Abstract
Background
The MHC region of the chromosome contains a lot of genes involved in immune responses. Here we have investigated the mouse NG29/Cd320 gene in the centrometrically extended MHC region of chromosome 17.
Methods
We cloned the NG29 gene by RT-PCR and confirmed the tissue distribution of its gene expression by northern blot hybridization. We generated the NG29 gene expression constructs and polyclonal antibody against the NG29 protein to perform the immunofluorescence, immunoprecipitation and flow cytometric analysis.
Results
The murine NG29 gene and its human homologue, the CD320/8D6 gene, were similar in the gene structure and tissue expression patterns. We cloned the NG29 gene and confirmed its expression in plasma membrane and intracellular compartments by transfecting its expresssion constructs into HEK 293T cells. The immunoprecipitation studies with rabbit polyclonal antibody raised against the NG29-NusA fusion protein indicated that NG29 protein was a glycoprotein of about 45 kDa size. A flow cytometric analysis also showed the NG29 expression on the surface of Raw 264.7 macrophage cell line.
The major histocompatibility complex (MHC) is a genomic region that spans about 2 centimorgans on murine chromosome 17 and contains more than 200 genes that have specialized functions in innate and adaptive immunity (1-3). The MHC region is distinguished from other regions of genome by a high gene density with about 40% genes having immune system functions. Conventionally, MHC is subdivided into three regions termed class I (telomeric), class III (central), and class II (centromeric). Immunologic functions of genes in the MHC region include antigen processing and presentation (LMP and TAP); innate immunity, inflammation, and regulation of immunity (C4, HSP70, TNF, and so on); and intercellular interactions via peptide-presenting MHC class I and II molecules or non-classical MHC molecules (4,5). Whereas the central class III MHC region encodes more than 60 genes including genes involved in the activation cascades of the complement system, hormone synthesis, and inflammation, there are another extended region of immune-related gene cluster located centromerically of MHC (6), which includes TAPASIN, DAXX, and RGL2. Since the linkage is conserved in different species, this region was proposed as the extended MHC region (3).
The physiological roles for most of genes in this region are yet to be determined. In this region, we paid attention to genes that encode cell surface proteins involved in interactions among lymphocytes, antigen-presenting cells, and various types of hematopoetic and non-hematopoietic stromal cells. Non-hematopoietic stromal cells in the lymphoid tissue participate in the formation and development of lymphoid tissues and immune responses through cognate interactions with lymphocytes and other types of stromal cells (7). For example, thymic stromal cells provide critical signals for T cell differentiation (8) and follicular dendritic cells are essential for maintenance of B cell population and germinal center reaction (9). These stromal cells appear to be highly pleomorphic in morphology and molecular properties and support compartmentalized functions in different anatomic compartments of primary and secondary lymphoid organs (10,11). Some monoclonal antibodies were shown to define subsets of stromal cells in lymph nodes, but the molecular identities are not yet known (12,13). Macrophages and dendritic cells are also comprised of several different subsets, which have different cell surface markers and functions. To clearly define the functional relationships between various types of lymphocytes and stromal cells, new cell surface molecules or receptors need to be elucidated.
