Abstract
The pathophysiology of glandular dysfunction in Sjögren's syndrome (SS) has not been fully elucidated. Previously, we reported the presence of autoantibodies to AQP-5 in patients with SS, which was associated with a low resting salivary flow. The purpose of this study was to investigate the presence of anti-AQP1 autoantibodies. To detect anti-AQP1 autoantibodies, cell-based indirect immunofluorescence assay was developed using MDCK cells that overexpressed human AQP1. By screening 112 SS and 52 control sera, anti-AQP1 autoantibodies were detected in 27.7% of the SS but in none of the control sera. Interestingly, the sera that were positive for anti-AQP1 autoantibodies also contained anti-AQP5 autoantibodies in the previous study. Different from anti-AQP5 autoantibodies, the presence of anti-AQP1 autoantibodies was not associated with the salivary flow rate. Although anti-AQP1 autoantibodies are not useful as a diagnostic marker, the presence of autoantibodies to AQP1 may be an obstacle to AQP1 gene therapy for SS.
Sjögren's syndrome (SS) is a chronic autoimmune disease that primarily targets the lacrimal and salivary glands. Thus, the chief clinical manifestation of SS is dryness in the mouth and eyes (1). The mechanism for glandular dysfunction includes glandular atrophy that involves the inflammation and infiltration of cytotoxic T cells into the salivary and lacrimal glands (2). Despite the focal appearance of T cells in the salivary gland, however, B cell–deficient NOD.Igµnull mice required transfer of purified IgG from patients with SS to develop the loss of secretory function, indicating the role of autoantibodies in glandular dysfunction in SS (3). Although autoantibodies to type 3 muscarinic acetylcholine receptors identified in SS can interfere with the secretory process (45), the pathophysiology of glandular dysfunction in SS has not yet been fully elucidated.
The salivary glands produce saliva in two steps. First, acinar cells secrete Cl- from the interstitial fluid into the acinar lumen, involving several ion transporters. Through osmosis, salt accumulation allows water outflow through aquaporin (AQP)-5 into the lumen to produce a plasmalike isotonic fluid. In the second step, ductal epithelial cells reabsorb most of the NaCl and make the final saliva hypotonic (678). Normal salivary production by the acinar cells depends not only on water outflow through the AQP5 expressed on the apical membrane, but also on water inflow into the acinar cells through the AQP3 expressed on the basolateral membrane (9). Acinar cells are surrounded by myoepithelial cells and blood vessels (10). AQP1 is a major water channel protein expressed on myoepithelial and endothelial cells that surround acinar cells, through which water outflow occurs (1112).
AQP5 plays an important role in salivary production, as seen in AQP5-deficient mice (13). Although AQP1-deficient mice experience no defects in salivary volume or composition (8), the expression of AQP1, but not AQP3, is downregulated in the salivary glands of patients with SS (9). Furthermore, B-cell depletion with rituximab increased both the AQP1 expression in myoepithelial cells and salivary flow rate in patients with SS (14). In addition, AQP1 was selected for gene therapy because of its expression in a polarity-independent manner (15), and adenoviral-mediated transfer of AQP1 cDNA significantly increased the salivary flow rate in irradiated rats, human subjects with radiation-induced salivary hypofunction, and a murine model of SS (1617).
We recently identified anti-AQP5 autoantibodies in the sera of SS patients, the presence of which was associated with a low resting salivary flow rate (18). Because AQP1 is relatively closely related to AQP5 in the phylogenic tree of 12 human AQPs (supplementary Fig. 1A), we hypothesized that SS patients may also have autoantibodies to AQP1. The aim of this study was to investigate the potential presence of autoantibodies to AQP1 in the sera of patients with SS.
