Abstract
11β-hydroxysteroid dehydrogenase 1 (11β-HSD1) catalyzes the conversion of inactive cortisone into active cortisol, which has pleiotropic roles in various biological conditions, such as immunological and metabolic homeostasis. Cortisol is mainly produced in the adrenal gland, but can be locally regenerated in the liver, fat, and muscle. Its diverse actions are primarily mediated by binding to the glucocorticoid receptor. SW982, a human synovial cell line, expresses 11β-HSD type 1, but not type 2, that catalyzes the conversion of cortisone to cortisol. In this study, therefore, we investigated the control of lipopolysaccharide (LPS)-induced inflammatory responses by prereceptor regulation-mediated maintenance of cortisol levels. Preliminarily, cell seeding density and incubation period were optimized for analyzing the catalytic activity of SW982. Additionally, cellular 11β-HSD1 still remained active irrespective of monolayer or spheroid culture conditions. Inflammatory stimulants, such as interleukin (IL)-1β, tumor necrosis factor (TNF)α, and LPS, did not affect the catalytic activity of 11β-HSD1, although a high dose of LPS significantly decreased its activity. Additionally, autocrine effects of cortisol on inflammatory responses were investigated in LPS-stimulated SW982 cells. LPS upregulated pro-inflammatory cytokines, including IL-6 and IL-1β, in SW982 cells, while cortisol production, catalyzed by cellular 11β-HSD1, downregulated LPS-stimulated cytokines. Furthermore, suppression of NFκB activation-mediated pro-inflammatory responses by cortisol was revealed. In conclusion, the activity of cellular 11β-HSD1 was closely correlated with suppression of LPS-induced inflammation. Therefore, these results partly support the notion that prereceptor regulation of locally regenerated cortisol could be taken into consideration for treatment of inflammation-associated diseases, including arthritis.
Cortisol is a glucocorticoid hormone (GH) mainly produced in the adrenal gland and is tightly regulated via the hypothalamic-pituitary-adrenal (HPA) axis (1). Besides, it can be converted from its substrate cortisone by the action of 11β-hydroxysteroid dehydrogenase (11β-HSD1) in the peripheral tissues, including the liver, fat, brain, and skeletal muscle (2345). Physiologically, cortisol is a biologically active GH found in humans, and exerts pleiotropic effects by binding to the glucocorticoid receptor (GR) (67). Blood cortisol levels are closely associated with the development of diseases, such as Cushing's syndrome and Addison's disease (89). It has also been reported that dysregulation of cortisol is closely linked to metabolic syndromes, including type 2 diabetes and obesity. On the contrary, cortisol exhibits a potent anti-inflammatory activity within the normal range, although the precise mechanisms remain unclear. In fact, cortisol acts as a regulator of lymphocytes, which are recruited to the injury sites to trigger inflammation (10). It can be mechanistically proposed that immunosuppressive or anti-inflammatory effects of cortisol are accomplished by blocking inflammatory signals from the transcription factors, especially nuclear factor-κB (NFκB) and AP-1 (11).
Rheumatoid arthritis (RA) is characterized by several changes, including synovial hyperplasia, inflamed synovial membrane, leukocyte recruitment, and cytokine overproduction, resulting in the formation of pannus—abnormal layers of granulation tissue in which massive inflammatory signals occur. Synovial membrane consists of macrophage-like synovial cells and fibroblast-like synoviocytes (FLS), which are involved in maintenance of the internal joint homeostasis. In particular, FLS play key roles in the pathogenesis of RA by producing various chemokines and matrix metalloproteinases (MMPs) (12). A human synovial cell line, SW982, was used for investigating the anti-inflammatory effects of GH. SW982 cells were previously reported to express 11β-HSD1, but not 11β-HSD2 (13), that can catalyze its substrate cortisone into cortisol. Accordingly, this study aimed to investigate the regulation of the inflammatory responses of synovial cells to external stimuli by autocrine glucocorticoids (GCs).
Primarily, we optimized various conditions, such as substrate concentration, cell seeding density, and incubation period that would affect the conversion of inactive substrate to active product in SW982 cells. SW982 cells can be grown in monolayer or spheroid conditions that simulate inflamed synovium. However, there was a slight difference between the 11β-HSD1 activity of the monolayer culture and that of the spheroid culture. To examine the effects of 11β-HSD1 on inflammatory responses, monolayer culture of SW982 was stimulated with lipopolysaccharide (LPS) with or without cortisone. Cortisol produced by cellular 11β-HSD1 downregulated LPS-mediated cytokines, including interleukin (IL)-β and IL-6. Treatment of cells with 11β-HSD1 inhibitor or GH antagonist reversed anti-inflammatory effects of cortisol, suggesting that its mode of action is closely correlated to prereceptor regulation. Furthermore, we suggested that cortisol could inactivate LPS-mediated NFκB activation via regulation of IκB phosphorylation. In summary, locally regenerated cortisol level might alleviate various inflammatory genes under NFκB regulation, highlighting that prereceptor regulation of 11β-HSD1 is significant in the pathogenesis of inflammation-associated diseases.
