Abstract
Tumor necrosis factor-α (TNF) is well known to be involved in the immune system and ovarian inflammation. Ovarian cancer is an inflammation-related malignancy that lacks early screening strategies, resulting in late diagnosis followed by high mortality. Based on our previous data, TNF induced abundant serum amyloid A (SAA), an acute phase protein linked to inflammation, in ovarian granulosal cells. To date, the regulation and expression of SAA in ovarian cancer is not fully elucidated. Here, we investigated the relationship between TNF and SAA by comparing human normal ovarian tissues and serous ovarian tumors. We found that SAA1/2 was significantly expressed in tumor tissues, but no or trace expression levels in normal tissues. TNF was also significantly upregulated in ovarian tumor tissues compared to normal tissues. Moreover, TNF significantly increased SAA1/2 levels in human ovarian cancer cell lines, OVCAR-3 and SKOV-3, in a time-dependent manner. Since the SAA1 promoter contains two nuclear factor (NF)-κB sites, we examined whether TNF regulates SAA1 promoter activity. Deletion analysis revealed that the proximal NF-κB site (−95/−85) played a critical role in regulating TNF-induced SAA1 promoter activity. Within 2 h after intraperitoneal injection of lipopolysaccharide, a product known to stimulate release of TNF, SAA preferably localized to ovarian epithelial cells and the thecal-interstitial layers compared to granulosal cell layers. Based on Gene Expression Omnibus (GEO) database, SAA1/2 and TNF were dominantly expressed in advanced grade ovarian cancer. Taken together, the accumulation of SAA1/2 in ovarian cancer could be mediated by TNF-induced NF-κB activation.
Ovarian cancer is the most common cause of cancer death from gynecologic malignancies and a heterogeneous disease (1). Epithelial ovarian carcinoma (EOC) is the most common type of ovarian cancer, accounting for 80~95% of all ovarian tumors (2). High-grade serous ovarian carcinoma (HGSOC) is the most common and lethal subtype of ovarian cancer (3). Several studies have demonstrated that factors related to inflammation, such as cytokines and inflammation mediators, are associated with EOC. Women with advanced cancer had significantly elevated serum levels of interleukin (IL)-2, IL-4, IL-6, IL-10, IL-12, and IL-13 (45). Our previous studies showed that tumor necrosis factor-α (TNF) elicited proinflammatory chemokines in ovarian cancer cells via nuclear factor (NF)-κB signaling pathway activation, which was closely associated with ovarian cancer progression (678). TNF is highly expressed in ovarian cancer and plays a critical role as a regulator of the proinflammatory ovarian tumor microenvironment (910,11).
Serum amyloid A (SAA) is a highly conserved acute-phase protein, and a precursor protein in inflammation-associated reactive amyloidosis (AA-type), and produced in response to inflammation or infection. It is expressed in the liver, but a broader view of SAA expression and function has been emerging in normal, atherosclerotic, Alzheimer, inflammatory and tumor tissues (12). Proinflammatory cytokines, such as IL-6, IL-1, TNF, interferon-g, and transforming growth factor stimulate production of acute-phase SAA (13). TNF and IL-6 are involved in SAA1 and SAA2 promoter transcriptional activation in hepatic and epithelial cells (14). The expression levels of SAA in human benign and malignant ovarian epithelial tumors were investigated (15). The local expression levels of SAA increased through benign and borderline adenomas to primary and metastatic adenocarcinomas (14). In addition, high serum levels of SAA were found in patients with ovarian carcinoma (14). Although elevated levels of SAA were observed in ovarian carcinomas, the mechanisms by which SAA expression is regulated in ovarian cancer cells remain to be elucidated. Here, we examined the expression of SAA isoforms (SAA1/2 and SAA4) in human ovarian cancer tissues and delineated the involvement of TNF in regulating SAA1/2 expression. Then, we defined the role of TNF-induced NF-κB signaling on the accumulation of SAA1/2 in ovarian cancer.
Specific PCR primers for SAA1/2 and SAA4 were obtained from Eurofins MWG Operon (Huntsville, AL, USA). SYBR® Green Master Mix (cat #: 330503) and primers for TNF and GAPDH were purchased from SA Biosciences/Qiagen (Frederick, MD, USA) for qRT-PCR. Recombinant human TNF and lipopolysaccharide (LPS from Escherichia coli, 0111:B4) were obtained from R&D Systems (Minneapolis, MN, USA) and Sigma (St. Louis, MO, USA), respectively. The pGEM-T Easy Vector, pGL4.12 Luciferase Reporter Vector and the Luciferase Reporter Assay System were obtained from Promega (Madison, WI, USA). Lipofectamine, TRIzol, Moloney murine leukemia virus, SP6 and T7 RNA polymerase and all liquid culture media were acquired from Invitrogen (Grand Island, NY, USA).
