Abstract
Interferon is an important cytokine that plays a critical role in the initial host defense against viral infection. Recombinant human adenoviruses expressing human interferon-α (Ad-HIFNα) or pig interferon-β fused with interleukin-18 (Ad-PIFNβ-IL18) were constructed and used to induce an early protective response against foot and mouth disease (FMD). To analyze the antiviral effect, bovine thyroid and porcine kidney IBRS-2 cells and ICR mice were treated with Ad-HIFNα, Ad-PIFNβ-IL18, and cocktail of Ad-HIFNα and Ad-PIFNβ-IL18. The survival rate of suckling mice was monitored after foot and mouth disease virus (FMDV) challenge following intra-peritoneal (IP) administration of appropriate adenovirus. Indirect antigen ELISA was performed to evaluate inhibition of FMDV replication following challenge with the FMDV O, A, or Asia 1 serotypes in vitro. These recombinant adenoviruses reduced the replication of FMDV in susceptible cells, thereby decreasing the fatality in mice, suggesting that they can be a useful control method for the early protection against FMD infection in livestock after field trial.
Foot and mouth disease (FMD) is a devastating disease in cattle, swine, sheep, goats, and many wild cloven-hoofed animal species. The causative agent of the disease belongs to the Aphthoviruses genus of the Picornaviridae family and has a positive sense, single-stranded RNA genome. This disease is characterized by temporary and debilitating oral and pedal vesicles (1).
Despite the success of the inactivated vaccine development, a significant portion of FMD research has been focused on the development of novel vaccines which could be manufactured without the concern of virus mutation or the need for high-containment facilities (2). Disease-free countries hesitate to use vaccination to eliminate FMD because the current vaccine contains varying degrees of contaminating viral non-structural (NS) proteins that make it difficult to differentiate infected from vaccinated animals. In addition, the current vaccine requires 7~14 days for protection to fully develop. This concern is particularly relevant to the control of FMD since FMDV replicates and spreads so rapidly. Prior to induction of the adaptive immune response, animals are still susceptible to new infection. As a result, more rapidly acting vaccines are needed. A number of different anti-viral strategies such as interferon, small interference RNA (siRNA) or single chain fragment of antibody (ScFv) have been tested (3~5).
Interferon (IFN) α/β are cytokines produced for a powerful defense against virus infection. The virus-infected cells express IFN and rapidly achieve an anti-viral state in vivo (6). Type I IFN inhibits replication of picornavirus, retrovirus, influenza virus, vesicular stomatitis virus (VSV), herpes simplex virus (HSV), vaccinia virus, adenovirus and reovirus in vitro (7). Interleukin-18 (IL-18) plays an important role in the cell-mediated immune response, in particular through its ability to induce the production of IFN (8).
Recent pharmacokinetic studies indicate that IFN exhibits an extremely short half-life in the blood after parenteral administration. The poor performance of IFNs may have been caused by an insufficient delivery or lack of sustained delivery of the protein to the active site (9). Recently, delivery systems using adenovirus have been recommended as an effective therapeutic strategy to deliver genes and to express foreign protein in mammals (10~12). The use of adenovirus can induce mucosal immunity and stimulate an immune response in the respiratory and digestive systems (13, 14). Furthermore, the advantages of the adenovirus expression system are its ability to infect various cell types and to deliver foreign genes for expression in host animals like cattle and pigs (15). Adenovirus containing porcine IFNα (Ad-PIFNα) has been reported to be effective against FMDV infection (16). It was well known that porcine IFNα had better anti-FMDV effect than IFNβ (4). Furthermore, bovine IFNα also had anti-FMDV effect (17).