In this study, we report the characterization of Cd320/NG29, a murine transmembrane protein, whose gene is located in the centrometrically extended MHC region of mouse. Its homologue was found to be the human CD320/8D6, which was reported to be a follicular dendritic cell surface molecule and support B cell proliferation and plasma cell differentiation (14,15). We here provide data supporting that the Cd320/NG29 gene is the murine orthologue for the human CD320/8D6 gene, but it appeared that the functions of NG29 protein is not confined to follicular dendritic cells since their expression was widespread in different types of cells and tissues, especially in macrophages, dendritic cells, fibroblasts, and kidney.
Total RNA was extracted from Balb/c mouse kidney and spleen with RNAzol agent (Gibco/BRL, Gaithersberg, MD). For reverse transcription into cDNA, 5µg of RNA were incubated at 37℃ for 90 min, heat-inactivated at 70℃ for 10 min with random hexamer primers and subjected to Power-Script™ Reverse Transcriptase (Clontech, Palo Alto, CA). For generation of full-length NG29 cDNA clone, PCR reaction was performed as the following amplification cycle; denaturation at 94℃ for 1 min, annealing at 52℃ for 1 min, extension at 72℃ for 2 min for 35 cycles. A pair of oligonucleotide primers were used (5'-GATGAATTCATGGCGCGGGGCGGA-3' and 5'-CACTCGAGTCAGATCAGAGAGGTTTTC-3'). After purifying the resulting PCR product electrophoretically, DNA fragment was inserted into TOPO-TA-cloning vector (Invitrogene, Carlsbad, CA) and confirmed by sequencing. Subsequently the NG29 cDNA was inserted into NheI and HindIII site of pcDNA3.1/myc and pEGFP-N3 vectors (Invitrogen). For the fusion construct of the extracellular domain of NG29 and NusA (NG29ext-NusA vector), the corresponding NG29 sequence was amplified by PCR using a pair of primers, 5'-AGATCTatggcgcggggcggagct-3' and 5'-AAGCTTCacgtggctttcgggatgagtc-3' and then cloned into pET NusA vector (Merck, Darmstadt, Germany).
HEK-293T, Raw 264.7, and NIH-3T3 cells were grown in DMEM media (Gibco/BRL, Gaithersburg, MD) supplemented with 3.7% sodium bicarbonate (Sigma, Deisenhofen, Germany), 2 mM glutamine, and 10% fetal bovine serum (Gibco) under 5% CO2 at 37℃. A day before transfection, cells were plated 4×106 cells in a 6 well plate. Then cells were transfected with 10µg plasmid cDNAs using the calcium phosphate precipitation method. After 24 hr, cells were harvested for the next experiments. For immunofluorescence analysis, cells were washed, permeabilized with 0.1% Triton X-100, and incubated with PBS/3% BSA. Then cells were stained with FITC-conjugated anti-Myc tag antibody (Abcam, Cambridge, UK). After washing, coverslips were mounted on slides and confocal images were obtained with a Zeiss Axioplan photomicroscope and Zess LSM510 (Zeiss, Hamburg, Germany).
Human multiple tissue Northern blot (Clontech) was used for analyzing the expression pattern of the CD320 gene. To analyze mouse NG29 gene expression, total RNA was prepared from different tissues or cell lines, loaded and subjected to the probe hybridization. For probes, the CD320 and NG29 cDNAs were radiolabelled and hybridized with the blots essentially according to the manufacturer's protocol (MTN®, Clontech).
To obtain the probe for the human CD320 gene expression, various human cell lines were tested for the expression of CD320 by RT-PCR using a pair of oligonucleotide primers 5'-TTATGCGTGCCCCTCACC-3' and 5'-CGAGCTCGTCGCTGGAGTC-3', which encompass the partial sequence of human CD320 cDNA. The RT-PCR product obtained from HEK-293T cells were cloned into pTBlue-T vector (Novagen, Madison, WI) and labelled for the northern blot analysis.
HEK-293T cells were transfected with the NG29ext-NusA expression vector. 48 hours after transfection, the cell growth media was harvested and diluted 10-fold in PBS. Then, one-step Ni-NTA (Qiagen, Hilden, Germany) affinity chromatography was performed as previously described (16). The diluents were loaded onto pre-equilibrated Ni-NTA affinity column and washed with 20 mM pH 8.0 Tris buffer containing 300 mM NaCl. The fusion proteins were eluted with a linear gradient of 100~350 mM imidazole in the same buffer. The fusion protein-containing fractions were confirmed by SDS-PAGE and pooled.
