Abstract
Graft-versus-host disease (GHVD) is a severe complication after allogeneic hematopoietic stem cell transplantation. The degree of inflammation in the gastrointestinal tract, a major GVHD target organ, correlates with the disease severity. Intestinal inflammation is initiated by epithelial damage caused by pre-conditioning irradiation. In combination with damages caused by donor-derived T cells, such damage disrupts the epithelial barrier and exposes innate immune cells to pathogenic and commensal intestinal bacteria, which release ligands for Toll-like receptors (TLRs). Dysbiosis of intestinal microbiota and signaling through the TLR/myeloid differentiation primary response gene 88 (MyD88) pathways contribute to the development of intestinal GVHD. Understanding the changes in the microbial flora and the roles of TLR signaling in intestinal GVHD will facilitate the development of preventative and therapeutic strategies.
Allogeneic (allo) hematopoietic stem cell transplantation (HSCT) is an effective treatment for hematological disorders, including lymphoma and leukemia (1234). Graft-versus-leukemia (GVL) effects, which are derived from the activation of donor T cells that recognize the allo-antigens expressed by the recipient's tumor cells, contribute to the eradication of malignant host cells (5). However, donor T cells are also reactive to allo-antigens expressed by the recipient's tissues and parenchymal cells in the gastrointestinal (GI) tract, liver, lung, and skin, and induce graft-versus-host disease (GVHD), a life-threatening complication of allo-HSCT (67). The suppression of severe GVHD is important for the success of allo-HSCT.
GI tract damage is a critical event in the pathogenesis of GVHD (89). The integrity of the GI tract and innate immunity to the intestinal microbiome both contributes to the maintenance of intestinal homeostasis; disruption of intestinal homeostasis during allo-HSCT provokes intestinal GVHD, which leads to exacerbation of the disease and systemic GVHD (9). Signaling through Toll-like receptors (TLRs) and myeloid differentiation factor 88 (MyD88), a signaling adaptor downstream of TLRs, is pivotal in innate immunity that controls response to microbial stimulation; evidence supporting the significances of their signaling in GVHD is accumulating (1011). In this article, we will review recent research into the role of TLR/MyD88-mediated innate immunity in acute intestinal GVHD.
GVHD is broadly classified into acute and chronic GVHD, depending on the timing of disease incidence after allo-HSCT. Chronic GVHD was classically defined as a late complication of allo-BMT that occurs in 100 days post-transplantation. Chronic GVHD is similar to autoimmune and other immunological diseases, such as scleroderma (1213), systemic lupus-like diseases (14), primary biliary cirrhosis (15), and immune cytopenia (16); it is characterized by tissue inflammation and fibrosis, and is mediated by cellular and CD4 T helper cell type 2-dependent humoral immunity (1718). In 2014, revised chronic GVHD criteria were proposed, which facilitate distinction of chronic and acute GVHD, that include diagnostics in the skin (e.g., poikiloderma and sclerotic features including lichen planus-like features), mouth (e.g., lichen planus-like changes), lung (e.g., bronchiolitis obliterans), and GI tract (e.g., esophageal web, strictures or stenosis in the upper to middle third of the esophagus) (19).
Development of acute GVHD is observed within 100 days post-HSCT, with symptoms indicating damage to the skin (e.g., maculopapular rash on the palms, soles and ears, and diffuse erythematous rash over the entire body), liver (e.g., hyperbilirubinemia, jaundice, and elevated transaminases), GI tract (e.g., nausea, vomiting, abdominal cramps, anorexia, bleeding, and diarrhea), and, occasionally, lungs, eyes and oral mucosa (20). Although donor T cell-mediated adaptive immunity is an essential component of the development of acute GVHD, innate immunity also plays significant roles (62122). Chemoirradiation conditioning of recipients prior to HSCT provokes apoptosis of epithelial cells and tissue inflammation in several organs, including the intestines. The release