The human ovarian cancer cell lines (OVCAR-3 and SKOV-3) were purchased from the American Type Culture Collection (Manassas, VA, USA). Ovarian cancer cells (~5×104 cells/ml) were cultured in RPMI medium containing penicillin/streptomycin and 10% FBS at 37℃ in a water-saturated atmosphere of 95% air and 5% CO2. Treatments were initiated as outlined in Results.
The specimens (normal ovarian and tumor Stage IIIc tissues) in the study were obtained through the Cooperative Human Tissue Network (CHTN) and Institutional Review Board (IRB) approval at Vanderbilt University Medical Center.
C57BL6 mice (6 to 8-week-old, Charles River Laboratories, Wilmington, MA, USA) were given commercial pellet feed and drinking water ad libitum and housed with controlled 12-h light, 12-h dark cycle under pathogen-free conditions. All handling of animals and procedures were performed under institutional guidelines approved by the Institutional Animal Care and Use Committee at Meharry Medical College and adhere to the ARRIVE (Animal Research: Reporting In Vivo Experiments) guidelines for reporting animal research (16). LPS (100 µg/mouse) was injected intraperitoneally (ip) into the peritoneal cavity of mice and then the ovarian samples were collected at 2 h after LPS injection.
After isolating total RNA and eliminating genomic DNA, the expression of SAA1/2 and SAA4 were analyzed. Specific primers for SAA were designed as follows: 5'-TTT TCT GCT CCT TGG TCC TG-3' (sense) and 5'-TGG AAG TAT TTG TCT GAG CCG-3' (antisense) for human SAA1/2, 5'-CGT TCC AGG GTC TAT CTT CAG-3' (sense) and 5'-TTC CTC AGC TTT CTC GTT GG-3' (antisense) for human SAA4. For RT-PCR, the reverse transcriptase reaction conditions, using random primers with Moloney murine leukemia virus, were at 42 C for 60 min followed by 94 C for 10 min. PCR was performed under the following conditions: denaturation at 94 C for 1 min, annealing at 60 C for 1 min and extension at 74 C for 1 min with 30 cycles. Amplified PCR products were analyzed by electrophoresis in 2% agarose gels containing 1 µg ethidium bromide/ml. The fluorescent images were photographed under UV light.
For real-time PCR, reverse transcription reactions were performed at 42℃ for 15 min followed by 94℃ for 5 min. According to manufacturers' instructions, PCR was performed using a Bio-Rad CFX96 under the following two-step cycling program: 1 cycle at 95℃ for 10 min, 40 cycles at 95℃ for 15 sec and at 60o℃ for 1 min. Data analysis was performed using PCR Array Data Analysis Software (http://www.sabiosciences.com/RTPCR.php) provided by SABiosciences/Qiagen.
SAA1 (−490/+43) promoter was generated by PCR using primer sets as follows: 5'-GGG ATT ATA GGA GTG AGC CAC-3' for sense and 5'-CTC CTC ACC TGA TCT GTG CTG-3' for antisense. The PCR was performed for 35 cycles at 94 C for 1 min, 58 C for 1 min and 74 C for 1 min with a final extension at 74 C for 10 min. The amplified SAA1 DNA fragment was subcloned into pGEM-T easy vector. Deletion constructs of pGL4.12 Luciferase Reporter Vector were generated from the SAA1 DNA fragment in pGEM-T Easy Vector under the same PCR conditions using the following primers containing the XhoI and Hind III sites: 5'-TAA CTC GAG ATC TGC CAT GTG GCC CAG CAG-3' for SAA1-P401, 5'-TAA CTC GAG ACA CCT TCC AGC AGC CCA GGT-3' for SAA1-P319, 5'-GCA CTC GAG CCA GGA ACT TGT CTT AGA CCG-3' for SAA1-P139, and 5'-TAC CTC GAG CCA GGG ACC ACA TCC AGC TTT-3' for SAA1-P85. Common reversed primer sequence was 5'-CCG AAG CTT CTC CTC ACC TGA TCT GTG CTG-3' for SAA1+43. The constructs of the SAA1 promoter were confirmed by DNA sequencing analysis.
Data analysis was performed using microarray data sets deposited in the NCBI Gene Expression Omnibus (GEO, http://www.ncbi.nlm.nih.gov/geo/) database under accession number GSE51088. Raw microarray data for SAA and pro-inflammatory cytokine (IL-1α, IL-β, IL-6, TNF) expression levels were obtained from ovarian tissue samples in 13 normal ovarian tissues, 7 borderline ovarian tumor and 122 serous ovarian cancer. We employed Gitools 2.2.3 (http://www.gitools.org) based on Oracle Java 7, an open-source tool to perform Genomic Analysis and Visualization as interactive heat-maps (19).
Data values were expressed as the mean±SEM. Data were analyzed and compared using paired Student's t test and one-way ANOVA as appropriate. If statistical significance (p≤0.05) was determined by ANOVA, the data were further analyzed by Tukey's pairwise comparisons to detect specific differences between treatments.
