a survival strategy of pathogenic bacteria (8). V. vulnificus inhabits sea water and can infect the human body. During the infection process, V. vulnificus must be able to sense and sustain changes in environmental factors including SCTS (13~17). Signals emanating from changes in environmental factors are relayed to specific genes by way of cognate signal transduction systems and result in the expressions of genes associated with specific virulence factors. Our previous (17) and present studies show that SCTS stimulates V. vulnificus growth and facilitates vvpE expression or extracellular VvpE production. These findings suggest that VvpE functions as one of the several virulence factors required for the better survival of V. vulnificus on entering the human body. As there have been serious doubts concerning the pathogenetic role of VvpE (2), a new paradigm is required to elucidate the currently obscure pathogenetic roles of VvpE. Generally, bacteria grow asynchronously, for example, V. vulnificus cells at different phases of growth can exist simultaneously in cultures, and this is invariably the case in vivo. Furthermore, the VvpE produced by V. vulnificus cells that have arrived at the stationary growth phase can affect the growth or survival of V. vulnificus cells beginning to grow, by destroying various host proteins. In our previous study, we found that VvpE can destroy various host proteins, such as lactoferrin and immunoglobulin A and that VvpE is essentially required for V. vulnificus swarming (27). Bacterial swarming is defined as a rapid and coordinated population migration of flagellated bacteria across solid surfaces, and is considered a good model of adherence to, colonization on, and invasiveness into mucosa, which are essential steps during early infection (28). Moreover, the destruction of the iron-withholding protein lactoferrin and immunoglobulin A, both of which are important components for mucosal immunity, by VvpE may affect the growth and survival of V. vulnificus at the early stage of infection (29). Accordingly, VvpE is likely to play significant roles in adherence to, colonization on, and invasiveness into mucosa by V. vulnificus during early infection, although VvpE is mainly produced at the stationary growth phase in vitro.
It is believed that QS plays a vital role in environmental response, and that it provides a survival advantage, although its precise role is still a matter of debate (8). A mutation in luxS or smcR lowers virulence expression in experimental mouse models (18, 30), and QS actually operates in a human ex vivo experimental system (submitted). In our previous study (17), SCTS stimulated and advanced V. vulnificus growth with no increase in the maximal growth level, and the consequent prolongation of the stationary growth phase significantly increased growth phase-dependent luxS expression. In the present study, SCTS significantly increased smcR expression. These findings indicate that the activity of QS systems is higher at 37℃/0.9% than at 25℃/2.5%. The expression level of luxS alone cannot represent actual AI-2-QSS activity or smcR expression level because AI-2 (furanosyl borate diester) production is mediated by several spontaneous non-enzymatic reactions in addition to the enzymatic action of LuxS (31). Moreover, another QS signal molecule AHL may also affect smcR expression (10). AI-2 signaling is almost completely transferred to SmcR, and AHL signaling is considered to be finally transferred to SmcR in V. vulnificus, and the two QS systems can cooperate to improve survival in V. vulnificus, as in V. harveyi (11). Accordingly, the expression level of smcR is likely to directly represent the total or final activity of the QS systems. The smcR mutation adopted in this study is more suitable for the complete disruption of QS than the luxS mutation adopted in our previous study (17). In addition, QS activity should be evaluated with vvpE expression, because vvpE expression is the best known target directly regulated by SmcR. In this study, the smcR mutation seriously repressed vvpE expression, which reconfirms that SmcR, the master regulator of QS systems, regulates vvpE expression.
Temperature-dependent expression of virulence genes has been extensively studied in Shigella species (32, 33). Shigella infection is spread via the fecal-oral route, and is commonly acquired by drinking water contaminated with human fecal material. Virulence genes in Shigella species, including ipa (invasion plasmid antigen), spa (surface presentation antigens) and mxi (membrane expression of invasion) operons, are induced at 37℃ and repressed at 30℃. Changes in DNA supercoiling and the transcription repressor H-NS (VirR) are likely to play important roles in the temperature-dependent regulation of virulence genes. VirF positively regulates virB transcription, and VirB acts as a positive regulator for the transcriptions of the ipa, mxi, and spa operons. An increase in temperature activates the VirF-dependent virB promoter by changing DNA supercoiling. In addition, virB and virF transcriptions are repressed by H-NS (VirR) at a low temperature, but derepressed at a permissive temperature (i.e., 37℃). Although there is no experimental evidence, similar mechanisms may also be involved in the temperature-dependent expressions of smcR and vvpE in V. vulnificus.
