Abstract
The synuclein family consists of three distinct genes, α-synuclein, β-synuclein, and γ-synuclein. The α-synuclein and β-synuclein are predominately expressed in brain and especially α-synuclein is related with Parkinson's disease, Alzheimer's disease, and dementia with Lewy bodies. The γ-synuclein was first identified as breast cancer specific gene 1. It is expressed in the peripheral nervous system and also detected in breast and ovarian cancers. The γ-synuclein is also known to mediate metastasis of breast and ovarian cancer cells. Insulin-like growth factor 1 (IGF-I) is one of the growth factors that plays an important role in cell proliferation and migration in cancer cells, as well as in normal cells. In this study, we investigated the migrations of SKOV-3, MDAMB-231, and HeLa cells by the recombinant synuclein proteins (α-, β-, and γ-synucleins) and IGF-I and the molecular mechanism. Furthermore, we investigated the membrane ruffle formation of SKOV-3 cells by recombinant synuclein proteins and IGF-I. As a result, synucleins and IGF-I were found to induce cancer cell migrations. Simultaneous synucleins and IGF-I treatment on the cancer cells induced more migrations than the individual synuclein or IGF-I treatments. The synucleins or IGF-I treatments increased the expressions of membrane-type1 matrix metalloproteinase (MT1-MMP) and cluster of differentiation 44 (CD44). Moreover, simultaneous synucleins and IGF-I treatments further increased the expressions of MT1-MMP and CD44. The synucleins and IGF-I promoted the conformational change of actin filaments, and then this led to the membrane ruffle formation.
Figures and Tables
Figure 1
SDS-PAGE analysis of the purified recombinant synucleins. The purified recombinant synucleins were separated by 15% SDS-PAGE and stained with Coomassie brilliant blue. α-synuclein (α-syn), β-synuclein (β-syn), and γ-synuclein (γ-syn).