First, we evaluated the expression pattern of SAA isoforms in ovarian epithelial normal and tumor tissues. Identities of mRNAs (M23698 vs. M23700) and protein (AAI05797 vs. NP_110381) for SAA1 and SAA2 are 97% and 93%, respectively, by Align Sequences Nucleotide and Protein BLAST (https://blast.ncbi.nlm.nih.gov). Identities of mRNAs (M23698 vs. M23700) and protein (AAI05797 vs. EAW68415) for SAA1 and SAA4 are 69% (no significant similarity in megablast, but 69% in discontinuous megablast) and 55%, respectively. Because of difficult identification due to the highly similar sequences between SAA1 and SAA2, here we described as SAA1/2. We did not evaluate SAA3 isoform in human samples because human SAA3 is a pseudogene, unlike mouse SAA3, which is a main isoform (20). SAA1/2 mRNA was highly expressed in five different ovarian cancers compared to normal ovarian tissue samples (Fig. 1A). However, low expression of SAA4 mRNA was detected in tumor tissues compared to SAA1/2 (Fig. 1B). The results from RT-PCR confirmed the higher expression of SAA1/2 and similar detectable expression of SAA4 in the tumor tissues compared to normal tissues (Fig. 1C). These results suggest that SAA1/2 is the dominant isoform induced in human ovarian tumor tissues rather than SAA4.
Next, we investigated the expression of TNF in ovarian normal vs. tumor tissues and the correlation between TNF and SAA isoforms. TNF is well known to be a proinflammatory cytokine abundantly expressed in ovarian cancer (91011). Interestingly, TNF is also a critical regulator for ovarian SAA (18,20). TNF was found to be highly upregulated in ovarian tumor tissues compared to normal tissues (Fig. 2A). Correlation between the expression levels of TNF and SAA isoforms revealed that SAA1/2 (R2=0.69) was more closely associated with TNF rather than SAA4 (R2=0.33) (Fig. 2B). Using TNF responsive ovarian cancer cells such as OVCAR-3 and SKOV-3 (6), we confirmed that TNF induced SAA1/2 mRNAs in a time-dependent manner (Fig. 2C). Because proinflammatory cytokines such as IL-1 and IL-6 were produced by ovarian tumors (10), these NF-κB activators also could augment ovarian SAA1/2 through NF-κB mediated signaling (1820). In addition, TNF mRNA was ~4.63 fold highly expressed compared to IL-1α in human ovarian tumor tissues (data not shown).
We investigated whether TNF regulates human SAA1 at the promoter levels because SAA3, the main isoform of SAA in mouse, is induced by TNF (1820). We generated a SAA 1 promoter containing NF-κB like and consensus sites (Fig. 3A). We found that TNF induced human SAA1 promoter activity and IkB overexpression blocked TNF-induced promoter activity (Fig. 3B). These results implicate NF-κB mediated signaling in regulating human SAA1. In spite of the lack of NF-κB consensus sites in mouse SAA3 promoter, NF-κB like sites played a critical role in regulating SAA3 (1820). Using deletion constructs, we examined which NF-κB site is critical for TNF-induced SAA1 promoter activity. SAA1-P401, P319 and P139 deletions were induced by TNF, whereas P85 without NF-κB site was not induced (Fig. 3C). These results indicate that the proximal NF-κB consensus site (−95/−86) is critical in regulating human SAA1.
Finally, because EOC is the most common type in ovarian cancer (2), we evaluated whether NF-κB activation induces the expression of SAA in ovarian epithelial cells exposed to LPS (21), a product known to stimulate release of TNF through toll-like receptors (TLRs), in mice. We injected LPS or vehicle (saline) to mice, collected the ovaries and assessed ovarian localization of SAA3 by in situ hybridization because SAA3 is a main isoform in mice (20). SAA3 mRNA was preferably localized in the ovarian epithelial cells and the thecal-interstitial layers as compared to granulosal cell layers (Fig. 4A). These results indicate that NF-κB signaling induces ovarian localization of SAA3 in ovarian epithelial cells.
Based on the NCBI GEO database (GSE51088), we compared expression levels of SAA1/2, SAA4, IL-1α, IL-1β, IL-6 and TNF in normal ovarian tissues (NOT), borderline ovarian tumor (BOT) and serous ovarian cancer (SOC). Along with TNF and LPS, IL-1β and IL-6 are also well-known NF-κB activators (21). Because the correlation coefficient in expression levels between SAA1 and SAA2 is 0.98, we described SAA1/2 together. SAA1/2, IL-1β and TNF were significantly expressed in SOC compared to NOT and BOT (Fig. 4B). The surface epithelium cells adjacent to the site of ovulation were associated with an increased risk of malignant transformation (10). Proinflammatory cytokines such as TNF, IL-1β and IL-6, produced by tumor itself and/or activated immune cells, have been shown to promote ovarian tumor growth and