In our previous study (17), the SCTS-mediated modulations of vvpE expression and extracellular VvpE production were slight attenuated in the background of luxS mutation. In the present study, the SCTS-mediated modulations of vvpE expression and extracellular VvpE production were also slight attenuated but was still observed in the background of smcR mutation. Taken together, these findings indicate that the QS systems are only partially involved in the SCTS-mediated changes of vvpE expression and extracellular VvpE production. In addition to SmcR or QS signals, another factor is likely to be involved in the SCTS-mediated change of vvpE expression. We found that SCTS prolonged the duration of the stationary growth phase by stimulating V. vulnificus growth, and significantly increased the expression level of vvpE only during the prolonged stationary growth phase in 24-h batch culture. These findings indicate that the duration of the stationary growth phase plays an important role in the stimulation of growth phase-dependent vvpE expression. SCTS-mediated growth stimulation and the resulting prolongation of the stationary growth phase, may increase metabolic or oxidative stress, and result in Crp and/or RpoS increases. Furthermore, Crp and SmcR synergistically affect RpoS-dependent vvpE promoter (3). Our recent research also revealed that Crp functions as an essential activator, and SmcR functions as a modulator for full vvpE expression only in the presence of Crp (4). It has been reported that Crp can affect rpoS expression in V. vulnificus (34). We also recently found that Crp can affect smcR expression in V. vulnificus (submitted). Accordingly, Crp alone or in combination with SmcR may be involved in the SCTS-mediated change of RpoS-dependent vvpE expression.
In addition to vvpE expression, SCTS also appeared to facilitate extracellular VvpE production. In a previous study (5), we found that two forms of VvpE are secreted via type II GSS after being produced within bacterial cells. These suggest that SCTS may also affect the activity of type II GSS. In another study, we found that iron can facilitate the extracellular secretion of cytolysin/hemolysin VvhA by increasing the activity of type II GSS (7). Accordingly, it has been suggested that iron can increase both vvpE expression and extracellular VvpE production (35).
In summary, SCTS was found to facilitate both smcR and vvpE expression by stimulating V. vulnificus growth. However, SCTS-mediated changes in vvpE expression were found to be slightly attenuated in the background of smcR mutation. Accordingly, it is likely that SCTS stimulates the expressions of smcR and vvpE by stimulating V. vulnificus growth, and that SmcR is only partially involved in SCTS-mediated changes in vvpE expression.
Figures and Tables
Figure 1
Effect of a temperature and salinity change from 25℃/2.5% to 37℃/0.9% on smcR expression. After preconditioning by culturing at 25℃/2.5% overnight, the V. vulnificus RC196 strain with the PsmcR::lacZ transcriptional fusion was transferred into fresh broths and cultured at 25℃/2.5% or 37℃/0.9%. Bacterial growth (A) is expressed as the optical densities of culture aliquots at 600 nm (OD600). Accumulated β-galactosidase activity in culture aliquots is expressed in Miller units, and plotted against culture time (B) and bacterial growth (C). Means and standard deviations are of triplicate measurements.
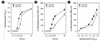
Figure 2
Effect of smcR mutation on the regulation of vvpE expression induced by a change of temperature and salinity from 25℃/2.5% to 37℃/0.9%. After preconditioning by culturing at 25℃/2.5% overnight, the two V. vulnificus strains with PvvpE::lacZ transcriptional fusion, CMM2106 with wild-type smcR (A to C) and RC164 with mutated smcR (D to F) strains, were transferred to fresh broths and cultured at 25℃/2.5% or 37℃/0.9%. Bacterial growth (A and D) is expressed as optical densities of culture aliquots at 600 nm (OD600). Accumulated β-galactosidase activity in culture aliquots is expressed in Miller units, and plotted against culture time (B and E) and bacterial growth (C and F). Means and standard deviations are of triplicate measurements.
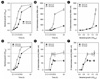
Figure 3
Effect of smcR mutation on the regulation of extracellular VvpE production induced by a change of temperature and salinity from 25℃/2.5% to 37℃/0.9%. After preconditioning by culturing at 25℃/2.5% overnight, the V. vulnificus M06-24/O (wild-type smcR), RC174 (with mutated smcR: SmcR-) and RC186 (with in trans complemented smcR: SmcR+) strains were transferred to fresh broths and cultured at 25℃/2.5% (open symbols) or 37℃/0.9% (filled symbols). (A) Bacterial growth is expressed as optical densities of culture aliquots at 600 nm (OD600). (B) Western blot using rabbit polyclonal anti-VvpE antibody was conducted after the electrophoresis of equal volumes of culture supernatants under denaturing conditions. A representative example of the three times-repeated experiments is shown. Arrows indicate the two forms of VvpE. (C) Quantitative analysis of the Western blot result using densitometry. Total intensity indicates the sum of the intensities of the two VvpE bands.