In this study, to induce an antiviral state in vivo, we used adenoviruses expressing IFNα or β. Anti-FMDV effects of human IFN has been questionable in porcine or bovine-originated cells and animals. Therefore, we investigated the potential anti-FMDV effect of human IFN as the first line of defense in the animals. In addition, an adenovirus expressing porcine IFNβ and IL-18 was tested to cover the host-specific response for pig with use of human interferon. IL-18, a known inducer of IFNγ, was used for an additional effect by sustaining the delayed anti-viral state. The fused form for porcine cytokines would be effective as a defense strategy for one shot vaccine carrying function for double effects. Cattle and pig cells, in vitro, and sucking mice were infected with the recombinant adenoviruses for the measurement of their antiviral activity and effect on FMDV replication.
Foot and mouth disease (FMD) viruses were isolated by the Animal, Plant and Fisheries Quarantine and Inspection Agency (QIA) in Korea in 2000 and 2002 (O/SKR/2000 or O/SKR/2002). Other FMDV serotypes, O/UKG, Asia1/ISR and A22/Iraq were used for inhibition test of FMDV replication. The viruses were tested in a Bio-safety level (BSL) 3 facility. Human embryonic kidney (HEK) 293A cells were purchased from Q-BIO gene (Carlsbad, CA, USA). For inhibition test in various cell types, primary bovine thyroid and pig-originated IBRS-2 cells were used.
The human IFNalpha (HIFNα) gene was obtained from Cytokine Bank (Chonbuk National University, Korea). The forward (F) and reverse (R) primers for cloning of human IFNα were 5'-GCTCCGGATCCGCTAGCATGTGTGATCTCCCTGAGACCC-3' and 5'-CGTCCGGATCCTTATTCCTTCCTCCTTAATCTTTCTTGC-3', respectively. A BamH I restriction endonuclease site was added to 5' end of the primers for cloning into the expression vector. To obtain porcine IFNβ and IL-18 genes, porcine blood was stimulated with lipopolysaccharide (Sigma-Aldrich, St. Louis, MO, USA). Porcine IFNβ and IL-18 mRNA were extracted and amplified by RT-PCR with IFNβ (IFNβF: 5'-GCTCCGGATCCATGAGCTATGATGTGCTTCGAT-3', IFNβR: 5'-CGTCCGGTACCGTTCCGGAGGTAATCTGTAAGTCTGTT-3') and IL-18 (IL-18F: 5'-CGTCCGGATCCATGTACTTTGGCAAGCTTGAACCTAAAC-3', IL-18R: 5'-GCTCCGGATCCGTTCTTGTTTTGAACAGTGAACATTA-3') specific primers designed in reference to Genbank accession M86762 for porcine IFNβ and AY-450287 for porcine IL-18. PCR was applied with Taq PCR Master Mix (QIAGEN, Hilden, Germany) and the PCR products were cloned to pGEM-T easy vector (Promega, Madison, WI, USA). After insertion of IL-18 gene using Kpn I-linker sequence to the IFNβ-cloned vector for fused gene, the PCR with the primers IFNβF and IL-18R for the fused form was done using a general protocol with an annealing temperature of 56℃. The amplified PCR products of HIFNα and PIFNβ-IL18 were purified by Gene clean III kit (Q-BIO gene, CA, USA). pQBI-AdBM5-GFP (Q-BIO gene) was used as an adenovirus transfer vector by ligation with BamH I-treated PCR products into Bgl II site of the vector (Fig. 1). The HIFNα or PIFNβ-IL18 genes were inserted into cloning site under the control of major late promoter (MLP) of the transfer vector carrying green fluorescent protein (GFP) gene under the control of CMV promoter (pHIFNα, pPIFNβ-IL18). Recombinant adenoviruses were produced in E1 protein expressing HEK 293A cells by Adeno-Quest System (Q-BIO gene). Using fluorescence microscopy, protein expression was examined by fluorescence light emitted from HEK 293A cells.