The purified protein was used for immunization into rabbit and rabbit immunization was performed following standard protocols. After immunization, the serum was obtained and used for immunopreciptation and immunoblotting.
For immunoprecipitation, cells were lysed with NP40 lysis buffer (17) and the lysate was incubated with anti-Myc tag (Upstate Biotechnology, Lake Placid, NY) or anti-NG29 antibody for 2 hours. The antibody complexes were isolated with protein A-agarose beads (Pharmacia Biotech, Uppsala, Sweden) and washed with the lysate buffer four times. Then, the immunocomplexes were subjected to SDS-PAGE and immunoblotting with anti-Myc tag or anti-NG29 antibody. Protein bands were visualized by exposure of the membrane to autoradiographic film (Kodak, Rochester, NY). For the de-glycosylation assay, cell growth media was incubated in 1X glycosylation denaturing buffer (New England Biolabs, Beverly, MA) at 100℃ for 10 min. Then, 0.1 volume of G7 buffer, 10% NP40, and 2 µl of PNGase F (New England Biolabs) were added and incubated for 1 hr at 37℃. Then, the lysate was immunoprecipitated and analyzed.
Raw 264.7 cells were washed with the flow cytometry staining medium (PBS, 2% BSA, and 0.2% NaN3) and 1×106 cells were then incubated with 1µl rabbit anti-NG29 serum that we produced previously. Then cells were incubated with FITC- conjugated anti-rabbit immunoglobulin Ab (Zymed, South San Francisco, CA). Data were collected with a flow cytometry (FACS Calibur, Becton Dickinson, San Jose, CA) and analyzed with Cell Quest Analysis Software.
In order to identify new proteins of immunological interest, we searched for genes in the murine MHC region, which encode transmembrane proteins that may function in intercellular interactions between immune cells. We identified the NG29 gene, which encodes a putative transmembrane protein partly similar to the LDL receptor family member within a sequence data under the GeneBank accession number AF110520. It was found to be located in the region that is centromeric to the extended MHC region, which corresponds to the extended MHC class II region in human (3). Its location was just centromeric to Hset gene, the most centromerically located gene of the extended MHC region. By a homology search, the NG29 gene was found to be homologous to the human CD320/8D6A gene, which encodes a novel human follicular dendritic cell antigen, 8D6, as reported by Choi et al. (14), but the gene for human CD320/8D6A was located in the chromosome 19p, not in the human MHC region. However, the synteny of NG29 gene with genes for NADH dehydrogenase, ribosomal protein S28, and NG28 was conserved in human chromosome 19p13.2-3, which suggests that mouse NG29 and human CD320/8D6A genes may have originated from a common ancestral gene.
We designed specific primers corresponding to the putative start and stop translation codons to obtain NG29 cDNA sequence by RT-PCR. A major band of approximately 800 bp was isolated from cDNA of C57BL/6 mouse kidney and its sequence was confirmed. The amplified product revealed an ORF of 780 bp encoding a protein of 260 amino acid residues. Comparison of the predicted amino acid sequences of NG29 and 8D6A genes is presented in Fig. 1A. The predicted NG29 protein consists of a leader peptide (29 amino acid residues), an extracellular region with two LDL receptor-like domains, a transmembrane region (17 amino acid residues), and a cytoplasmic tail of 33 residues. Comparison of two sequences showed an amino acid identify of 65%.
Based on the NG29 cDNA sequence we obtained, we determined the genomic organization of the NG29 gene. Based on the cDNA sequence, the NG29 gene was supposed to be composed of five exons, as the predicted coding sequence of NG29 within the genomic sequence of the extended MHC region in the Genebank accession number AF110520 (Fig. 1B). All exon-intron boundary sequences conformed to the GT-AG rule and surrounding nucleotides closely related to consensus sequences found near splice junctions. This overall genomic structure of NG29 gene was similar to that of human CD320/8D6 gene, which is also composed of five exons. The lengths of introns between exons were similar between the human and mouse genes.