Table 1
Bacterial strains, plasmids and primers used in this study
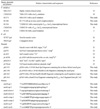
a Vv and Ec stand for V. vulnificus and E. coli, respectively.
b CmR, AmpR, TcR, and KmR stand for chloramphenicol-, ampicillin-, tetracycline-, and kanamycin-resistance, respectively.
c Capital bold letters indicate the restriction enzyme-recognition sequences: CCCGGG for SmaI, ACTAGT for SpeI, AAGCTT for HindIII, GAATTC for EcoRI, and GGATCC for BamHI.
References
1. Jones MK, Oliver JD. Vibrio vulnificus: disease and pathogenesis. Infect Immun. 2009. 77:1723–1733.
2. Shao CP, Hor LI. Metalloprotease is not essential for Vibrio vulnificus virulence in mice. Infect Immun. 2000. 68:3569–3573.


3. Jeong HS, Lee MH, Lee KH, Park SJ, Choi SH. SmcR and cyclic AMP receptor protein coactivate Vibrio vulnificus vvpE encoding elastase through the RpoS-dependent promoter in a synergistic manner. J Biol Chem. 2003. 278:45072–45081.


4. Kim CM, Shin SH. Regulation of the Vibrio vulnificus vvpE expression by cyclic AMP-receptor protein and quorum-sensing regulator SmcR. Microb Pathog. 2010. 49:348–353.


5. Park J, Ryu SY, Kim CM, Shin SH. Two forms of Vibrio vulnificus metalloprotease VvpE are secreted via the type II general secretion system. J Microbiol. 2008. 46:338–343.


6. Hülsmann A, Rosche TM, Kong IS, Hassan HM, Beam DM, Oliver JD. RpoS-dependent stress response and exoenzyme production in Vibrio vulnificus. Appl Environ Microbiol. 2003. 69:6114–6120.


7. Kim CM, Chung YY, Shin SH. Iron differentially regulates gene expression and extracellular secretion of Vibrio vulnificus cytolysin-hemolysin. J Infect Dis. 2009. 200:582–589.


8. Redfield RJ. Is quorum sensing a side effect of diffusion sensing? Trends Microbiol. 2002. 10:365–370.


9. Roh JB, Lee MA, Lee HJ, Kim SM, Cho Y, Kim YJ, et al. Transcriptional regulatory cascade for elastase production in Vibrio vulnificus: LuxO activates luxT expression and LuxT represses smcR expression. J Biol Chem. 2006. 281:34775–34784.


10. Valiente E, Bruhn JB, Nielsen KF, Larsen JL, Roig FJ, Gram L, et al. Vibrio vulnificus produces quorum sensing signals of the AHL-class. FEMS Microbiol Ecol. 2009. 69:16–26.
11. Defoirdt T, Boon N, Sorgeloos P, Verstraete W, Bossier P. Quorum sensing and quorum quenching in Vibrio harveyi: lessons learned from in vivo work. ISME J. 2008. 2:19–26.


12. Carnes EC, Lopez DM, Donegan NP, Cheung A, Gresham H, Timmins GS, et al. Confinement-induced quorum sensing of individual Staphylococcus aureus bacteria. Nat Chem Biol. 2010. 6:41–45.


13. McDougald D, Rice SA, Kjelleberg S. SmcR-dependent regulation of adaptive phenotypes in Vibrio vulnificus. J Bacteriol. 2001. 183:758–762.