This study was performed in compliance with the Helsinki Declaration after obtaining approvals from the Institutional Review Board of Seoul National University Hospital (IRB Number: 0912-011-302), the Institutional Review Board of Seoul National University School of Dentistry (IRB Number: S-D20140022), and the Institutional Review Board of Seoul St. Mary's Hospital (IRB Number: KC13ONMI0646). The clinical data and samples were obtained after obtaining written informed consent. The same serum samples that were used in our previous study involving anti-AQP5 autoantibodies (18) were used in this study. Serum samples were obtained from 112 primary SS patients who were diagnosed according to the 2002 American-European Consensus group (AECG) classification criteria (19) and/or the 2012 American College of Rheumatology (ACR) criteria (20). In addition, resting and stimulated whole salivary flow rates were measured by spiting and masticatory stimuli using wax gum (GC America Inc, St. Alsip, IL, USA), respectively, according to the method previously described (21). All patients were females with ages ranging from 21 to 80 years (mean age 52.5±10.7 years). Control sera were obtained from 53 healthy female controls who did not show any signs or symptoms of SS (mean age 37.1±7.4 years). Subjects with systemic diseases other than hypertension were excluded.
Total RNA from whole blood was extracted using an easy-BLUE™ total RNA extraction kit (iNtRON Biotechnology, Sungnam, Gyeonggi, Korea). Complementary DNA (cDNA) was synthesized from 1 µg of total RNA using an oligo-d (T) primer and M-MLV reverse transcriptase enzyme (Promega, Madison, WI, USA) in a 25 µl reaction mix. Full-length human AQP1 was amplified using forward (5′-GGATCCATGGCCAGCGAGTTCAAGA AGAAGC-3′) and reverse (5′-CTCGAGCTATTTGG GCTTCATCTCCACCC-3′) primers. The PCR product was first cloned into a cloning TA vector (Real Biotech Corporation, Banquiao, Taiwan) and then sub-cloned into a pcDNA3.1 vector using the BamHI and XhoI restriction sites (Invitrogen, Carlsbad, CA, USA). MDCK cells (Korean Cell Line Bank, Seoul, Korea) were transfected with the cloned plasmid using a calcium phosphate precipitation method and maintained in the presence of G418 (22).
A standard cell-based indirect immunofluorescence assay to detect AQP1 was developed as previously described (182324). MDCK cells expressing full-length human AQP1 were fixed with 4% paraformaldehyde in PBS (pH 7.4) and subjected to antigen retrieval by incubation in sodium citrate buffer (pH 6) at 105℃ for 20 min. After permeabilization with 0.3% Triton X-100 and blocking with 5% BSA in PBS, the cells were incubated with a rabbit polyclonal antibody raised against amino acids 215~269 of human AQP1 (Santa Cruz, Paso Robles, CA, USA) along with a control or a SS serum overnight, followed by Alexa Fluor 488-conjugated goat anti-rabbit IgG and Alexa Fluor 555-conjugated goat anti-human IgG (Invitrogen). To detect anti-AQP1 IgA, goat antihuman IgA TXRD (Southern Biotech, Birmingham, AL, USA) was used as a secondary antibody. All images were taken with a confocal microscope LSM 700 (Carl Zeiss, Jena, Germany). Three images of AQP1-expressing cells were randomly selected and sequentially imaged for the presence of anti-AQP1 IgG or IgA. The relative intensities of the anti-AQP1 signals were determined by decreasing the brightness of the red signal until the anti-AQP1 IgG/IgA signals disappeared. The mean brightness obtained from the three images was used to express the anti-AQP1 IgG/IgA level for each sample.
Student's t-test was used to determine differences in Mander's coefficient between the control and SS sera. Correlation between the anti-AQP1 and anti-AQP5 autoantibody intensities was determined using a Pearson correlation analysis. Associations between the salivary flow rates and the presence of anti-AQP1 autoantibodies were examined using one-way analysis of variance (ANOVA). Because some groups did not pass the normality test, the difference was also analyzed using the Mann–Whitney U test. All statistics were performed using SPSS (SPSS Inc., Chicago, USA).