progression (45). Our results indicate that TNF is a key regulator of SAA1/2 via the NF-κB signaling pathway in EOC. A previous study showed that the expression of SAA in human EOC gradually increased during the progress through benign and borderline adenomas to primary and metastatic adenocarcinomas (15). In addition, high serum levels of SAA were found in patients with ovarian carcinoma, and the SAA levels are correlated strongly with the ovarian tumor marker CA-125 (15). Our analysis of the GEO dataset (Fig. 4B) support these previous results. The high expression of SAA1/2 via NF-κB mediated signaling in human EOC may have prognostic and therapeutic applications. In conclusion, the accumulation of SAA1/2 in EOC could be mediated by NF-κB activation through TNF, requiring further study regarding the functional role of SAA in EOC.
Figures and Tables
Figure 1
SAA1/2 is a dominant isoform in human ovarian tumor tissues. (A) Expression levels of SAA1/2 mRNA determined by real-time PCR in five different ovarian normal (N1-N5) and tumor tissues (T1-T5). (B) Expression levels of SAA4 mRNA in normal ovarian tissues and tumor specimens determined by real-time PCR. (C) Visual confirmation of SAA1/2 and SAA4 expression by RT-PCR. After isolating total RNA, RT-PCR and real-time PCR were carried out using primers for SAA1/2 and SAA4. GAPDH was used as a loading control.
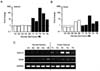
Figure 2
TNF enhances SAA1/2 expression in ovarian cancer. (A) Expression levels of TNF mRNA determined by real-time PCR in five different ovarian normal (N1-N5) and tumor tissues (T1-T5). (B) Correlation between mRNA levels of TNF and SAA isoforms. (C) Time-dependent effects of TNF on SAA1/2 mRNA by real-time PCR in ovarian cancer cell lines (OVCAR-3 and SKOV-3). Cells were incubated with TNF (10 ng/ml) for 0, 1, 3, 6, 12 and 24 hours. *Indicates significant increase (p≤0.05) compared to its own control as calculated by the paired Student's t test.
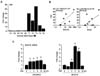
Figure 3
TNF increases SAA1 promoter activity via NF-κB signaling. (A) DNA sequences of the human SAA1 promoter. (B) Effect of TNF and IκB on luciferase activity of SAA1 promoter in SKOV-3 cells. Results were normalized to the protein level and represented as a fold increase compared to empty vector (pA) controls. (C) Effects of TNF on luciferase activity of the SAA1 promoter with NF-κB site deletions. After SKOV-3 cells were transfected with SAA1 luciferase vectors overnight, cells were treated with TNF (10 ng/ml) for 6 h, followed by luciferase assay. Results were normalized to the protein level and represented as a fold increase compared to non-treated control. Grey and white colors indicate NF-κB like (lower case for unmatched DNA sequence) and consensus sites, respectively. *Indicates significant increase (p≤0.05) compared to its own control as calculated by the paired Student's t test.
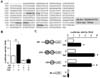
Figure 4
Ovarian localization of SAA after LPS treatment in mice, and GEO profiles of SAAs and proinflammatory cytokines in human ovarian tumor tissues. (A) LPS-induced SAA3 accumulation in ovarian epithelial and thecal-interstitial layers of mouse ovary. Six week-old mice were given vehicle (saline) or LPS (100 µg/mouse, ip). Ovaries were collected at 2 hrs after LPS treatment and ovarian localization of SAA3 mRNA was investigated by using in situ hybridization with a DIG-labeled RNA probe. Sense probe of SAA did not express any signal (data not shown). (B) GEO profiles of SAAs and proinflammatory cytokines in normal ovarian tissues (NOT), benign ovarian tumor (BOT) and serous ovarian cancer (SOC) based on the NCBI GEO database (GSE51088). Data values were expressed as the mean±SEM. *indicate significantly higher (p≤0.05) within groups as analyzed by Tukey's pairwise comparisons.
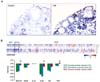
ACKNOWLEDGEMENTS
This research was supported, in whole or in part, by National Institutes of Health as the following grants: NIGMS SC1 089630 and R01ES024756 (E.L.), NCATS UL1TR000445 (A.W.), and NIAID SC1AI089073, NCI SC1CA200519 and U54CA163069 (D.S.). Its contents are solely the responsibility of the authors and do not necessarily represent the official views of NIH.
References
2. Shih IM, Kurman RJ. Ovarian tumorigenesis: a proposed model based on morphological and molecular genetic analysis. Am J Pathol. 2004; 164:1511–1518.
3. Kobel M, Kalloger SE, Boyd N, McKinney S, Mehl E, Palmer C, Leung S, Bowen NJ, Ionescu DN, Rajput A, Prentice LM, Miller D, Santos J, Swenerton K, Gilks CB, Huntsman D. Ovarian carcinoma subtypes are different diseases: implications for biomarker studies. PLoS Med. 2008; 5:e232.