14. Jones MK, Warner E, Oliver JD. Survival of and in situ gene expression by Vibrio vulnificus at varying salinities in estuarine environments. Appl Environ Microbiol. 2008. 74:182–187.


15. Shao CP, Hor LI. Regulation of metalloprotease gene expression in Vibrio vulnificus by a Vibrio harveyi LuxR homologue. J Bacteriol. 2001. 183:1369–1375.


16. Kawase T, Miyoshi S, Sultan Z, Shinoda S. Regulation system for protease production in Vibrio vulnificus. FEMS Microbiol Lett. 2004. 240:55–59.
17. Kim CM, Shin SH. Change of Vibrio vulnificus Metalloprotease VvpE production by temperature and salinity. J Bacteriol Virol. 2011. 41:147–156.


18. Kim SY, Lee SE, Kim YR, Kim CM, Ryu PY, Choy HE, et al. Regulation of Vibrio vulnificus virulence by the LuxS quorum-sensing system. Mol Microbiol. 2003. 48:1647–1664.


19. Reddy GP, Hayat U, Abeygunawardana C, Fox C, Wright AC, Maneval DR jr, et al. Purification and determination of the structure of capsular polysaccharide of Vibrio vulnificus M06-24. J Bacteriol. 1992. 174:2620–2630.


20. Miller VL, Mekalanos JJ. A novel suicide vector and its use in construction of insertion mutations: Osmoregulation of outer membrane proteins and virulence determinants in Vibrio cholerae requires toxR. J Bacteriol. 1988. 170:2575–2583.


21. McGee K, Horstedt P, Milton DL. Identification and characterization of additional flagellin genes from Vibrio anguillarum. J Bacteriol. 1996. 178:5188–5198.


22. Farinha MA, Kropinski AM. Construction of broad-host-range plasmid vectors for easy visible selection and analysis of promoters. J Bacteriol. 1990. 172:3496–3499.


23. Staskawicz B, Dahlbeck D, Keen N, Napoli C. Molecular characterization of cloned avirulence genes from race 0 and race 1 of Pseudomonas syringae pv. Glycinea. J Bacteriol. 1987. 169:5789–5794.


24. Ditta G, Stanfield S, Corbin D, Helinski DR. Broad host range DNA cloning system for gram-negative bacteria: construction of a gene bank of Rhizobium meliloti. Proc Natl Acad Sci U S A. 1980. 77:7347–7351.


25. Herrero M, de Lorenzo V, Timmis KN. Transposon vectors containing non-antibiotic resistance selection markers for cloning and stable chromosomal insertion of foreign genes in gram-negative bacteria. J Bacteriol. 1990. 172:6557–6567.


26. Miller JH. A short course in bacterial genetics. 1992. New York: Cold Spring Harbor, Cold Spring Harbor Laboratory Press.
27. Kim CM, Park RY, Chun HJ, Kim SY, Rhee JH, Shin SH. Vibrio vulnificus metalloprotease VvpE is essentially required for swarming. FEMS Microbiol Lett. 2007. 269:170–179.


29. Walker KE, Moghaddame-Jafari S, Lockatell CV, Johnson D, Belas R. ZapA, the IgA-degrading metalloprotease of Proteus mirabilis, is a virulence factor expressed specifically in swarmer cells. Mol Microbiol. 1999. 32:825–836.


30. Lee JH, Rhee JE, Park U, Ju HM, Lee BC, Kim TS, et al. Identification and functional analysis of Vibrio vulnificus SmcR, a novel global regulator. J Microbiol Biotechnol. 2007. 17:325–334.
31. Vendeville A, Winzer K, Heurlier K, Tang CM, Hardie KR. Making 'sense' of metabolism: autoinducer-2, LuxS and pathogenic bacteria. Nat Rev Microbiol. 2005. 3:383–396.


32. Konkel ME, Tilly K. Temperature-regulated expression of bacterial virulence genes. Microbes Infect. 2000. 2:157–166.


33. Maurelli AT, Blackmon B, Curtiss R 3rd. Temperature-dependent expression of virulence genes in Shigella species. Infect Immun. 1984. 43:195–201.