of inflammatory cytokines activates antigen-presenting cells (APCs), which promote the activation and effector differentiation of allo-reactive donor T cells. Activated of T cells mediate cytotoxicity against allo-antigen-bearing recipient cells in damaged tissues, which increase inflammation in the target organs (Fig. 1). In particular, intestinal inflammation initiated by epithelial cell damage disrupts the epithelial barrier, which exposes innate immune cells to intestinal microbial stimuli. This innate cell stimulation by microbial antigens enhances the recruitment of activated T cells to the intestines, where they kill GI epithelial cells and cause cryptic cell degeneration, resulting in heightened intestinal inflammation and nutrient malabsorption. The degree of intestinal inflammation is associated with the severity of acute GVHD. Acute intestinal GVHD occurs in more than 50% of allo-HSCT patients (23).
The gut microbiome consists of diverse sets of bacteria, fungi, archaea, and viruses (24). Under physiological conditions, 1014 bacteria from 200 to 1500 species are approximated to exist in the colon (2526). Alterations to or loss of intestinal microbiome diversity is related to the aggravation of acute GVHD (2728). In a murine acute GVHD model, distinct microbes in the ileum were highly decreased (e.g., Clostridiales and phylum Firmicutes) or increased (e.g., Lactobacillus johnsonii) compared to bone marrow transplanted control mice without GVHD counterparts. L. johnsonii participated in the amelioration of acute GVHD by suppressing Enterococcus spp. (27). Inhibition of the production of the antimicrobial peptide α-defensin by Paneth cells reduced the physiological diversity of the microflora and permitted expansion of Escherichia coli in GVHD mice (28). Antibiotic treatment to reduce gram-negative bacteria in the GI tract ameliorated acute GVHD severity (29). Shifts in the gut microbiota towards enterobacteria, enterococci, and Bacteroides/Prevotella spp. are associated with increased inflammatory responses in intestinal GVHD (11). Thus, the intestinal microbiota could potentially be manipulated to improve allo-HSCT outcomes.
Innate pattern recognition receptors (PRRs), such as TLRs and nucleotide oligomerization domain (NOD)-like receptors (NLRs), recognize intestinal bacterial pathogens and/or pathogenic molecules. Ligand binding by the TLRs and NLRs expressed on host and/or donor-derived APCs substantially amplifies the release of inflammatory mediators (30). The transfer of HoxB8 neutrophils that lack expression of TLR 2, 3 4, 7, and 9 reduced GVHD severity compared with the transfer of WT HoxB8 neutrophils, indicating that TLR signals promote GVHD development (31). Conditioning-induced GI damage allows the translocation of outer membrane-derived endotoxins from gram-negative bacteria (e.g., lipopolysaccharide (LPS)) into systemic circulation (113233). The binding of LPS to TLR4 accelerated lethal intestinal GVHD by stimulating the production of inflammatory cytokines (e.g., TNFα, IL-1, IL-6, IL-10, IL-12, and TGFβ) from gut-associated lymphoid tissues (GALTs) and macrophages, and IFN-γ from activated donor T cells (934). The endogenous TLR4 agonist heparan sulfate activated dendritic cells (DCs) and aggravated acute GVHD (35). Unexpectedly, however, Tlr4–/– mic developed fulminant GVHD, and allogeneic hosts with a TLR4 mutation (C3H/HeJ mice) had increased intestinal damage compared to wild type counterparts (3637). TLR4 signaling mediated protective effects during GVHD, characterized by reduced intestinal cell apoptosis compared to that in hosts that did not undergo TLR4 signaling (36). In addition, TLR4 ligands were not necessary for the maturation of host APCs for GVHD induction (37). Collectively, these finding suggest that TLR4 signaling is involved in both positive and negative regulation of GVHD. Tlr9–/– mice developed less severe acute GVHD post-HSCT than controls (1138). Consistent with these findings, treatment of wild type mice with a synthetic TLR9 agonist (CpG oligonucleotides) markedly accelerated GVHD severity (39), and treatment with the TLR9-inhibitory oligonucleotide (iODN) 2088 reduced apoptosis of colonic cells in intestinal GVHD (1139). Thus, TLR9 signaling is associated with the induction of intestinal GVHD.