The amino acid sequence of the predicted NG29 protein was shown and aligned with that of human CD320 protein to compare the sequence similarity between two proteins (Fig. 1A). A few strings of amino acid sequences were more conserved than other areas between the two. Two shaded conserved sequences indicate the LDL receptor-like domains and the C-terminal region also showed a high similarity. The two human and mouse proteins were similar in the domain structure and the relative locations of exon-intron boundaries. The first exon consisted of untranslated 5' leader, signal sequences, and N-terminal portion of the predicted transmembrane protein. The second and third exons contained one of two LDL receptor-like domains (Fig. 1A, grey boxes), respectively, and the putative transmembrane (Fig. 1A, underlined) and cytoplasmic domains were correspondent to the fifth exon. These similarities in the genomic structure and the predicted protein domain structure suggest that the NG29 gene is an orthologue of the human CD320/8D6 gene.
To investigate the expression pattern of the mouse NG29 gene, we performed a northern blot analysis of different mouse tissues and cell lines by using the labelled NG29 cDNA as a probe. Differently from our expectation that the NG29 expression would be in lymphoid tissues, the spleen showed almost no expression of NG29 (Fig. 2A). In contrast, NG29 was highly expressed in the kidney and cell lines such as NIH3T3 and Raw264.7 cells. Mouse embryonic fibroblasts also showed a high expression of NG29. This finding led us to check the expression of human CD320/8D6 gene in various types of tissues. We prepared the CD320 cDNA probe by using the full length cDNA construct that had been cloned as described in Materials and Methods, and then performed a northern blot hybridization using human multiple tissue Northern blot. The CD320/8D6 gene expression was detected strongly in the placenta, heart, muscle, spleen and kidney. Colon, liver, lung and small intestine expressed the transcript in a low level (Fig. 2B). These results showed that the human and mouse CD320 genes were not highly specific to follicular dendritic cells, but also strongly expressed in the non-lymphoid organs such as kidney and placenta and macrophage cell lines.
Next we determined the subcellular localization of the enforcedly expressed NG29 protein in HEK-293T cells that had been transiently expressed with NG29 expression constructs. To this end, we made two constructs containing the full coding sequence of NG29 fused with EGFP (NG29-EGFP) or with myc (NG29-Myc). HEK-293T cells were transfected with the NG29-EGFP construct and cells were visualized under the fluorescence microscope 24 hours after the transfection (Fig. 3A). HEK-293T cells transfected with the NG29-myc construct were stained with FITC-conjugated anti-myc antibody 24 hours after transfection and observed (Fig. 3B). NG29-GFP fusion proteins were found be localized in the cytoplasm and plasma membrane. Large and small green fluorescence spots were scattered in the cytoplasm. A smooth membranous staining of anti-myc antibody was detected in HEK-293T cells transfected with NG29-myc (Fig. 3B). These transfection and intracellular localization results suggested that NG29 protein was a transmembrane protein localized on the plasma membrane and the intracellular vesicles.