Serial diluted DNAs of pHIFNα and pPIFNβ-IL18 were transfected using lipofectamine and plus reagent (Invitrogen, NY, USA) in IBRS-2 cells. At 24 h after transfection, cells were infected with FMDV (100 TCID50/0.1 ml) for challenge. Cytopathic effect (CPE) was examined for 4 days, and then the supernatant from infected cells was harvested and assayed for FMDV antigen ELISA. Anti-FMDV monoclonal antibody was coated on the 96 well ELISA plate for 1 h with coating buffer. After the incubation, the plate was washed with PBS-T (PBS with 0.05% tween 20) three times, and blocked with PBS-T containing 5% skim milk for 1 h. The infected cell supernatant was added to the plate and the plate was placed at 37℃ for 1 h. After the incubation, anti-FMDV MAb HRP conjugate (3H4) was added and the reaction was incubated for another hour. A 3,3_,5,5_-tetramethylbenzidine substrate (TMB, Moss Inc., CA, USA) for colorization was incubated for 15 minute, and optical density was examined within 15 min at 450 nm using ELISA reader (Tecan, Männedorf, Switzerland).
Five micrograms of adenoviral DNA (Q-BIO gene, CA, USA) and EcoR I-linearized pHIFNα and pPIFNβ-IL18 DNA were co-transfected by co-precipitation using calcium phosphate in HEK 293A cells. After 24 h, co-transfected cells were transferred to 6 well plates and overlaid with 2 ml of 1.25% SeaPlaque® agarose (Cambrex Bio Science, NJ, USA) containing 2% FBS in DMEM. Within 2 weeks after co-transfection, the recombinant virus was collected from virus plaque and selected by GFP expression. The viruses were re-infected in HEK 293A cells in 175 cm2 culture dishes. When the cells exhibited CPE of 90%, the cells were freeze-thawed three times, and the stock virus was harvested. The virus was amplified upto the 5th passage to obtain a high viral titer.
For protein analysis, recombinant virus infected HEK 293A cells were prepared in lysis buffer and then disrupted by sonication. The clarified supernatant after centrifugation at 13,000 rpm for 10 min was used for 12% sodium dodecyl sulfate polyacrylamide gel electrophoresis (SDS-PAGE). The electrophoresed proteins in a 12% SDS-PAGE gel were transferred to nitrocellulose membrane. Sheep anti-human IFNα (Biosource, NY, USA) or sheep anti-porcine IFNβ (Biosource) was used as a primary antibody for Western blotting. A HRP-rabbit anti-sheep IgG (H + L) (Zymed, NY, USA) was used for secondary detection with a DAB Substrate kit (Pierce, IL, USA).
Porcine originated IBRS-2 or bovine originated bovine thyroid cells were pre-cultured for 24 h in 96 well plates.
Recombinant adenoviruses, Ad-HIFNα and Ad-PIFNβ-IL18, were used to infect the cultured cells by 10-fold dilution. At 24 h after infection, the cells were infected superinfected with FMDV (100 TCID50/0.1 ml) for challenge. CPE was monitored for 7 days, after that period the supernatant from infected cells was assayed using a FMDV antigen ELISA for assessing for antiviral effect. FMDV antigen ELISA was determined from the supernatant for FMDV growth at 72 h after FMDV infection. Cells were infected with Ad-HIFNα or Ad-PIFNβ-IL18 (104.0 TCID50/0.1 ml) and FMDV serotypes (102.0 TCID50/0.1 ml) of O/UKG, Asia1/ISR and A22/Iraq were used to challenge cells at 24 h after infection with recombinant adenoviruses. The control group was inoculated with adenovirus expressing lamin-shRNA (Ad-control) (Invitrogen, NY, USA).
All experimental animals were cared for according to the animal management guideline of QIA in Korea. Ten of 7 day-old suckling ICR mice per group were injected with Ad-HIFNα (106.5 TCID50/0.1 ml) and Ad-PIFNβ-IL18 (107.5 TCID50/0.1 ml). After 24 h, mice were injected with FMDV (10 to 125 LD50/0.1 ml) for challenge and their survival rate was observed for following 10 days post-challenge.