Application of the TLR7/8 agonist R-848 (resiquimod) promoted substantial innate immune activation and T cell migration into target organs (40). Another TLR7/8 agonist, 3M-011, caused differential effects on GVHD depending on the timing of the treatment. Administration of 3M-011 after allogenic transplant increased GVHD mortality, but pre-treatment with 3M-011 reduced the damage to target organs by inducing IDO expression in the colon (394142). Alterations to TLR2 expression on recipient lymphoid and myeloid cells from splenocytes had little effect on acute GVHD (43) (Table I). Thus, each of the TLRs is involved in acute GVHD to a different extent (43444546). Reports on the functional associations of TLRs and their adaptor molecules with GVHD are summarized in Table I and Table II.
MyD88 is an adaptor molecule that activates inflammatory responses downstream of TLR ligand ligation (Table II) (474849). All TLRs, except TLR3, transduce signals through MyD88 (50). In MyD88-deficient recipient mice, the infiltration of donor T cells into the intestines and the apoptosis of colon cells were reduced, resulting in improved survival and clinical scoring for acute intestinal GVHD (11). However, MyD88-deficiency in donor bone marrow (BM) cells aggravated GVHD, resulting in increased intestinal pathology (51). The exacerbation of intestinal GVHD in recipients of MyD88-deficient BM cells was associated with insufficient expansion of MDSCs from the transplanted MyD88-deficient stem cells. These findings indicate that MyD88 signaling in donor cells promotes MDSC expansion and immune suppression in acute GVHD. The transfer of WT MDSCs into recipients of MyD88-deficient BM cells ameliorated intestinal GVHD, which supports a role for MyD88 in driving MDSC expansion in GVHD. Thus, MyD88 signaling has opposite impacts on intestinal GVHD, depending on whether MyD88 is expressed by host or donor cells.
MDSCs consist of two main subtypes: granulocytic/polymorphonuclear MDSCs and monocytic MDSCs. The phenotypes CD11b+LyG6+Ly6Clow and CD11b+LyG6lowLy6Chigh are used to identify the respective populations in mice. MDSCs expand robustly in various pathological conditions, such as cancers (52), autoimmune diseases (53), inflammation (54), infectious diseases (55565758), and GVHD (49515960). Most MDSC biology has been studied in tumor microenvironments, and preclinical and clinical tumor therapies have been tested for their ability to block MDSC expansion and function. Inhibitors of vascular endothelial growth factor (VEGF; bevacizumab) (61), signal transducer and activator of transcription 3 (STAT3; sunitinib) (62), arginase (NOHA) (52), inducible nitric oxide synthase (iNOS; nitroaspirin) (63), and cyclooxygenase-2 (COX2; celecoxib) (64), as well as agents that induce MDSC apoptosis and necrosis (gemcitabine and IL4Rα aptamer), have been shown to decrease MDSC expansion and tumor growth (6566). The expansion and functional enhancement of MDSCs are required for the control of acute intestinal GVHD. Arginase-1, iNOS, reactive oxygen species (ROS), and nitric oxide (NO) are mediators of the suppressive functions of MDSCs (52). Inflammatory mediators such as COX-2, G-CSF, GM-CSF, IFN-γ, IL-6, IL-10, VEFG, and prostaglandin E2 induce the differentiation and expansion of MDSCs, and inhibit the differentiation of mature myeloid cells in pathogenic environments (6768). These mediators could be targeted to enhance the suppressive functions of MDSCs to ameliorate GVHD. The selective modulation or exploitation of MyD88-mediated signaling to induce MDSC expansion and functional enhancement could be a strategy to suppress acute intestinal GVHD.
The dysregulation of microbial homeostasis and TLR signaling-mediated inflammatory responses are involved in the pathogenesis of intestinal GVHD. Understanding the effects and cellular/molecular mechanisms of TLR/MyD88 signaling on innate immune regulation of gut bacteria and MDSCs would aid the development of specific immune modulators to treat intestinal GVHD.
Figures and Tables
Figure 1