We developed anti-NG29 polyclonal antibodies to elucidate the biochemical characteristics of the NG29 protein. To generate rabbit polyclonal antibody, we prepared an expression vector (NG29ext-NusA) that encoded the extracellular domain of NG29 fused with the NusA protein as described in Materials and Methods. HEK-293T cells transfected with the NG29ext-NusA construct were checked for the protein expression by immunoblotting with anti-myc since myc-tag was inserted in the NG29ext-NusA construct. The fusion proteins were found to be both in the culture supernatant and the cell lysate (Fig. 4). The NG29-NusA protein was confirmed to be a glycoprotein since the size of the fusion protein was reduced by treatment with PNGase F, an amidase that cleaves between the innermost GlcNAc and asparagine residues of high mannose. It appeared that the secreted fusion protein were more heavily glycosylated than the fusion protein retained in the cell. The fusion protein was purified from the supernatant of NG29-NusA-transfected HEK-293T cell culture and used for the immunization into rabbits. The resultant serum was used for the immunoprecipitation and flow cytometry study.
Then, the apparent molecular weight of the NG29 protein was determined by using HEK-293T cells transfected with the NG29-myc expression construct. The transfected cells were lysed and the lysate was immunoprecipitated with anti-NG29 antibody that we generated. Then, the immunoblotting was performed with the anti-myc antibody. The major band of the NG29 protein was found to be of 45 kDa and the molecular weight was reduced to about 43 kDa after PNGase F treatment (Fig. 5). To further confirm whether the NG29 protein is expressed on the cell surface, we performed a flow cytometric analysis in Raw264.7 cells by using the anti-NG29 polyclonal antibody and could find that the anti-NG29 antibody labelled Raw264.7 cells (Fig. 6). These results suggested that the NG29 protein was expressed as a glycosylated transmembrane cell surface protein.
In this study, we have isolated a novel murine gene, NG29, in the extended MHC region and confirmed its expression as mRNA as well as protein. The NG29 gene was predicted be an an ortholog to the human CD320/8D6A gene based on their sequence similarity, but its characterization was not reported so far. Overall, there is 64.8% nucleotide identity between mouse NG29 and human CD320/8D6A genes within the ORF nucleotide sequences. There is also 55.9% amino acid identity and 62.9% similarity between predicted NG29 and human CD320 protein sequences. In particular, the extracellular region of both proteins contains two domains homologous to the type A cysteine-rich repeats found in the low density lipoprotein receptor (LDL-R) family and several other proteins, including the terminal complement components such as C8 and C9 (18,19). Both cysteine-rich repeat domains of the NG29 protein contains the six cysteine residues and the acidic amino acid residues between the fifth and sixth cysteines, which characterizes the domain. The first cysteine-rich repeat domain of the NG29 protein has 80% identity to that of the human CD320 and 44% identity to that of the NH2-terminal domain of the human LDL-R, whereas the second domain of the NG29 protein has 72% identity to that of the human CD320 and 41% identity to that of the LDL-R. In addition, the homology between the NG29 and human CD320 protein was also strong in the C-terminal region representing the transmembrane domain and cytoplasmic tail. The hydropathy plot of the NG29 protein predicted a putative trasnmembrane region from the stretch of amino acid residues 210-226, and this region was highly matched with that of human CD320 (82% of identity). The cytoplasmic domain also showed a strong similarity, suggesting that the two proteins might use the same kinds of signaling pathways.
The LDL-R is essential for the transport LDL out of plasma into tissues. However, Bar et al. demonstrated that most of the LDL-R superfamily do not have a primary function in the LDL import, but binds multiple ligands and have roles in motility and adhesions of neurons (20). For example, very low-density lipoprotein receptor (VLDL-R) is involved in neuron traffic in brain development, as demonstrated in VLDL-R-deficient mice (21). It is intriguing to find that the human and mouse CD320 molecules share a significant homology with LDL-R since these molecules may also be implicated in the cell-cell interactions. The human CD320 molecule, originally named as 8D6 by use of a monoclonal antibody, was shown to be expressed abundantly on follicular dendritic cells (FDCs). Monoclonal antibody 8D6 against CD320 blocked the plasma cell generation and IgG secretion, but not memory B cell proliferation, which indicated that FDC signaling molecule CD32/08D6 augments plasma cell generation in the germinal center (15). Hence, it is important to investigate whether the NG29 molecule expressed in stromal cells is involved in attracting certain types of cells, especially B cells and other hematopoietic cells. So far the ligands for the human and mouse CD320 are not known and need to be elucidated.