4. Nowak M, Glowacka E, Szpakowski M, Szyllo K, Malinowski A, Kulig A, Tchorzewski H, Wilczynski J. Proinflammatory and immunosuppressive serum, ascites and cyst fluid cytokines in patients with early and advanced ovarian cancer and benign ovarian tumors. Neuro Endocrinol Lett. 2010; 31:375–383.
5. Clendenen TV, Lundin E, Zeleniuch-Jacquotte A, Koenig KL, Berrino F, Lukanova A, Lokshin AE, Idahl A, Ohlson N, Hallmans G, Krogh V, Sieri S, Muti P, Marrangoni A, Nolen BM, Liu M, Shore RE, Arslan AA. Circulating inflammation markers and risk of epithelial ovarian cancer. Cancer Epidemiol Biomarkers Prev. 2011; 20:799–810.


6. Son DS, Kabir SM, Dong Y, Lee E, Adunyah SE. Characteristics of chemokine signatures elicited by EGF and TNF in ovarian cancer cells. J Inflamm (Lond). 2013; 10:25.


7. Ignacio RM, Kabir SM, Lee ES, Adunyah SE, Son DS. NF-kappaB-mediated CCL20 reigns dominantly in CXCR2-driven ovarian cancer progression. PLoS One. 2016; 11:e0164189.
8. Dong YL, Kabir SM, Lee ES, Son DS. CXCR2-driven ovarian cancer progression involves upregulation of proinflammatory chemokines by potentiating NF-kappaB activation via EGFR-transactivated Akt signaling. PLoS One. 2013; 8:e83789.
9. Dobrzycka B, Terlikowski SJ, Kowalczuk O, Kinalski M. Circulating levels of TNF-alpha and its soluble receptors in the plasma of patients with epithelial ovarian cancer. Eur Cytokine Netw. 2009; 20:131–134.