Schematic diagram of the development of acute GVHD. Acute GVHD can be classified into five distinct phases. Conditioning regimens (radiation or chemotherapy) induce tissue damage (I), and increase production of inflammatory cytokines, which cause the activation and maturation of APCs (II), leading to allo-reactive donor T cell priming and expansion (III). Activated donor T cells migrate to damaged host tissues (IV), where they amplify inflammatory responses and worsen GVHD (V). DC, dendritic cell; MΦ, macrophage.
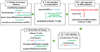
Table I
Studies of GVHD associated with innate immune responses through TLRs
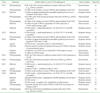
TLRs | Treatments | Results related to acute GVHD pathogenesis | Donor/recipient | References |
---|---|---|---|---|
TLR1 | SNP genotyping | SNPs in the TLR1 showed significant association with acute GVHD (e. g., SNP id: rs483307) | Donor (human) | 44 |
SNP genotyping | No effect on the incidence of acute GVHD by polymorphisms of the TLR1 | Donor (human) | 45 | |
TLR2 | Deficient | No effect on apoptosis/proliferation/neutrophilic granulocytes/survival in intestinal GVHD, donor T cells ↓ | Recipient (mouse) | 11 |
SNP genotyping | Four SNPs in the TLR2 showed association with acute GVHD (e.g., SNP id: rs6535927) | Donor (human) | 44 | |
SNP genotyping | No effect on the incidence of acute GVHD by polymorphisms of the TLR2 | Donor (human) | 45 | |
Deficient | No effect on acute GVHD by upregulation of TLR2 expression in G-CSF-mobilized donor grafts | Donor (mouse) | 43 | |
Deficient | GVHD severity ↓, translocating bacteria ↓ (in TLR2/3/4/7/9– in HoxB8 neutrophils) | Recipient (mouse) | 31 | |
TLR3 | Deficient | GVHD severity ↓, translocating bacteria ↓ (in TLR2/3/4/7/9– in HoxB8 neutrophils) | Recipient (mouse) | 31 |
SNP genotyping | No effect on the incidence of acute GVHD by polymorphisms of the TLR3 | Donor (human) | 45 | |
TLR4 | Deficient | No effects on apoptosis/proliferation/neutrophilic granulocytes/survival in intestinal GVHD, donor T cells ↓ | Recipient (mouse) | 11 |
Agonist | GVHD severity ↑, alloreactive donor T cell proliferation ↑ | Recipient (mouse) | 35 | |
Deficient | Protection against intestinal cell apoptosis during acute GVHD by induction of tissue protective factors | Recipient (mouse) | 36 | |
Mutation | No difference in GVHD in HLA-matched HCT with mutation in donor | Both (human) | 46 | |
Deficient | No effect on GVHD severity | Donor (mouse) | 37 | |
TLR5 | SNP genotyping | SNP in the TLR5 showed no sufficient evidence for the TLR5 importance in GVHD | Donor (human) | 44 |
TLR6 | SNP genotyping | SNP in the TLR6 showed association with acute GVHD (e.g., SNP id: rs6531656) | Both (human) | 44 |
TLR7 | Deficient | GVHD severity ↓, translocating bacteria ↓ (in TLR2/3/4/7/9– in HoxB8 neutrophils) | Recipient (mouse) | 31 |
Agonist | Localized GVHD ↑ , infiltration of donor T cells ↑ | Recipient (mouse) | 39 | |
TLR8 | SNP genotyping | No effect on the incidence of acute GVHD by polymorphisms of the TLR8 | Donor (human) | 45 |
TLR9 | Deficient | GVHD severity ↓, translocating bacteria ↓ (in TLR2/3/4/7/9– in HoxB8 neutrophils) | Recipient (mouse) | 31 |
Deficient | Intestinal GVHD severity ↓ (dependent on MyD88 signaling), survival rates ↑ | in-17-144-i001 | 11 | |
Agonist | GVHD severity ↑ | Recipient (mouse) | 11 | |
Deficient | GVHD severity ↓, apoptotic cells , proliferation of cells in colon ↑ | Recipient (mouse) | 44 | |
SNP genotyping | Associated with the risk of acute GVHD by TLR9 SNPs in the donors of allogeneic HSCT | Donor (human) | 45 | |
TLR10 | SNP genotyping | SNP in the TLR10 showed significant association with acute GVHD (e.g., SNP id: rs337629) | Both (human) | 44 |
Table II
Studies of GVHD associated with innate immune responses through TLR adaptor molecules
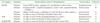
ACKNOWLEDGEMENTS
This work was supported by the Education and Research Encouragement Fund of Seoul National University Hospital (2017).
References
1. Mohty M, de LH, Ladaique P, Faucher C, Vey N, Coso D, Stoppa AM, Gastaut JA, Blaise D. The role of reduced intensity conditioning allogeneic stem cell transplantation in patients with acute myeloid leukemia: a donor vs no donor comparison. Leukemia. 2005; 19:916–920.