IBRS-2 cells were transfected with plasmids containing the antiviral genes HIFNα and PIFNβ-IL18 at various concentrations for comparison of antiviral effects. After 24 h, the cells were infected by FMDV (100 TCID50/0.1 ml). After 48 h, the supernatant was harvested for FMDV antigen quantitation. The plasmid containing HIFNα gene (pHIFNα) showed a better anti-FMDV effect than pPIFNβ-PIL18 (Fig. 2). The lower concentrations of pPIFNβ-IL18 had very little effect on FMDV replication, but a high concentration (1 µg) had a measurable antiviral effect. The plasmid mixture of pHIFNα and pPIFNβ-IL18 showed antiviral activity at the same level as pHIFNα. Both plasmids at a concentration of 1 or 2 µg showed strong anti-FMDV activities, but the pPIFNβ-IL18 at concentration of 3.9 ng to 0.5 µg could not inhibit FMDV replication (Fig. 2).
The molecular weight of proteins expressed in HEK 293A cells was 25 kDa for Ad-HIFNα, and 53 kDa for Ad-PIFNβ-IL18, as determined by Western blot assay (Fig. 3).
To identify inhibition of FMDV replication after recombinant adenovirus infection, FMDV antigen ELISA was performed using the supernatant collected 48 h after FMDV challenge following the adenovirus infection for 24 h in bovine thyroid or IBRS-2 cells. Ad-HIFNα showed strong antiviral effect as demonstrated using plasmid transfection (Fig. 4). The contribution of the PIFNβ-IL18 appeared to be negligible.
Suckling mice were infected with either Ad-HIFNα or Ad-PIFNβ-IL18. As shown in Fig. 5, the group with recombinant adenovirus had a longer survival rate compared to the control group. The group of Ad-HIFNα or Ad-PIFNβ-IL18 showed extended survival of 2 days compared with control group (Fig. 5 and Fig 6). Among the groups, the group infected with Ad-HIFNα had the highest survival rate when inoculated repeatedly.
The action of IFN is the first line of defense mechanism to protect against viral infection. The activated cell secretes IFN which binds to the cell receptor, and remains in antiviral states at broad regions. Human adenovirus type 5 has been proven as an effective vector for expression of foreign protein and gene delivery to induce humoral or cellular immunity in digestive and respiratory organs in animals (10~15).
The epithelial surface in oropharyngeal region is favored for entry by many kinds of viruses, bacteria and parasites. Immune responses in mucosal region are an important defense mechanism to protect against the first infection of microorganism. The first replication site of FMDV is an epithelial surface in oropharyngeal region, which is unavailable for the conventional inactivated vaccine. This disadvantage could be overcome by introduction of antiviral molecules into the oropharyngeal regions and could provide a safer tool to protect against FMDV infection.
It has been shown that the pigs infected by adenovirus expressing porcine IFNα by intramuscular (IM) route were protected after FMDV challenge by the same route (16).
In this study, human IFNα showed anti-FMDV activity. Human IFN could be used in many different species that are susceptible to FMDV infection, including pig, cattle and other target species. However, previous study strategies have utilized porcine IFN which is limited to pigs (4). For pigs as a target animal, porcine IFNβ/IL-18 (PIFNβ-IL18) was constructed additionally. Anti-FMDV activity in IBRS-2 cell and suckling mice was assayed by expression of HIFNα and PIFNβ-IL18. We proved that the strategy using HIFNα elicited a better antiviral effect independent of recombinant adenoviral titers. pPIFNβ-IL18 showed anti-FMDV effect only at high concentrations in IBRS-2 cells, and the groups inoculated with Ad-PIFNβ-IL18 showed low survival rates in suckling mice. Using an inhibition test of FMDV replication with the various cell types, an anti-FMDV effect in both bovine and porcine-originated cells was shown (Fig. 4). The results might also demonstrate protection in vivo of susceptible animals like cattle or pigs if a similar mechanism is required. Furthermore, we found the possibility for enhancing the survival of FMDV infected mice by repeat injection until a maximum of 4 injections of the recombinant adenovirus. This phenomenon shows the relationship with the number of injections and duration of the gene expression in vivo because of the short half life of IFN.