Cortisone, cortisol, carbenoxolone (CBX), and 18β-glycyrrhetinic acid (GA) were obtained from Sigma (St Louis, MO). Enhancer spray, and [3H] cortisone and cortisol as radioactive substrate and product were obtained from Amersham Life Sciences Ltd. (Piscataway, NJ). HTRF® cortisol assay product was obtained from Nihon Schering K.K. (Tokyo, Japan). EcoDye DNA staining solution was purchased from Biofact (Daejeon, South Korea). IκB and phosphor-IκB were purchased from Cell Signaling Technology (Danvers, MA). All other chemicals and reagents were purchased from Sigma-Aldrich.
Cell line SW982 (ATCC:HTB-93) was purchased from ATCC and maintained in Dulbecco's modified Eagle medium (DMEM) supplemented with antibiotics and 10% fetal bovine serum (FBS). Conventionally, cells were seeded into tissue culture plates and incubated until attachment to plate base. To simulate pannus condition, cells were plated at a high density into each well of 96-well plate, the bottoms of which were not pretreated, and incubated for at least 4 days.
To verify the catalytic conversion from cortisone to cortisol, enzyme activity was also measured by conversion of radioactive cortisone into cortisol on TLC [8]. [3H] Cortisone (160 nM, specific activity: 42.0 Ci/mmol) was added to SW982 culture (500 µL) grown in 24-well plates and incubated for given times. Cortisone metabolites present in culture medium were extracted with ethyl acetate and separated by thin layer chromatography (TLC) using chloroform:methanol (9:1, v/v) as development solution and then visualized by autoradiography of the plate sprayed with enhancer on film. In contrast, non-radioactive assay was carried out by HTRF® protocol. Briefly, cells were seeded onto 24-well plates at different densities and were incubated in 500 µL DMEM containing 160 nM cortisone. Culture media (2 µL) were removed at the indicated time points, and enzyme activity was assessed by HTRF® assay as carried out previously (14).
SW982 cells were stimulated with LPS and then the level of its downstream inflammatory cytokines were monitored and correlated with the levels of 11β-HSD1 expression. RNAs extracted from SW982 cells were amplified by reverse transcription-polymerase chain reaction (RT-PCR) using specific primers described below. Specific primers for IL-1β, IL-6, 11β-HSD1, and β-actin were designed for amplification of these genes. Sequences for those genes are as follow. S or AS in parentheses indicates sense or antisense primer, respectively.
IL-6: 5′-ATG AAC TCC TTC TCC ACA AGC GC (S)-3′, 5′-GAA GAG CCC TCA GGC TGG ACT G-3′ (AS)
IL-β: 5′-AAA CAG ATG AAG TGC TCC TTC CAG G-3′(S), 5′-TGG AGA ACA CCA CTT GTT GCT CCA-3′ (AS)
11β-HSD1: 5′-TTG CTT TGG ATG GGT TCT TC-3′ (S), 5′-AGA GCT CCC CCT TTG ATG AT-3′ (AS)
β-actin: 5′-GCC ATG TAC GTT GCT ATC-3′ (S), 5′-CTC CTT AAT GTC ACG CAC-3′(AS)
Aliquots of the PCR products were electrophoresed on 1.2% agarose gel, followed by visualization using EcoDye staining solution, and gel images were acquired with a UV trans-illuminator. To investigate the effects of LPS alone or LPS with cortisone on inflammatory cytokines, RNA samples were extracted from cells treated with LPS in the presence or absence of cortisone and subjected to RT-PCR using specific primers for IL-6 and IL-1β.
NFκB activity was indirectly determined by measuring the levels of phosphor-IκB. The lysates prepared from SW982 cells were subjected to SDS-PAGE and transferred onto nitrocellulose (NC) membrane. Resolved proteins on NC were probed with an antibody against phosphor-IκB, and then further incubated with a secondary antibody conjugated with horseradish peroxidase to visualize its target proteins.
The catalytic activity of cellular 11β-HSD1 in SW982 cells to convert cortisone into cortisol was examined on TLC. Incubation of SW982 cells with cortisone as a substrate could convert cortisone into cortisol in a cell density dependent manner as demonstrated by slower migration of products than reactants on a TLC plate (Fig. 1A). HTRF assay, a non-radioactive method, was employed to detect cellular 11β-HSD1 activity. As shown in Fig. 1B, conversion of cortisone into cortisol was proportional to cell numbers plated, indicating that cells at a density of 106 converted most of the cortisone within 48 h. Moreover, cortisone was converted into cortisol in cells in a time dependent manner. To ascertain the involvement of cellular 11β-HSD1 in cortisone/cortisol conversion, 11β-HSD inhibitors were used. Treatment of cells with either GA or CBX substantially inhibited cortisol production, suggesting that cellular 11β-HSD1 is responsible for catalytic synthesis of cortisol (Fig. 1C).
SW982 cells can be grown in two different conditions—monolayer and spheroid cultures (Fig. 2A). Microscopic observation revealed that spheroid culture induced conglomeration of cells within a limited area. 11β-HSD1 activity of cells grown under these culture conditions was compared using the HTRF assay, and a slight difference in the cortisol production was noted between monolayer and spheroid cultures (Fig. 2B).