2. Poon LM, Bassett R Jr, Rondon G, Hamdi A, Qazilbash M, Hosing C, Jones RB, Shpall EJ, Popat UR, Nieto Y, Worth LL, Cooper L, De LM, Champlin RE, Kebriaei P. Outcomes of second allogeneic hematopoietic stem cell transplantation for patients with acute lymphoblastic leukemia. Bone Marrow Transplant. 2013; 48:666–670.


3. Demirer T, Barkholt L, Blaise D, Pedrazzoli P, Aglietta M, Carella AM, Bay JO, Arpaci F, Rosti G, Gurman G, Niederwieser D, Bregni M. Transplantation of allogeneic hematopoietic stem cells: an emerging treatment modality for solid tumors. Nat Clin Pract Oncol. 2008; 5:256–267.


4. Dvorak CC, Cowan MJ. Hematopoietic stem cell transplantation for primary immunodeficiency disease. Bone Marrow Transplant. 2008; 41:119–126.


5. Warren EH, Fujii N, Akatsuka Y, Chaney CN, Mito JK, Loeb KR, Gooley TA, Brown ML, Koo KK, Rosinski KV, Ogawa S, Matsubara A, Appelbaum FR, Riddell SR. Therapy of relapsed leukemia after allogeneic hematopoietic cell transplantation with T cells specific for minor histocompatibility antigens. Blood. 2010; 115:3869–3878.


6. Blazar BR, Murphy WJ, Abedi M. Advances in graft-versus-host disease biology and therapy. Nat Rev Immunol. 2012; 12:443–458.


8. Washington K, Jagasia M. Pathology of graft-versus-host disease in the gastrointestinal tract. Hum Pathol. 2009; 40:909–917.


9. Hill GR, Ferrara JL. The primacy of the gastrointestinal tract as a target organ of acute graft-versus-host disease: rationale for the use of cytokine shields in allogeneic bone marrow transplantation. Blood. 2000; 95:2754–2759.


10. Fukata M, Vamadevan AS, Abreu MT. Toll-like receptors (TLRs) and Nod-like receptors (NLRs) in inflammatory disorders. Semin Immunol. 2009; 21:242–253.


11. Heimesaat MM, Nogai A, Bereswill S, Plickert R, Fischer A, Loddenkemper C, Steinhoff U, Tchaptchet S, Thiel E, Freudenberg MA, Gobel UB, Uharek L. MyD88/TLR9 mediated immunopathology and gut microbiota dynamics in a novel murine model of intestinal graft-versus-host disease. Gut. 2010; 59:1079–1087.


12. Marcellus DC, Altomonte VL, Farmer ER, Horn TD, Freemer CS, Grant J, Vogelsang GB. Etretinate therapy for refractory sclerodermatous chronic graft-versus-host disease. Blood. 1999; 93:66–70.


13. Pai CC, Chen M, Mirsoian A, Grossenbacher SK, Tellez J, Ames E, Sun K, Jagdeo J, Blazar BR, Murphy WJ, Abedi M. Treatment of chronic graft-versus-host disease with bortezomib. Blood. 2014; 124:1677–1688.


14. Rozendaal L, Pals ST, Gleichmann E, Melief CJ. Persistence of allospecific helper T cells is required for maintaining autoantibody formation in lupus-like graft-versus-host disease. Clin Exp Immunol. 1990; 82:527–532.


15. Wakae T, Takatsuka H, Seto Y, Iwata N, Mori A, Okada M, Fujimori Y, Okamoto T, Kakishita E, Hara H. Similarity between hepatic graft-versus-host disease and primary biliary cirrhosis. Hematology. 2002; 7:305–310.


16. Shono Y, Shiratori S, Kosugi-Kanaya M, Ueha S, Sugita J, Shigematsu A, Kondo T, Hashimoto D, Fujimoto K, Endo T, Nishio M, Hashino S, Matsuno Y, Matsushima K, Tanaka J, Imamura M, Teshima T. Bone marrow graft-versus-host disease: evaluation of its clinical impact on disrupted hematopoiesis after allogeneic hematopoietic stem cell transplantation. Biol Blood Marrow Transplant. 2014; 20:495–500.


17. Silva R, Morgado JM, Freitas A, Couceiro A, Orfao A, Regateiro F, Paiva A. Influence of pro- and anti-inflammatory cytokines in Th1 polarization after allogeneic stimulation. Int J Biomed Sci. 2005; 1:46–52.
18. Biedermann BC, Sahner S, Gregor M, Tsakiris DA, Jeanneret C, Pober JS, Gratwohl A. Endothelial injury mediated by cytotoxic T lymphocytes and loss of microvessels in chronic graft versus host disease. Lancet. 2002; 359:2078–2083.


19. Jagasia MH, Greinix HT, Arora M, Williams KM, Wolff D, Cowen EW, Palmer J, Weisdorf D, Treister NS, Cheng GS, Kerr H, Stratton P, Duarte RF, McDonald GB, Inamoto Y, Vigorito A, Arai S, Datiles MB, Jacobsohn D, Heller T, Kitko CL, Mitchell SA, Martin PJ, Shulman H, Wu RS, Cutler CS, Vogelsang GB, Lee SJ, Pavletic SZ, Flowers ME. National institutes of health consensus development project on criteria for clinical trials in chronic graft-versus-host disease: I. The 2014 diagnosis and staging working group report. Biol Blood Marrow Transplant. 2015; 21:389–401.