Similarly to the broad expression of CD320 (14), the NG29 gene was shown to be expressed in various tissues and cell lines tested. The expression level was particularly high in the kidney and several types of cell lines such as Raw264.7 and NIH-3T3, which had been originated from macrophage and fibroblast, respectively. In contrast to human CD320, the NG29 gene expression was barely detected in mouse B cells and spleen. We think that it is needed to check whether the NG29 gene expression is regulated by cell activation or tumorigenesis. Especially, the high expression of the NG29 gene in kidney, which is a target organ of many kinds of immunological disorders compared with other organs, may reflect a possible role of this molecule during immunological response in those disease processes. Functional roles of conserved cysteine-rich repeat domains of NG29 and CD320 are not known, which would be clearer when the ligands be elucidated, but the highly conserved nature of two domains between the human and mouse CD320 suggests that these domains may interact some evolutionarily shared ligand molecule( s). Furthermore, the highly conserved cytoplasmic tail sequence indicates that the two molecules may bind the same kinds of signaling molecules and trigger some kinds of conserved functions.
Additionally, we confirmed that NG29 is a glycosylated transmembrane protein as predicted by the gene analysis. The full-length NG29 protein tagged with EGFP or myc was localized in plama membrane and cytoplasm, which suggests that it is a transmembrane protein that is retained in the cytoplasmic vesicles or recycling to the intracytoplasmic compartment. The nature of the NG29 protein as a glycoprotein was further confirmed by the immunoblotting study after the glycosylase treatment. The size of immunoprecipitated NG29 protein was reduced by ~2 kDa by the pretreatment of the immunoprecipitants with PNGase F. Glycosylation is one of the most common post-translational modifications, and approximately 50% of all proteins are presumed to be glycosylated in eukaryotes (22). It is well known that glycosylation is involved in a variety of physiological and pathological events, including cell growth, migration, differentiation, adhesion, and so on (23,24). Since NG29 protein is highly expressed in stromal cells, glycosylation may play a role in the cell-cell or cell-stromal molecule adhesion through NG29.
By virtue of overwhelming evidences for the genetic linkage conservation among mammalian and vertebrate species, the mapping of conserved chromosomal positions may now constitute one of important criteria for orthology. Although the comparison of genomic organizations and amino acid sequences provide a strong evidence that murine NG29 is an ortholog of human CD320/8D6, their locations on chromosome are unexpectedly distinctive; the NG29 gene is located in the centrometrically extended MHC region of chromosome 17 whereas the CD320/8D6 gene is located in a non-MHC region, chromosome 19p13. Kasagara et al. and Katsanis et al. found independently that the human chromosome 6p21.3 and 9p33-q34 regions are paralogous and pointed out that two additional regions in the human genome, 19p13.1-p13.3 and 1q21-q25, contain a cluster of MHC-related genes (25-27). These observations strongly suggest that the four regions in the human chromosome, which are MHC, 9p33-q34, 1q21-25, and 19p13.1-p13.3, have been descended from a common ancestral region and have diverged by chromosomal duplications. In addition, the majority of the human paralogs residing on 19p13.1-p13.3 have corresponding copies in the region telomeric to the MHC on mouse chromosome 17. Although the NG29 gene is located in the centrometrically extended MHC region of mouse chromosome 17, data presented by this study showed an indisputable evidence that the NG29 gene is an ortholog of CD320/8D6.