Figure 2
The recombinant synucleins induced cancer cell migration. SKOV-3, MDAMB-231, HeLa cells were treated with 100 nM and 500 nM of the recombinant synucleins for 24 h. (A) The wound gaps of SKOV-3 cells at 0 h and 24 h. The gaps of 0 h and 24 h were compared by ImageJ. (B) SKOV-3, (C) MDAMB-231, (D) HeLa cells were quantified (n = 3). Scale bar, 500 µm. *p < 0.05, **p < 0.001.
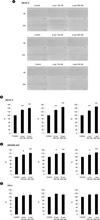
Figure 3
IGF-I induced cancer cell migration. SKOV-3, MDAMB-231, HeLa cells were treated with 1 to 100 ng/ml of IGF-I for 24 h. (A) The wound gaps of SKOV-3 cells at 0 h and 24 h. The gaps of 0 h and 24 h were compared by ImageJ. (B) SKOV-3, (C) MDAMB-231, (D) HeLa cells were quantified (n = 3). Scale bar, 500 µm. *p < 0.05.
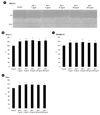
Figure 4
The recombinant synucleins and IGF-I induced cancer cell migration. SKOV-3, MDAMB-231, HeLa cells were treated with 5 ng/ml of IGF-I and 500 nM of the recombinant synucleins for 24 h. (A) The wound gaps of SKOV-3 cells at 0 h and 24 h. The gaps of 0 h and 24 h were compared by ImageJ. (B) SKOV-3, (C) MDAMB-231, (D) HeLa cells were quantified (n = 3). Scale bar, 500 µm. *p < 0.05.
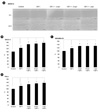
Figure 5
The recombinant synucleins induced the expression of MT1-MMP. For investigation of the expression of MT1-MMP, FACS analysis was accomplished. SKOV-3 cells were treated with 500 nM of (A) α-synuclein, (B) β-synuclein, and (C) γ-synuclein for 24 h. MDAMB-231 cells were treated with 500 nM of (D) α-synuclein, (E) β-synuclein, and (F) γ-synuclein for 24 h. HeLa cells were treated with 500 nM of (G) α-synuclein, (H) β-synuclein, and (I) γ-synuclein for 24 h. Solid gray, unstained (uns). Tinted gray with line, control (con). Bold black line, recombinant synucleins.
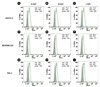
Figure 6
The recombinant synucleins and IGF-I induced the expression of MT1-MMP. For investigation of the expression of MT1-MMP, FACS analysis was accomplished. SKOV-3 cells were treated with (A) 5 ng/ml of IGF-I, 5 ng/ml of IGF-I and 500 nM of (B) α-synuclein, (C) β-synuclein, and (D) γ-synuclein for 24 h. MDAMB-231 cells were treated with (E) 5 ng/ml of IGF-I, 5 ng/ml of IGF-I and 500 nM of (F) α-synuclein, (G) β-synuclein, and (H) γ-synuclein for 24 h. HeLa cells were treated with (I) 5 ng/ml of IGF-I, 5 ng/ml of IGF-I and 500 nM of (J) α-synuclein, (K) β-synuclein, and (L) γ-synuclein for 24 h. Solid gray, unstained. Tinted gray with line, control (con). Gray line, IGF-I. Bold black line, recombinant synucleins.
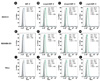
Figure 7
The recombinant synucleins induced the expression of CD44. For investigation of the expression of CD44, FACS analysis was accomplished. SKOV-3 cells were treated with 500 nM of (A) α-synuclein, (B) β-synuclein, and (C) γ-synuclein for 24 h. MDAMB-231 cells were treated with 500 nM of (D) α-synuclein, (E) β-synuclein, and (F) γ-synuclein for 24 h. HeLa cells were treated with 500 nM of (G) α-synuclein, (H) β-synuclein, and (I) γ-synuclein for 24 h. Solid gray, unstained. Tinted gray with line, control (con). Bold black line, recombinant synucleins.
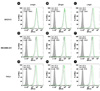
Figure 8
The recombinant synucleins and IGF-I induced the expression of CD44. For investigation of the expression of CD44, FACS analysis was accomplished. SKOV-3 cells were treated with (A) 5 ng/ml of IGF-I, 5 ng/ml of IGF-I and 500 nM of (B) α-synuclein, (C) β-synuclein, and (D) γ-synuclein for 24 h. MDAMB-231 cells were treated with (E) 5 ng/ml of IGF-I, 5 ng/ml of IGF-I and 500 nM of (F) α-synuclein, (G) β-synuclein, and (H) γ-synuclein for 24 h. HeLa cells were treated with (I) 5 ng/ml of IGF-I, 5 ng/ml of IGF-I and 500 nM of (J) α-synuclein, (K) β-synuclein, and (L) γ-synuclein for 24 h. Solid gray, unstained. Tinted gray with line, control (con). Gray line, IGF-I. Bold black line, recombinant synucleins.

Figure 9
The recombinant synucleins and IGF-I induced the membrane ruffling formation of SKOV-3. For investigation of the membrane ruffling formation, confocal microscopy was accomplished. (A) as control. SKOV-3 cells were treated with 500 nM of (B) α-synuclein, (C) β-synuclein, and (D) γ-synuclein, (E) 5 ng/ml of IGF-I, 5 ng/ml of IGF-I and 500 nM of (F) α-synuclein, (G) β-synuclein, and (H) γ-synuclein for 24 h. For F-actin staining, cells were stained with rhodamine phalloidin. Scale bar, 20 µm.
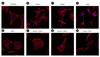
Table 1
Endotoxin contents of before and after removing the endotoxin from the purified recombinant synucleins. For removing the endotoxin, the recombinant synucleins were reacted in Detoxi-Gel Endotoxin Removing Column. Before and after removing the endotoxin, endotoxin contents were quantified by LAL test.
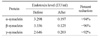
References
1. George JM. The synucleins. Genome Biol. 2002. 3:REVIEWS3002.
2. Weinreb PH, Zhen W, Poon AW, Conway KA, Lansbury PT Jr. NACP, a protein implicated in Alzheimer's disease and learning, is natively unfolded. Biochemistry. 1996. 35:13709–13715.