To overcome the many disadvantages of the conventional inactivated FMD vaccine, we constructed recombinant adenovirus delivering HIFNα which exhibited an anti-FMDV activity. The constructs are expected to protect transmission of FMDV in replication sites as well as early infection in various viral diseases by the same mechanism of enhancing non-specific innate immunity. A further experiment will be carried out to establish if human IFN works in target animals such as cattle and pigs.
Figures and Tables
Figure 1
Construction of recombinant adenovirus expressing HIFNα or PIFNβ-IL18. (A) Schematic diagram of genome organization of recombinant adenovirus, Ad5; adenovirus serotype 5, LITR; left inverted terminal repeat, RITR; right inverted terminal repeat, ΔE1; deletion of E1 gene, ΔE3; deletion of E3 gene, pA; β-globin poly A, MLP; adenoviral major late promoter, CMV; cytomegalovirus promoter, GFP; green fluorescent protein. (B) Sequences of the adenoviral DNA (Ad-HIFN and Ad-PIFNβ-IL18) in cloning region for foreign gene.
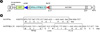
Figure 2
Inhibition of FMDV replication by anti-viral plasmids in IBRS-2 cells. This result shows inhibition of FMDV replication by transfection of antiviral plasmids in IBRS-2 cells. The plasmids containing HIFNα or PIFNβ/IL18 fusion genes (pHIFNα and pPIFNβ-PIL18) demonstrated anti-FMDV potential. Optical density in only FMDV-infected or mock-infected cells was 3.37±0.0332 or 0.06±0.0035, respectively.
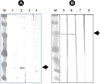
Figure 3
Western blot analysis of HIFNα, PIFNβ-IL18 expressed by recombinant adenovirus in HEK 293A cells. Lysates of HEK 293A cells infected with recombinant adenovirus were analyzed by transferring to nitrocellulose membranes for Western blot analysis. Lane M; protein molecular weight marker (BIO-RAD). Lane 1; cell lysates from Ad-HIFNα infected HEK 293A cells. Lane 2; supernatant from Ad-HIFNα infected HEK 293A cell. Lane 3, 7; cell lysate from mock-infected HEK 293A cell. Lane 4, 8; supernatant of mock-infected HEK 293A cell. Lane 5; cell lysate of Ad-PIFNβ-PIL18 infected HEK 293A cell. Lane 6; supernatant from Ad-PIFNβ-PIL18 infected HEK 293A cell.
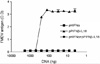
Figure 4
Anti-FMDV activity by recombinant adenoviruses in bovine and porcine-originated cells. FMDV antigen ELISA was determined from the supernatant taken at 72 h after FMDV infection. Cells were infected with Ad-HIFNα or Ad-PIFNβ-IL18 (104.0 TCID50/0.1 ml) and FMDV serotypes (102.0 TCID50/0.1 ml) of O/UKG, Asia1/ISR and A22/Iraq was used to challenge cells at 24 h after infection with the recombinant adenoviruses. The control group for adenovirus control (Ad-control) was inoculated adenovirus expressing lamin-shRNA.
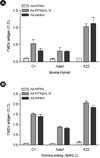
Figure 5
Fatality in suckling mice following adenovirus treatment and FMDV challenge. (A) Mice were challenged with FMDV (20 LD50/0.1 ml) at 24 h after recombinant adenovirus (106 TCID50/0.1 ml) inoculation by intraperitoneal (IP) route. (B) Mice were challenged with FMDV O/SKR/2002 (125 LD50/0.1 ml) at 24 h. To determine synergic effect by co-administration with adenoviruses, high dose of FMDV (125 LD50/0.1 ml) and adenovirus (Ad-HIFNα, 106.5 TCID50/0.1 ml and Ad-PIFNβ-IL18, 107.5 TCID50/0.1 ml) were administrated to suckling ICR mice by intraperitoneal (IP) route. The control group (control) was only inoculated with FMDV.