11. Szlosarek PW, Grimshaw MJ, Kulbe H, Wilson JL, Wilbanks GD, Burke F, Balkwill FR. Expression and regulation of tumor necrosis factor alpha in normal and malignant ovarian epithelium. Mol Cancer Ther. 2006; 5:382–390.


12. Urieli-Shoval S, Linke RP, Matzner Y. Expression and function of serum amyloid A, a major acute-phase protein, in normal and disease states. Curr Opin Hematol. 2000; 7:64–69.


13. Targonska-Stepniak B, Majdan M. Serum amyloid A as a marker of persistent inflammation and an indicator of cardiovascular and renal involvement in patients with rheumatoid arthritis. Mediators Inflamm. 2014; 2014:793628.
14. Thorn CF, Lu ZY, Whitehead AS. Regulation of the human acute phase serum amyloid A genes by tumour necrosis factor-alpha, interleukin-6 and glucocorticoids in hepatic and epithelial cell lines. Scand J Immunol. 2004; 59:152–158.


15. Urieli-Shoval S, Finci-Yeheskel Z, Dishon S, Galinsky D, Linke RP, Ariel I, Levin M, Ben-Shachar I, Prus D. Expression of serum amyloid a in human ovarian epithelial tumors: implication for a role in ovarian tumorigenesis. J Histochem Cytochem. 2010; 58:1015–1023.


16. Kilkenny C, Browne WJ, Cuthill IC, Emerson M, Altman DG. Improving bioscience research reporting: the ARRIVE guidelines for reporting animal research. PLoS Biol. 2010; 8:e1000412.


17. Son DS, Roby KF. Interleukin-1alpha-induced chemokines in mouse granulosa cells: impact on keratinocyte chemoattractant chemokine, a CXC subfamily. Mol Endocrinol. 2006; 20:2999–3013.


18. Son DS, Terranova PF, Roby KF. Interaction of adenosine 3',5'-cyclic monophosphate and tumor necrosis factor-alpha on serum amyloid A3 expression in mouse granulosa cells: dependence on CCAAT-enhancing binding protein-beta isoform. Endocrinology. 2010; 151:3407–3419.


19. Perez-Llamas C, Lopez-Bigas N. Gitools: analysis and visualisation of genomic data using interactive heat-maps. PLoS One. 2011; 6:e19541.


20. Son DS, Roby KF, Terranova PF. Tumor necrosis factor-alpha induces serum amyloid A3 in mouse granulosa cells. Endocrinology. 2004; 145:2245–2252.


21. Hoesel B, Schmid JA. The complexity of NF-kappaB signaling in inflammation and cancer. Mol Cancer. 2013; 12:86.