To detect anti-AQP1 autoantibodies in sera, MDCK cells overexpressing full-length human AQP1 were double stained with anti-AQP1 antibodies and sera from either control subjects (n=52) or SS patients (n=112). To compare the prevalence of anti-AQP1 autoantibodies with that of anti-AQP5 autoantibodies, the same dilutions of sera were used for screening in the previous and current studies. In the previous study, the lowest dilution that did not stain AQP5 by pooled control sera was chosen for screening (18). By screening sera diluted to 1:200 for the presence of anti-AQP1 IgG, autoantibodies were detected in 23 patients with SS (20.5%), and the sera with anti-AQP1 IgG showed a high degree of colocalization between the signals of human IgG and those of AQP1-specific rabbit IgG. None of the control sera was positive for anti-AQP1 IgG (Fig. 1A and B). Among the anti-AQP1 IgG-positive SS sera, 19 samples were also positive for anti-AQP5 IgG in the previous study (18), and in the other four samples, the anti-AQP5 IgG was also detected but at lower levels than the cut off used for positivity (Supplementary Table I). For several anti-AQP1 IgG-negative but anti-AQP5 IgG-positive sera (n=7 for each control and SS), the presence of anti-AQP1 IgG was additionally screened using 1:50 dilution, but all samples were still negative for anti-AQP1 IgG.
Interestingly, anti-AQP1 autoantibodies were not detected in any control sera, although 32.1% of them were positive for anti-AQP5 IgG in the previous study (18). Even though the control sera were negative for anti-AQP1 autoantibodies by indirect immunofluorescence assay, not only the SS but also control sera immunoprecipitated the AQP1–GFP from the lysates of the HEK-293 cells transfected with a gene construct encoding the AQP1-GFP (data not shown). Similarly, immunoprecipitation was not effective in differentiating the levels of anti-AQP5 autoantibodies between the control and patient samples (18).
By screening sera diluted to 1:20 for the presence of anti-AQP1 IgA, autoantibodies were detected in eight SS samples (7.1%) but in none of the control sera (Fig. 1C and D). All anti-AQP1 IgA-positive sera were also positive for anti-AQP5 IgA and/or IgG. However, none of the samples with anti-AQP1 IgA were positive for anti-AQP1 IgG (Supplementary Table I).
In contrast to anti-AQP5 autoantibodies, for which the presence of anti-AQP5 IgA had overlapped with the presence of anti-AQP5 IgG in the previous study (18), the presence of anti-AQP1 IgA coincided with the presence of anti-AQP5 IgA, rather than the presence of anti-AQP1 IgG. Similarly, the presence of anti-AQP1 IgG overlapped with the presence of anti-AQP5 IgG. Therefore, anti-AQP1 autoantibodies may be cross-reactive antibodies produced during antibody response to AQP5. A significant positive correlation (p=0.003) between the intensities of the anti-AQP1 IgG/IgA and anti-AQP5 IgG/IgA supports it (Fig. 1E). The amino acid sequence of AQP1 has 44% identity and 62% similarity with AQP5. In contrast to AQP5 in which the full lengths of loops B and E are exposed to the cytosolic and extracellular compartments, respectively, the middle portion of loops B and E in AQP1 is embedded within the membrane (Fig. 2). However, short stretches of conserved sequences were observed in loop B, loop D, and loop E, as well as the C-terminal cytoplasmic tail, which may provide cross-reactive epitopes.
Alternatively, both autoantibodies against AQP5 and AQP1 might have been developed during an immune response against bacterial proteins that are homologous to human AQPs. Previously, we reported a high degree of homology between AQP5 and AQPZ or porins from many human-associated bacteria, including the members of normal human oral flora (18). The same oral bacterial AQPs presented 35~42% identity and 52~56% similarity with AQP1 (Fig. 3). Whereas the sequences of the bacterial AQPs are conserved mostly at the transmembrane alpha helixes and the pore-forming loops B and E of AQP5 (18), conserved sequences were observed mostly at the transmembrane alpha helixes and the loops B and C of AQP1 (Fig. 3). Epitope mapping for anti-AQP5 and anti-AQP1 autoantibodies in a future study would help clarify the relationship of these autoantibodies to each other.