22. Ju JM, Lee H, Oh K, Lee DS, Choi EY. Kinetics of IFN-gamma and IL-17 production by CD4 and CD8 T cells during acute graft-versus-host disease. Immune Netw. 2014; 14:89–99.


23. Ross WA, Ghosh S, Dekovich AA, Liu S, Ayers GD, Cleary KR, Lee JH, Couriel D. Endoscopic biopsy diagnosis of acute gastrointestinal graft-versus-host disease: rectosigmoid biopsies are more sensitive than upper gastrointestinal biopsies. Am J Gastroenterol. 2008; 103:982–989.


24. Mai V, Draganov PV. Recent advances and remaining gaps in our knowledge of associations between gut microbiota and human health. World J Gastroenterol. 2009; 15:81–85.


25. Sender R, Fuchs S, Milo R. Revised estimates for the number of human and bacteria cells in the body. PLoS Biol. 2016; 14:e1002533.


26. Savage DC. Microbial ecology of the gastrointestinal tract. Annu Rev Microbiol. 1977; 31:107–133.


27. Jenq RR, Ubeda C, Taur Y, Menezes CC, Khanin R, Dudakov JA, Liu C, West ML, Singer NV, Equinda MJ, Gobourne A, Lipuma L, Young LF, Smith OM, Ghosh A, Hanash AM, Goldberg JD, Aoyama K, Blazar BR, Pamer EG, van den Brink MR. Regulation of intestinal inflammation by microbiota following allogeneic bone marrow transplantation. J Exp Med. 2012; 209:903–911.


28. Eriguchi Y, Takashima S, Oka H, Shimoji S, Nakamura K, Uryu H, Shimoda S, Iwasaki H, Shimono N, Ayabe T, Akashi K, Teshima T. Graft-versus-host disease disrupts intestinal microbial ecology by inhibiting Paneth cell production of alpha-defensins. Blood. 2012; 120:223–231.


29. Beelen DW, Elmaagacli A, Muller KD, Hirche H, Schaefer UW. Influence of intestinal bacterial decontamination using metronidazole and ciprofloxacin or ciprofloxacin alone on the development of acute graft-versus-host disease after marrow transplantation in patients with hematologic malignancies: final results and long-term follow-up of an open-label prospective randomized trial. Blood. 1999; 93:3267–3275.


30. Murphy S, Nguyen VH. Role of gut microbiota in graft-versus-host disease. Leuk Lymphoma. 2011; 52:1844–1856.


31. Schwab L, Goroncy L, Palaniyandi S, Gautam S, Triantafyllopoulou A, Mocsai A, Reichardt W, Karlsson FJ, Radhakrishnan SV, Hanke K, Schmitt-Graeff A, Freudenberg M, von Loewenich FD, Wolf P, Leonhardt F, Baxan N, Pfeifer D, Schmah O, Schonle A, Martin SF, Mertelsmann R, Duyster J, Finke J, Prinz M, Henneke P, Hacker H, Hildebrandt GC, Hacker G, Zeiser R. Neutrophil granulocytes recruited upon translocation of intestinal bacteria enhance graft-versus-host disease via tissue damage. Nat Med. 2014; 20:648–654.


32. Hill GR, Crawford JM, Cooke KR, Brinson YS, Pan L, Ferrara JL. Total body irradiation and acute graft-versus-host disease: the role of gastrointestinal damage and inflammatory cytokines. Blood. 1997; 90:3204–3213.


33. Cooke KR, Gerbitz A, Crawford JM, Teshima T, Hill GR, Tesolin A, Rossignol DP, Ferrara JL. LPS antagonism reduces graft-versus-host disease and preserves graft-versus-leukemia activity after experimental bone marrow transplantation. J Clin Invest. 2001; 107:1581–1589.


34. Holler E. Cytokines, viruses, and graft-versus-host disease. Curr Opin Hematol. 2002; 9:479–484.


35. Brennan TV, Lin L, Huang X, Cardona DM, Li Z, Dredge K, Chao NJ, Yang Y. Heparan sulfate, an endogenous TLR4 agonist, promotes acute GVHD after allogeneic stem cell transplantation. Blood. 2012; 120:2899–2908.