3. Iwai A, Masliah E, Yoshimoto M, Ge N, Flanagan L, de Silva HA, et al. The precursor protein of non-A beta component of Alzheimer's disease amyloid is a presynaptic protein of the central nervous system. Neuron. 1995. 14:467–475.


4. Nakajo S, Shioda S, Nakai Y, Nakaya K. Localization of phosphoneuroprotein 14 (PNP 14) and its mRNA expression in rat brain determined by immunocytochemistry and in situ hybridization. Brain Res Mol Brain Res. 1994. 27:81–86.


6. George JM, Jin H, Woods WS, Clayton DF. Characterization of a novel protein regulated during the critical period for song learning in the zebra finch. Neuron. 1995. 15:361–372.


7. Jenco JM, Rawlingson A, Daniels B, Morris AJ. Regulation of phospholipase D2: selective inhibition of mammalian phospholipase D isoenzymes by alpha- and beta-synucleins. Biochemistry. 1998. 37:4901–4909.


8. Colley WC, Sung TC, Roll R, Jenco J, Hammond SM, Altshuller Y, et al. Phospholipase D2, a distinct phospholipase D isoform with novel regulatory properties that provokes cytoskeletal reorganization. Curr Biol. 1997. 7:191–201.


9. Abeliovich A, Schmitz Y, Fariñas I, Choi-Lundberg D, Ho WH, Castillo PE, et al. Mice lacking alpha-synuclein display functional deficits in the nigrostriatal dopamine system. Neuron. 2000. 25:239–252.


10. Murphy DD, Rueter SM, Trojanowski JQ, Lee VM. Synucleins are developmentally expressed, and alpha-synuclein regulates the size of the presynaptic vesicular pool in primary hippocampal neurons. J Neurosci. 2000. 20:3214–3220.


11. Ji H, Liu YE, Jia T, Wang M, Liu J, Xiao G, et al. Identification of a breast cancer-specific gene, BCSG1, by direct differential cDNA sequencing. Cancer Res. 1997. 57:759–764.
12. Buchman VL, Adu J, Pinõn LG, Ninkina NN, Davies AM. Persyn, a member of the synuclein family, influences neurofilament network integrity. Nat Neurosci. 1998. 1:101–103.


14. Duda JE, Shah U, Arnold SE, Lee VM, Trojanowski JQ. The expression of alpha-, beta-, and gamma-synucleins in olfactory mucosa from patients with and without neurodegenerative diseases. Exp Neurol. 1999. 160:515–522.


15. Zhao W, Liu H, Liu W, Wu Y, Chen W, Jiang B, et al. Abnormal activation of the synuclein-gamma gene in hepatocellular carcinomas by epigenetic alteration. Int J Oncol. 2006. 28:1081–1088.


16. Yanagawa N, Tamura G, Honda T, Endoh M, Nishizuka S, Motoyama T. Demethylation of the synuclein gamma gene CpG island in primary gastric cancers and gastric cancer cell lines. Clin Cancer Res. 2004. 10:2447–2451.


17. Hibi T, Mori T, Fukuma M, Yamazaki K, Hashiguchi A, Yamada T, et al. Synuclein-gamma is closely involved in perineural invasion and distant metastasis in mouse models and is a novel prognostic factor in pancreatic cancer. Clin Cancer Res. 2009. 15:2864–2871.


18. Bruening W, Giasson BI, Klein-Szanto AJ, Lee VM, Trojanowski JQ, Godwin AK. Synucleins are expressed in the majority of breast and ovarian carcinomas and in preneoplastic lesions of the ovary. Cancer. 2000. 88:2154–2163.