The catalytic activity of 11β-HSD1 was monitored after SW982 cells were stimulated with pro-inflammatory cytokines or LPS (Fig. 3). Cells treated with IL-1β, TNFα, or LPS (concentrations lower than 100 ng/mL) did not substantially affect 11β-HSD1 activity, while those treated with LPS at a concentration of 100 ng/mL significantly reduced its activity by 30%.
As mentioned previously, expression levels of cytokines after treatment of cells with LPS were preliminarily evaluated by RT-PCR (Fig. 4). Stimulation of cells with LPS induced pro-inflammatory cytokines, IL-1β and IL-6. Addition of cortisone into SW982 culture suppressed LPS-induced inflammatory responses. Conversely, either GA or RU486 reversed the anti-inflammatory effects of cortisone against LPS stimulation.
It has been known that LPS induces various inflammatory cytokines via NFκB activation, and that cortisol has a negative effect on LPS-stimulated inflammation via its cognate receptor. To better understand the mechanism underlying suppression of various inflammatory cytokines by active cortisol, NFκB activity was investigated following LPS stimulation in the absence or presence of cortisone (Fig. 5). NFκB activation was indirectly evaluated by phosphor-IκB levels in SW982 cells. Cells showed NFκB activation in response to LPS, while LPS-induced NFκB activation was suppressed in a dose-dependent manner.
Synovial cells, SW982, express 11β-HSD type 1, but not type 2, that catalyzes the conversion of cortisone to cortisol. The principal role of cortisol is to increase glucose level by gluconeogenesis and carbohydrate metabolism (1516). Additionally, cortisol can be engaged in the suppression of acute immune responses and in the reduction of bone formation (17). Specifically, numerous lines of evidence suggest that inflammatory activation of synovial cells has been decisive in the pathogenesis of degenerative arthritis. Therefore, this study reveals the mechanism underlying regulation of the LPS-induced inflammatory responses by 11β-HSD activity. Autocrine cortisol significantly suppressed various LPS-stimulated responses, indicating that 11β-HSD might effectively regulate the local inflammatory responses.
Since GCs activate the negative feedback circuit of inflammation, they are widely prescribed in medicine to treat various diseases, such as allergies, asthma, autoimmune diseases, and sepsis (18192021). Furthermore, GC at high doses can reject the abnormal cancer cells helping towards treating cancers. However, their overuse should be restricted because of diverse pleiotropic effects on metabolic homeostasis as well as the immune system. Mechanistically, GCs exhibit their effects by binding to the GR, not only by trans-activating anti-inflammatory proteins, but also by trans-repressing pro-inflammatory proteins, to downregulate inflammation (22). In fact, an active GC cortisol inhibits T-cell proliferation by desensitizing T-cells to IL-1 and suppressing synthesis of T-cell growth factor (23). Moreover, cortisol synthesis in the epidermis exhibits a negative feedback effect on inflammatory cytokine IL-1 upon tissue injury (24).
Previously, it was reported that SW982 cells were phenotypically characterized after stimulation with IL-1β in the presence of dexamethasone (25). Dexamethasone effectively downregulates IL-1β, IL-6, and COX-2 transcripts at high cell density, but not at low cell density. To examine the anti-inflammatory role of locally regenerated cortisol, in this study, SW982 cells were stimulated with LPS in the presence or absence of cortisone. The conversion reaction between cortisone and cortisol was catalyzed in the forward and reverse directions by 11β-HSD1 and 11β-HSD2, respectively, but the latter was a more potent GC. It has been already reported that SW982 cells express 11β-HSD1, but not 11β-HSD2. 11β-HSD1 in SW982 cells catalyzes conversion of cortisone into cortisol, an active GH. Its cellular conversion was verified from TLC analysis of radioactive products present in culture media of SW982 cells (Fig. 1). The use of radioactive cortisone for TLC analysis was time-consuming and labor-intensive; therefore, a non-radioactive method was adopted to monitor the levels of cortisol, without its purification. An increase in cortisol production corresponding to the cell seeding density was comparable to values obtained from the HTRF assay, which depends on the use of an antibody specific towards cortisol. Cells were further optimized for incubation period and substrate concentration required to convert cortisone into cortisol during culture. The catalytic requirement of 11β-HSD1 for metabolic conversion was appropriately proved by the fact that cortisol synthesis was inhibited by an inhibitor of 11b-HSD1, CBX or GA. Notably, SW982 cells were grown in two different culture conditions—monolayer and spheroid cultures. Unlike monolayer culture of SW982 cells, spheroid culture mimics active FLS in the pannus of patients with RA. Since there was no significant difference in 11β-HSD1 activity between both the culture conditions (Fig. 3), SW982 cells grown under monolayer condition were treated with cytokines or LPS to provoke an inflammatory response. IL-1β or TNFα did not significantly increase 11β-HSD1 expression, while LPS at a high dose decreased 11β-HSD1 transcript in SW982 cells (Fig. 3). Pro-inflammatory cytokines, such as IL-1α/TNFα, increase 11β-HSD1 in human epithelial cells, A549, via phosphorylation of C/EBPβ (26) and keratinocytes (27). Conversely, it has been reported that 11β-HSD1-catalyzed cortisol production modulates expression of inflammatory cytokines.