36. Imado T, Iwasaki T, Kitano S, Satake A, Kuroiwa T, Tsunemi S, Sano H. The protective role of host Toll-like receptor-4 in acute graft-versus-host disease. Transplantation. 2010; 90:1063–1070.


37. Li H, Matte-Martone C, Tan HS, Venkatesan S, McNiff J, Demetris AJ, Jain D, Lakkis F, Rothstein D, Shlomchik WD. Graft-versus-host disease is independent of innate signaling pathways triggered by pathogens in host hematopoietic cells. J Immunol. 2011; 186:230–241.


38. Calcaterra C, Sfondrini L, Rossini A, Sommariva M, Rumio C, Menard S, Balsari A. Critical role of TLR9 in acute graft-versus-host disease. J Immunol. 2008; 181:6132–6139.


39. Taylor PA, Ehrhardt MJ, Lees CJ, Panoskaltsis-Mortari A, Krieg AM, Sharpe AH, Murphy WJ, Serody JS, Hemmi H, Akira S, Levy RB, Blazar BR. TLR agonists regulate alloresponses and uncover a critical role for donor APCs in allogeneic bone marrow rejection. Blood. 2008; 112:3508–3516.


40. Spranger S, Javorovic M, Burdek M, Wilde S, Mosetter B, Tippmer S, Bigalke I, Geiger C, Schendel DJ, Frankenberger B. Generation of Th1-polarizing dendritic cells using the TLR7/8 agonist CL075. J Immunol. 2010; 185:738–747.


41. Hemmi H, Kaisho T, Takeuchi O, Sato S, Sanjo H, Hoshino K, Horiuchi T, Tomizawa H, Takeda K, Akira S. Small anti-viral compounds activate immune cells via the TLR7 MyD88-dependent signaling pathway. Nat Immunol. 2002; 3:196–200.


42. Jasperson LK, Bucher C, Panoskaltsis-Mortari A, Mellor AL, Munn DH, Blazar BR. Inducing the tryptophan catabolic pathway, indoleamine 2,3-dioxygenase (IDO), for suppression of graft-versus-host disease (GVHD) lethality. Blood. 2009; 114:5062–5070.


43. Lee WS, Kim JY, Won HJ, Lee SM, Suh YS, Joo YD, Lee JY, Jang WH, Kang SW, Kang MS, Park SG, Choi IW, Choi I, Seo SK. Effect of upregulated TLR2 expression from G-CSF-mobilized donor grafts on acute graft-versus-host disease. Int Immunopharmacol. 2015; 29:488–493.


44. Sivula J, Cordova ZM, Tuimala J, Jaatinen T, Partanen J, Volin L, Turpeinen H. Toll-like receptor gene polymorphisms confer susceptibility to graft-versus-host disease in allogenic hematopoietic stem cell transplantation. Scand J Immunol. 2012; 76:336–341.


45. Xiao HW, Luo Y, Lai XY, Shi JM, Tan YM, He JS, Xie WZ, Zheng WY, Ye XJ, Yu XH, Cai Z, Lin MF, Huang H. Donor TLR9 gene tagSNPs influence susceptibility to aGVHD and CMV reactivation in the allo-HSCT setting without polymorphisms in the TLR4 and NOD2 genes. Bone Marrow Transplant. 2014; 49:241–247.


46. Lorenz E, Schwartz DA, Martin PJ, Gooley T, Lin MT, Chien JW, Hansen JA, Clark JG. Association of TLR4 mutations and the risk for acute GVHD after HLA-matched-sibling hematopoietic stem cell transplantation. Biol Blood Marrow Transplant. 2001; 7:384–387.


49. Lim JY, Lee YK, Lee SE, Ju JM, Park G, Choi EY, Min CK. Attenuation of hepatic graft-versus-host disease in allogeneic recipients of MyD88-deficient donor bone marrow. Immune Netw. 2015; 15:125–134.


50. Yamamoto M, Sato S, Hemmi H, Hoshino K, Kaisho T, Sanjo H, Takeuchi O, Sugiyama M, Okabe M, Takeda K, Akira S. Role of adaptor TRIF in the MyD88-independent toll-like receptor signaling pathway. Science. 2003; 301:640–643.


51. Lim JY, Lee YK, Lee SE, Ju JM, Eom KS, Kim YJ, Chung NG, Jeong DC, Park G, Choi EY, Min CK. MyD88 in donor bone marrow cells is critical for protection from acute intestinal graft-vs.-host disease. Mucosal Immunol. 2016; 9:730–743.