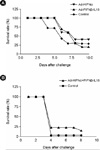
Figure 6
Change of fatality in suckling mice following several adenovirus administration and FMDV challenge. Recombinant adenoviruses (106.0 TCID50/0.1 ml) were inoculated by intraperitoneal (IP) route at -1, 3 DPC (2 round injection group, 2X) and at -1, 0, 3 and 6 DPC (4 round injection group, 4X) in sucking ICR mice. The mice were 7-day old when challenged by FMDV O/SKR/2002 (20 LD50/mouse). The control group for adenovirus (Ad-con) was inoculated adenovirus expressing lamin-shRNA. The control group (control) was only inoculated with FMDV.
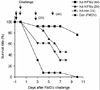
References
2. Edward S. Manual of Dignostic Tests and Vaccines for Terrestrial. 2004. 5th ed. Paris: OIE;111–128.
3. De los Santos T, Wu Q, De Avila Bottlon S, Grubman MJ. Short hairpin RNA targeted to the highly conserved 2B nonstructural protein coding region inhibits replication of multiple serotypes of foot-and-mouth disease virus. Virology. 2005. 335:222–231.


4. Moraes MP, Chinsangaram J, Brum MC, Grubman MJ. Immediate protection of swine from foot-and-mouth disease: a combination of adenoviruses expressing interferon alpha and a foot-and-mouth disease virus subunit vaccine. Vaccine. 2003. 22:268–279.


5. ShengFeng C, Ping L, Tao S, Xin W, Guo Feng W. Construction expression, purification, refold and activity assay of a specific scFv fragment against foot and mouth disease virus. Vet Res Commun. 2003. 27:243–256.
6. Zhou A, Paranjape JM, Der SD, Wiliams BR, Silverman RH. Interferon action in triply deficient mice reveals the existence of alternative antiviral pathways. Virology. 1999. 258:435–440.


7. Stark GR, Kerr IM, Wiliams BR, Silverman RH, Schreiber RD. How cells respond to interferons. Annu Rev Biochem. 1998. 67:227–264.


8. Fournout S, Dozois CM, Yerle M, Pinton P, Fairbrother JM, Oswald E, et al. Cloning, chromosomal location, and tissue expression of the gene for pig interleukin-18. Immunogenetics. 2000. 51:358–365.


9. Qin XQ, Tao N, Dergay A, Moy P, Fawell S, Davis A, et al. Interferon-beta gene therapy inhibits tumor formation and causes regression of established tumors in immune-deficient mice. Proc Natl Acad Sci U S A. 1998. 95:14411–14416.


10. Ertl HC, Xiang Z. Novel vaccine approaches. J Immunol. 1996. 156:3579–3582.
11. Hsu KH, Lubeck MD, Bhat BM, Bhat RA, Kostek B, Selling BH, et al. Efficacy of adenovirus-vectored respiratory syncytial virus vaccines in a new ferret model. Vaccine. 1994. 12:607–612.


12. Mittal SK, McDermott MR, Johnson DC, Prevec L, Graham FL. Monitoring foreign gene expression by a human adenovirus-based vector using the firefly luciferase gene as a reporter. Virus Res. 1993. 28:67–90.


13. Gogev S, Georgin JP, Schynts F, Vanderplasschen A, Thiry E. Bovine herpesvirus 1 glycoprotein D expression in bovine upper respiratory tract mediated by a human adenovirus type 5. Vet Res. 2004. 35:715–721.


14. Zhao J, Lou Y, Pinczewski J, Malkevitch N, Aldrich K, Kalyanaraman VS, et al. Boosting of SIV-specific immune responses in rhesus macaques by repeated administration of Ad5hr-SIVenv/rev and Ad5hr-SIVgag recombinants. Vaccine. 2003. 21:4022–4035.


15. Prevec L, Schneider M, Rosenthal KL, Belbeck LW, Derbyshire JB, Graham FL. Use of human adenovirus-based vectors for antigen expression in animals. J Gen Virol. 1989. 70:429–434.