LPS induced various inflammatory outcomes similar to those in previous reports, while pretreatment of SW982 cells with cortisone dramatically abrogated such LPS-induced inflammatory responses (Fig. 4). Cortisol is known as a potent anti-inflammatory hormone, and its underlying mechanisms have been proposed. Cortisol treatment suppresses LPS-induced IL-6, but not IL-8, transcripts (28). Generally, its anti-inflammatory potency is attributed to negative transcriptional regulation of the inflammatory gene (29). In fact, cortisol binds to the cognate GR in the cytoplasm to neutralize NFκB, which induces gene expression of inflammation-related proteins and adherence factors (30). Additionally, it partially interferes with a heterodimeric protein, AP-1, composed of Fos and Jun, which can induce transcriptional activation of pro-inflammatory proteins (31). Consequently, cortisol synthesized from cortisone was proposed to reduce LPS-mediated inflammatory responses through NFκB or AP-1 transcriptional regulation.
In summary, cortisol production catalyzed by a cellular enzyme prevents LPS-derived inflammatory response in the cell (Fig. 6). LPS induces pro-inflammatory cytokines by binding to TLR4 and then activating NFκB. Production of autocrine cortisol from cortisone is driven by cellular 11β-HSD1 and resulting locally regenerated cortisol might interfere with NFκB activation directly or indirectly, synergistically suppressing inflammatory responses to LPS. In conclusion, these results highlight that levels of locally regenerated cortisol in synovial cells play a key role in modulating inflammatory response to LPS or cytokines in synovial cells, which could cause pathogenesis of RA.
Figures and Tables
Figure 1
Determination of cortisol production by 11β-HSD 1 activity in SW982 cells. Cortisol was detected by (A) TLC, and (B) HTRF assay using a cortisol-specific antibody. Cells were plated at various densities of 5×104, 1×105, 5×105, 1×106 and incubated with medium containing radioactive cortisone for 48 h. Culture media were subjected to extraction with organic solvent and then TLC development as described in the Materials and Methods section. The ability of cells to convert cortisone/cortisol was further optimized in the HTRF assay by differing cell-seeding density (top of 1B) and incubation times (bottom of 1B). Cells (1×105) were incubated in 160 nM cortisone-containing medium in the presence or absence of 1 µM CBX or GA for 6 h, and then the medium was aliquoted to measure cortisol levels. E and F indicate cortisone and cortisol, respectively.
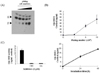
Figure 2
Microscopic evaluation and 11β-HSD 1 activity in cells grown under monolayer or spheroid culture conditions. (A) Cells were grown in monolayer or spheroid conditions and then observed microscopically using 10× ocular lens and 2× objective lens. (B) Cortisol levels in culture medium from SW982 cells grown under different conditions were measured. Cells were incubated in DMEM containing 160 nM cortisone as a substrate of 11β-HSD1.
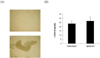
Figure 3
Effects of pro-inflammatory cytokines or LPS on 11β-HSD1 activity. Cells at a density of 106 were stimulated with IL-1β, LPS, or TNFα at the concentrations of 10 and 100 ng/mL for 24 h. Cortisone (160 nM) was added right before stimulation, and then the culture medium was aliquoted to measure conversion of cortisone to cortisol in the HTRF assay. Each bar represents the mean±S.D. of 2 separate experiments. *p<0.05 versus untreated control.
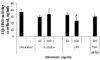
Figure 4
Effect of cortisone/cortisol on LPS-induced inflammatory cytokines in SW982 cells. SW982 cells were stimulated with 10 ng/mL LPS and then the profiles of pro-inflammatory cytokines were primarily investigated. To check the anti-inflammatory effect of cortisol, cells were incubated in DMEM containing cortisone. Pro-inflammatory cytokines, including IL-1β and IL-6, were monitored in cells stimulated with LPS in the presence or absence of cortisone.
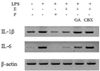
Figure 5
Suppression of LPS-mediated NFκB activation by cortisone. To examine the suppression of LPS-stimulated inflammatory signal by cortisone, phosphorylation of IB, an indicator of NFκB activation, was investigated by western blotting.
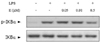
Figure 6
The schematic summary of cortisone-mediated suppression of inflammatory responses to LPS. LPS mediates inflammatory responses via NFκB signaling pathway. SW982 cells effectively converted cortisone to active cortisol, releasing it into the culture medium to act back on themselves in an autocrine manner.
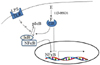
References
1. Stephens MA, Wand G. Stress and the HPA axis: role of glucocorticoids in alcohol dependence. Alcohol Res. 2012; 34:468–483.
2. Svendsen PF, Madsbad S, Nilas L, Paulsen SK, Pedersen SB. Expression of 11beta-hydroxysteroid dehydrogenase 1 and 2 in subcutaneous adipose tissue of lean and obese women with and without polycystic ovary syndrome. Int J Obes (Lond). 2009; 33:1249–1256.