52. Gabrilovich DI, Ostrand-Rosenberg S, Bronte V. Coordinated regulation of myeloid cells by tumours. Nat Rev Immunol. 2012; 12:253–268.


53. Crook KR, Liu P. Role of myeloid-derived suppressor cells in autoimmune disease. World J Immunol. 2014; 4:26–33.


54. Smith AR, Reynolds JM. Editorial: the contribution of myeloid-derived suppression to inflammatory disease. J Leukoc Biol. 2014; 96:361–364.


55. Chandra D, Jahangir A, Quispe-Tintaya W, Einstein MH, Gravekamp C. Myeloid-derived suppressor cells have a central role in attenuated Listeria monocytogenes-based immunotherapy against metastatic breast cancer in young and old mice. Br J Cancer. 2013; 108:2281–2290.


56. Schmid M, Zimara N, Wege AK, Ritter U. Myeloid-derived suppressor cell functionality and interaction with Leishmania major parasites differ in C57BL/6 and BALB/c mice. Eur J Immunol. 2014; 44:3295–3306.


57. Terrazas LI, Walsh KL, Piskorska D, McGuire E, Harn DA Jr. The schistosome oligosaccharide lacto-N-neotetraose expands Gr1(+) cells that secrete anti-inflammatory cytokines and inhibit proliferation of naive CD4(+) cells: a potential mechanism for immune polarization in helminth infections. J Immunol. 2001; 167:5294–5303.


58. Gabrilovich DI, Nagaraj S. Myeloid-derived suppressor cells as regulators of the immune system. Nat Rev Immunol. 2009; 9:162–174.


59. Koehn BH, Apostolova P, Haverkamp JM, Miller JS, McCullar V, Tolar J, Munn DH, Murphy WJ, Brickey WJ, Serody JS, Gabrilovich DI, Bronte V, Murray PJ, Ting JP, Zeiser R, Blazar BR. GVHD-associated, inflammasome-mediated loss of function in adoptively transferred myeloid-derived suppressor cells. Blood. 2015; 126:1621–1628.


60. Messmann JJ, Reisser T, Leithauser F, Lutz MB, Debatin KM, Strauss G. In vitro-generated MDSCs prevent murine GVHD by inducing type 2 T cells without disabling antitumor cytotoxicity. Blood. 2015; 126:1138–1148.


61. Kusmartsev S, Eruslanov E, Kubler H, Tseng T, Sakai Y, Su Z, Kaliberov S, Heiser A, Rosser C, Dahm P, Siemann D, Vieweg J. Oxidative stress regulates expression of VEGFR1 in myeloid cells: link to tumor-induced immune suppression in renal cell carcinoma. J Immunol. 2008; 181:346–353.


62. Ko JS, Zea AH, Rini BI, Ireland JL, Elson P, Cohen P, Golshayan A, Rayman PA, Wood L, Garcia J, Dreicer R, Bukowski R, Finke JH. Sunitinib mediates reversal of myeloid-derived suppressor cell accumulation in renal cell carcinoma patients. Clin Cancer Res. 2009; 15:2148–2157.


63. De SC, Serafini P, Marigo I, Dolcetti L, Bolla M, Del SP, Melani C, Guiducci C, Colombo MP, Iezzi M, Musiani P, Zanovello P, Bronte V. Nitroaspirin corrects immune dysfunction in tumor-bearing hosts and promotes tumor eradication by cancer vaccination. Proc Natl Acad Sci U S A. 2005; 102:4185–4190.


64. Veltman JD, Lambers ME, van NM, Hendriks RW, Hoogsteden HC, Aerts JG, Hegmans JP. COX-2 inhibition improves immunotherapy and is associated with decreased numbers of myeloid-derived suppressor cells in mesothelioma. Celecoxib influences MDSC function. BMC Cancer. 2010; 10:464.


65. Wu H, Tao N, Liu X, Li X, Tang J, Ma C, Xu X, Shao H, Hou B, Wang H, Qin Z. Polysaccharide from Lentinus edodes inhibits the immunosuppressive function of myeloid-derived suppressor cells. PLoS One. 2012; 7:e51751.


66. Roth F, De La Fuente AC, Vella JL, Zoso A, Inverardi L, Serafini P. Aptamer-mediated blockade of IL4Ralpha triggers apoptosis of MDSCs and limits tumor progression. Cancer Res. 2012; 72:1373–1383.