In summary, the NG29 gene, the muring ortholog of the human CD320/8D6 gene, was firstly characterized in this study. The gene was confirmed to encode a glycosylated transmembrane protein expressed in various stromal cells and tissues. Further studies including more detailed biochemical and molecular characterization and functional analyses in in vivo mouse models such as knockout or transgenic mice are required to elucidate its functional role in B cell immune responses and unknown roles in kidney and other tissues.
Figures and Tables
Figure 1
Comparison of mouse NG29 and human CD320/8D6A genes (Genebank accession number AF110520 and NM_016579, respectively). (A) Comparison of predicted amino acid sequences. Predicted transmembrane domains are underlined and all exon-intron boundaries are indicated as arrows. Shaded boxes are two LDL receptor-like domains. Putative signal sequences are indicated by lowercase letters. (B) Comparison of genomic structures of NG29 and 8D6A genes. The location of the coding regions is depicted by closed boxes. Introns are shown as horizontal lines ranging from 0.1 to 3 kb. The genomic organizations of both genes were deduced from the mouse and the human genomic clones with accession numbers AF110520 and NT_077812, respectively. 5'UT, 5' untranslated; LDLRD1 & 2, LDL receptor domains 1 & 2; int 1 & 2, intervening sequences 1 & 2; TM, transmembrane; cyto, cytoplasmic.
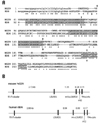
Figure 2
Analysis of the expression of mouse NG29 and CD320/8D6 genes. (A) Northern blot analysis of RNA samples from indicated murine tissues and cell lines. Raw, Raw264.7; 293T, HEK-293T; MEF, mouse embryonic fibroblast). COS-7 cells are from monkey and HEK-293T cells are from human. Upper: hybridization bands, Lower: 28S ribosomal RNA bands. (B) Expression of CD320 mRNA in human tissues. Human tissue blot (Clontech) was hybridized with human CD320 cDNA probe. S Intest, small intestine; PBL, peripheral blood leukocytes.
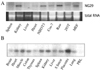
Figure 3
Immunofluorescent localization of mouse NG29 gene expression. HEK-293T cells were transfected with NG29-pEGFP-N3 plasmid in (A) or NG29-pcDNA3.1/myc plasmid in (B). 2 days after transfection, cells were observed under the Zeiss inverted confocal fluorescence microscopy. In (B), cells were stained with FITC-conjugated anti-Myc tag antibody. The size bar indicates 10 µm.
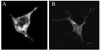
Figure 4
Production of NG29-NusA fusion protein. HEK-293T cells were transfected with the the NG29ext-NusA expression construct. The fusion protein was purified from the culture supernatant and cell lysate as described in Materials and Methods. Purified proteins were treated with or without PNGase F and immunoblotting was performed with anti-Myc tag antibody.
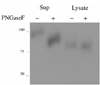
Figure 5
Immunoprecipitation of the NG29 protein in HEK-293T cells transfected with the NG29 expression construct. HEK-293T cells were transfected with the construct and cell lysate was immunoprecipitated with the serum we generated earlier. The immunoprecipitates was treated or not treated PNGase F and then immunoblotted with anti-Myc tag antibody. Mark indicates the size of 50 kDa.
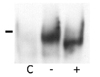
ACKNOWLEDGEMENTS
This work was supported by a grant of the Korea Health 21 R & D Project, Ministry of Health & Welfare, Republic of Korea (01-PJ3-PG6-01GN12-0001).
References
1. Amadou C, Kumánovics A, Jones EP, Lambracht-Washington D, Yoshino M, Lindahl KF. The mouse major histocompatibility complex: some assembly required. Immunol Rev. 1999. 167:211–221.