3. Du H, Liu L, Wang Y, Nakagawa Y, Lyzlov A, Lutfy K, Friedman TC, Peng X, Liu Y. Specific reduction of G6PT may contribute to downregulation of hepatic 11beta-HSD1 in diabetic mice. J Mol Endocrinol. 2013; 50:167–178.


4. Wyrwoll CS, Holmes MC, Seckl JR. 11beta-hydroxysteroid dehydrogenases and the brain: from zero to hero, a decade of progress. Front Neuroendocrinol. 2011; 32:265–286.


5. Biedasek K, Andres J, Mai K, Adams S, Spuler S, Fielitz J, Spranger J. Skeletal muscle 11beta-HSD1 controls glucocorticoid-induced proteolysis and expression of E3 ubiquitin ligases atrogin-1 and MuRF-1. PLoS One. 2011; 6:e16674.


6. Wake DJ, Walker BR. 11 beta-hydroxysteroid dehydrogenase type 1 in obesity and the metabolic syndrome. Mol Cell Endocrinol. 2004; 215:45–54.


7. Arampatzis S, Kadereit B, Schuster D, Balazs Z, Schweizer RA, Frey FJ, Langer T, Odermatt A. Comparative enzymology of 11beta-hydroxysteroid dehydrogenase type 1 from six species. J Mol Endocrinol. 2005; 35:89–101.


8. Singh Y, Kotwal N, Menon AS. Endocrine hypertension - Cushing's syndrome. Indian J Endocrinol Metab. 2011; 15:Suppl 4. S313–S316.