19. Jia T, Liu YE, Liu J, Shi YE. Stimulation of breast cancer invasion and metastasis by synuclein gamma. Cancer Res. 1999. 59:742–747.
20. Roskelley CD, Bissell MJ. The dominance of the microenvironment in breast and ovarian cancer. Semin Cancer Biol. 2002. 12:97–104.


21. Fidler IJ. Critical factors in the biology of human cancer metastasis: twenty-eighth G.H.A. Clowes memorial award lecture. Cancer Res. 1990. 50:6130–6138.
22. Cauwe B, Van den Steen PE, Opdenakker G. The biochemical, biological, and pathological kaleidoscope of cell surface substrates processed by matrix metalloproteinases. Crit Rev Biochem Mol Biol. 2007. 42:113–185.


23. Liotta LA, Tryggvason K, Garbisa S, Hart I, Foltz CM, Shafie S. Metastatic potential correlates with enzymatic degradation of basement membrane collagen. Nature. 1980. 284:67–68.


24. Seiki M. The cell surface: the stage for matrix metalloproteinase regulation of migration. Curr Opin Cell Biol. 2002. 14:624–632.


25. Kawashima M, Suzuki SO, Doh-ura K, Iwaki T. alpha-Synuclein is expressed in a variety of brain tumors showing neuronal differentiation. Acta Neuropathol. 2000. 99:154–160.


26. Fung KM, Rorke LB, Giasson B, Lee VM, Trojanowski JQ. Expression of alpha-, beta-, and gamma-synuclein in glial tumors and medulloblastomas. Acta Neuropathol. 2003. 106:167–175.
27. Cairns RA, Khokha R, Hill RP. Molecular mechanisms of tumor invasion and metastasis: an integrated view. Curr Mol Med. 2003. 3:659–671.


28. Kim S, Cho SH, Kim KY, Shin KY, Kim HS, Park CH, et al. Alpha-synuclein induces migration of BV-2 microglial cells by up-regulation of CD44 and MT1-MMP. J Neurochem. 2009. 109:1483–1496.


29. Pan ZZ, Bruening W, Godwin AK. Involvement of RHO GTPases and ERK in synuclein-gamma enhanced cancer cell motility. Int J Oncol. 2006. 29:1201–1205.
30. Sjögren K, Liu JL, Blad K, Skrtic S, Vidal O, Wallenius V, et al. Liver-derived insulin-like growth factor I (IGF-I) is the principal source of IGF-I in blood but is not required for postnatal body growth in mice. Proc Natl Acad Sci U S A. 1999. 96:7088–7092.


31. Isaksson OG, Lindahl A, Nilsson A, Isgaard J. Mechanism of the stimulatory effect of growth hormone on longitudinal bone growth. Endocr Rev. 1987. 8:426–438.


32. Grey A, Chen Q, Xu X, Callon K, Cornish J. Parallel phosphatidylinositol-3 kinase and p42/44 mitogen-activated protein kinase signaling pathways subserve the mitogenic and antiapoptotic actions of insulin-like growth factor I in osteoblastic cells. Endocrinology. 2003. 144:4886–4893.


33. Chan JM, Stampfer MJ, Giovannucci E, Gann PH, Ma J, Wilkinson P, et al. Plasma insulin-like growth factor-I and prostate cancer risk: a prospective study. Science. 1998. 279:563–566.


34. Hankinson SE, Willett WC, Colditz GA, Hunter DJ, Michaud DS, Deroo B, et al. Circulating concentrations of insulin-like growth factor-I and risk of breast cancer. Lancet. 1998. 351:1393–1396.


35. Ma J, Pollak MN, Giovannucci E, Chan JM, Tao Y, Hennekens CH, et al. Prospective study of colorectal cancer risk in men and plasma levels of insulin-like growth factor (IGF)-I and IGF-binding protein-3. J Natl Cancer Inst. 1999. 91:620–625.