2. Yeager M, Hughes AL. Evolution of the mammalian MHC: natural selection, recombination, and convergent evolution. Immunol Rev. 1999. 167:45–58.


3. Stephens R, Horton R, Humphray S, Rowen L, Trowsdale J, Beck S. Gene organisation, sequence variation and isochore structure at the centromeric boundary of the human MHC. J Mol Biol. 1999. 291:789–799.


4. Gruen JR, Weissman SM. Evolving views of the major histocompatibility complex. Blood. 1997. 90:4252–4265.


5. Complete sequence and gene map of a human major histocompatibility complex. The MHC sequencing consortium. Nature. 1999. 401:921–923.
7. Cupedo T, Kraal G, Mebius RE. The role of CD45+CD4+CD3- cells in lymphoid organ development. Immunol Rev. 2002. 189:41–50.
8. Anderson G, Jenkinson EJ. Lymphostromal interactions in thymic development and function. Nat Rev Immunol. 2001. 1:31–40.


9. Li L, Choi Y. Follicular dendritic cell-signaling molecules required for proliferation and differentiation of GC-B cells. Semin Immunol. 2002. 14:259–266.


10. Katakai T, Hara T, Sugai M, Gonda H, Shimizu A. Lymph node fibroblastic reticular cells construct the stromal reticulum via contact with lymphocytes. J Exp Med. 2004. 200:783–795.


11. Gretz JE, Anderson AO, Shaw S. Cords, channels, corridors and conduits: critical architectural elements facilitating cell interactions in the lymph node cortex. Immunol Rev. 1997. 156:11–24.


12. Bofill M, Akbar AN, Amlot PL. Follicular dendritic cells share a membrane-bound protein with fibroblasts. J Pathol. 2000. 191:217–226.


13. Balogh P, Horváth G, Szakal AK. Immunoarchitecture of distinct reticular fibroblastic domains in the white pulp of mouse spleen. J Histochem Cytochem. 2004. 52:1287–1298.


14. Li L, Zhang X, Kovacic S, Long AJ, Bourque K, Wood CR, Choi YS. Identification of a human follicular dendritic cell molecule that stimulates germinal center B cell growth. J Exp Med. 2000. 191:1077–1084.


15. Zhang X, Li L, Jung J, Xiang S, Hollmann C, Choi YS. The distinct roles of T cell-derived cytokines and a novel follicular dendritic cell-signaling molecule 8D6 in germinal center-B cell differentiation. J Immunol. 2001. 167:49–56.


16. Kim JY, Lee MH, Jung KI, Na HY, Cha HS, Ko EM, Kim TJ. Detection of antibodies against glucose 6-phosphate isomerase in synovial fluid of rheumatoid arthritis using surface plasmon resonance (BIAcore). Exp Mol Med. 2003. 35:310–316.


17. Hahn MJ, Yoon SS, Sohn HW, Song HG, Park SH, Kim TJ. Differential activation of MAP kinase family members triggered by CD99 engagement. FEBS Lett. 2000. 470:350–354.


18. Herz J, Hamann U, Rogne S, Myklebost O, Gausepohl H, Stanley KK. Surface location and high affinity for calcium of a 500-kd liver membrane protein closely related to the LDL-receptor suggest a physiological role as lipoprotein receptor. EMBO J. 1988. 7:4119–4127.


19. Krieger M, Herz J. Structures and functions of multiligand lipoprotein receptors: macrophage scavenger receptors and LDL receptor-related protein (LRP). Annu Rev Biochem. 1994. 63:601–637.


20. Bar I, Goffinet AM. Developmental neurobiology. Decoding the Reelin signal. Nature. 1999. 399:645–646.
21. Trommsdorff M, Gotthardt M, Hiesberger T, Shelton J, Stockinger W, Nimpf J, Hammer RE, Richardson JA, Herz J. Reeler/Disabled-like disruption of neuronal migration in knockout mice lacking the VLDL receptor and ApoE receptor 2. Cell. 1999. 97:689–701.


22. Apweiler R, Hermjakob H, Sharon N. On the frequency of protein glycosylation, as deduced from analysis of the SWISS-PROT database. Biochim Biophys Acta. 1999. 1473:4–8.


23. Earl LA, Baum LG. CD45 glycosylation controls T-cell life and death. Immunol Cell Biol. 2008. 86:608–615.


24. Zhao Y, Sato Y, Isaji T, Fukuda T, Matsumoto A, Miyoshi E, Gu J, Taniguchi N. Branched N-glycans regulate the biological functions of integrins and cadherins. FEBS J. 2008. 275:1939–1948.


25. Kasahara M, Hayashi M, Tanaka K, Inoko H, Sugaya K, Ikemura T, Ishibashi T. Chromosomal localization of the proteasome Z subunit gene reveals an ancient chromosomal duplication involving the major histocompatibility complex. Proc Natl Acad Sci U S A. 1996. 93:9096–9101.