9. Neary N, Nieman L. Adrenal insufficiency: etiology, diagnosis and treatment. Curr Opin Endocrinol Diabetes Obes. 2010; 17:217–223.


10. Yeager MP, Pioli PA, Guyre PM. Cortisol exerts bi-phasic regulation of inflammation in humans. Dose Response. 2011; 9:332–347.


11. Coutinho AE, Chapman KE. The anti-inflammatory and immunosuppressive effects of glucocorticoids, recent developments and mechanistic insights. Mol Cell Endocrinol. 2011; 335:2–13.


12. Chang JH, Lee KJ, Kim SK, Yoo DH, Kang TY. Validity of SW982 synovial cell line for studying the drugs against rheumatoid arthritis in fluvastatin-induced apoptosis signaling model. Indian J Med Res. 2014; 139:117–124.
13. Song JS, Kim CH, Heo JY, Cho YS. Rosiglitazone reduces a wide range of proinflammatory profiles in synovial fibroblast SW982 under spheroid culture. Immunol Lett. 2010; 131:81–88.


14. Cho YS, Kim CH, Cheon HG. Cell-based assay for screening 11beta-hydroxysteroid dehydrogenase 1 inhibitors. Anal Biochem. 2009; 392:110–116.


15. Christiansen JJ, Djurhuus CB, Gravholt CH, Iversen P, Christiansen JS, Schmitz O, Weeke J, Jorgensen JO, Moller N. Effects of cortisol on carbohydrate, lipid, and protein metabolism: studies of acute cortisol withdrawal in adrenocortical failure. J Clin Endocrinol Metab. 2007; 92:3553–3559.


16. Monder C, Coufalik A. Influence of cortisol on glycogen synthesis and gluconeogenesis in fetal rat liver in organ culture. J Biol Chem. 1972; 247:3608–3617.


17. Shultz TD, Bollman S, Kumar R. Decreased intestinal calcium absorption in vivo and normal brush border membrane vesicle calcium uptake in cortisol-treated chickens: evidence for dissociation of calcium absorption from brush border vesicle uptake. Proc Natl Acad Sci U S A. 1982; 79:3542–3546.


20. Flammer JR, Rogatsky I. Minireview: Glucocorticoids in autoimmunity: unexpected targets and mechanisms. Mol Endocrinol. 2011; 25:1075–1086.


21. Annane D. Glucocorticoids in the treatment of severe sepsis and septic shock. Curr Opin Crit Care. 2005; 11:449–453.


22. Sedwick C. Wanted: a new model for glucocorticoid receptor transactivation and transrepression. PLoS Biol. 2014; 12:e1001814.


23. Palacios R, Sugawara I. Hydrocortisone abrogates proliferation of T cells in autologous mixed lymphocyte reaction by rendering the interleukin-2 Producer T cells unresponsive to interleukin-1 and unable to synthesize the T-cell growth factor. Scand J Immunol. 1982; 15:25–31.


24. Vukelic S, Stojadinovic O, Pastar I, Rabach M, Krzyzanowska A, Lebrun E, Davis SC, Resnik S, Brem H, Tomic-Canic M. Cortisol synthesis in epidermis is induced by IL-1 and tissue injury. J Biol Chem. 2011; 286:10265–10275.


25. Yamazaki T, Yokoyama T, Akatsu H, Tukiyama T, Tokiwa T. Phenotypic characterization of a human synovial sarcoma cell line, SW982, and its response to dexamethasone. In Vitro Cell Dev Biol Anim. 2003; 39:337–339.


26. Esteves CL, Verma M, Rog-Zielinska E, Kelly V, Sai S, Breton A, Donadeu FX, Seckl JR, Chapman KE. Pro-inflammatory cytokine induction of 11beta-hydroxysteroid dehydrogenase type 1 in A549 cells requires phosphorylation of C/EBPbeta at Thr235. PLoS One. 2013; 8:e75874.
27. Itoi S, Terao M, Murota H, Katayama I. 11beta-Hydroxysteroid dehydrogenase 1 contributes to the proinflammatory response of keratinocytes. Biochem Biophys Res Commun. 2013; 440:265–270.


28. Philip AM, Vijayan MM. Stress-immune-growth interactions: cortisol modulates suppressors of cytokine signaling and JAK/STAT pathway in rainbow trout liver. PLoS One. 2015; 10:e0129299.


29. Newton R. Molecular mechanisms of glucocorticoid action: what is important? Thorax. 2000; 55:603–613.