36. Yu H, Spitz MR, Mistry J, Gu J, Hong WK, Wu X. Plasma levels of insulin-like growth factor-I and lung cancer risk: a case-control analysis. J Natl Cancer Inst. 1999. 91:151–156.


37. Peyrat JP, Bonneterre J, Hecquet B, Vennin P, Louchez MM, Fournier C, et al. Plasma insulin-like growth factor-1 (IGF-1) concentrations in human breast cancer. Eur J Cancer. 1993. 29A:492–497.


38. Reinmuth N, Fan F, Liu W, Parikh AA, Stoeltzing O, Jung YD, et al. Impact of insulin-like growth factor receptor-I function on angiogenesis, growth, and metastasis of colon cancer. Lab Invest. 2002. 82:1377–1389.


39. Dunn SE, Ehrlich M, Sharp NJ, Reiss K, Solomon G, Hawkins R, et al. A dominant negative mutant of the insulin-like growth factor-I receptor inhibits the adhesion, invasion, and metastasis of breast cancer. Cancer Res. 1998. 58:3353–3361.
40. Prager D, Li HL, Asa S, Melmed S. Dominant negative inhibition of tumorigenesis in vivo by human insulin-like growth factor I receptor mutant. Proc Natl Acad Sci U S A. 1994. 91:2181–2185.


41. Li M, Yin Y, Hua H, Sun X, Luo T, Wang J, et al. The reciprocal regulation of gamma-synuclein and IGF-I receptor expression creates a circuit that modulates IGF-I signaling. J Biol Chem. 2010. 285:30480–30488.


42. Ahn KJ, Paik SR, Chung KC, Kim J. Amino acid sequence motifs and mechanistic features of the membrane translocation of alpha-synuclein. J Neurochem. 2006. 97:265–279.


43. Harmey JH, Bucana CD, Lu W, Byrne AM, McDonnell S, Lynch C, et al. Lipopolysaccharide-induced metastatic growth is associated with increased angiogenesis, vascular permeability and tumor cell invasion. Int J Cancer. 2002. 101:415–422.


44. Wang JH, Manning BJ, Wu QD, Blankson S, Bouchier-Hayes D, Redmond HP. Endotoxin/lipopolysaccharide activates NF-kappa B and enhances tumor cell adhesion and invasion through a beta 1 integrin-dependent mechanism. J Immunol. 2003. 170:795–804.


45. Xie W, Huang Y, Xie W, Guo A, Wu W. Bacteria peptidoglycan promoted breast cancer cell invasiveness and adhesiveness by targeting toll-like receptor 2 in the cancer cells. PLoS One. 2010. 5:e10850.


46. Issekutz AC. Removal of gram-negative endotoxin from solutions by affinity chromatography. J Immunol Methods. 1983. 61:275–281.


47. Munson TE. Guideline for validation of the LAL test as an end-product endotoxin test for human and biological drug products. Prog Clin Biol Res. 1985. 189:211–220.
48. Kim EK, Yun SJ, Ha JM, Kim YW, Jin IH, Yun J, et al. Selective activation of Akt1 by mammalian target of rapamycin complex 2 regulates cancer cell migration, invasion, and metastasis. Oncogene. 2011. 30:2954–2963.


49. Ma QL, Chan P, Yoshii M, Uéda K. Alpha-synuclein aggregation and neurodegenerative diseases. J Alzheimers Dis. 2003. 5:139–148.
50. Manivel P, Muthukumaran J, Kannan M, Krishna R. Insight into residues involved in the structure and function of the breast cancer associated protein human gamma synuclein. J Mol Model. 2011. 17:251–263.


51. Jiang Y, Liu YE, Lu A, Gupta A, Goldberg ID, Liu J, et al. Stimulation of estrogen receptor signaling by gamma synuclein. Cancer Res. 2003. 63:3899–3903.