Associations between the presence of anti-AQP1 IgG or IgA and salivary flow rates were analyzed in patients who had their saliva flow rate recorded at the time of serum sampling. The presence of neither anti-AQP1 IgG nor IgA had a significant effect on salivary flow. Because more than one-half of the SS patients who were negative for anti-AQP1 autoantibodies had anti-AQP5 autoantibodies that were associated with a low resting salivary flow (18), the effect of anti-AQP1 autoantibodies on salivary flow was also examined in patients with anti-AQP5 autoantibodies. However, no difference in either resting or stimulatory salivary flow was observed by the additional presence of anti-AQP1 autoantibodies (Table I).
Two-thirds of the anti-AQP1 autoantibodies detected in patients with suspected neuromyelitis optica spectrum disorders were bound to the extracellular domain of AQP1 (25). If the anti-AQP1 autoantibodies detected in SS patients bind to the AQP1 expressed on myoepithelial cells and endothelial cells in vivo, antibodies may block water flow across these cells, interfering with the inflow of water to the acini. However, the presence of anti-AQP1 autoantibodies did not affect either resting or stimulated salivary flow. This supports the notion that AQP1 does not play a major role in normal physiology of salivary secretion, as observed in AQP1-deficient mice (8).
In conclusion, anti-AQP1 autoantibodies were detected in 27.7% of SS patients. Although anti-AQP1 autoantibodies are not useful as a diagnostic marker because of their low sensitivity, the presence of autoantibodies to AQP1 might become a major obstacle to AQP1 gene therapy for SS.
Figures and Tables
Figure 1
Detection of anti-AQP1 IgG and IgA in the sera of SS patients. (A) MDCK cells over-expressing AQP1 were stained with anti-AQP1 antibodies and either control or SS sera (1:200 dilution), followed by Alexa Fluor 488-conjugated anti-goat IgG (green) and CF™ 594-conjugated anti-human IgG (red). The colocalization of green and red signals in the three images was calculated using Mander's coefficient. *p=0.0001 (B) The intensities of the red signals for anti-AQP1 IgG were expressed by the magnitude of brightness that was reduced until the AQP1 staining disappeared. (C) MDCK cells over-expressing AQP1 were stained with anti-AQP1 antibodies and either control or SS sera (1:20 dilution), followed by Alexa Fluor 488-conjugated anti-goat IgG (green) and Alexa Fluor 555-conjugated anti-human IgA (red). The colocalization of green and red signals in the three images was calculated using Mander's coefficient. *p=0.02 (D) The intensities of the red signals for anti-AQP5 IgA were expressed by the magnitude of brightness that was reduced until the AQP1 staining disappeared. (E) The intensities of anti-AQP1 IgG or IgA and of anti-AQP5 IgG or IgA that were identified in each individual were plotted.
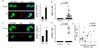
Figure 2
Sequence alignment of AQP5 and AQP1. Identical and conserved amino acids are highlighted with dark gray and light gray, respectively. The extracellular and cytosolic domains are boxed with solid and dotted lines, respectively.
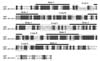
Figure 3
Sequence alignment of AQP1 and bacterial AQPs. Identical and conserved amino acids are highlighted with dark gray and light gray, respectively. The extracellular and cytosolic domains are boxed with solid and dotted lines, respectively.
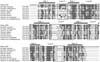
Table I
Association between autoantibodies to AQP1 and salivary flow rates
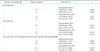
ACKNOWLEDGEMENTS
This study was supported by a grant of the Korean Health Technology R&D Project (HI13C0016) from Ministry of Health & Welfare, Republic of Korea.
References
1. Luciano N, Valentini V, Calabro A, Elefante E, Vitale A, Baldini C, Bartoloni E. One year in review 2015: Sjogren's syndrome. Clin Exp Rheumatol. 2015; 33:259–271.
2. Fox RI. Sjogren's syndrome. Lancet. 2005; 366:321–331.
3. Robinson CP, Brayer J, Yamachika S, Esch TR, Peck AB, Stewart CA, Peen E, Jonsson R, Humphreys-Beher MG. Transfer of human serum IgG to nonobese diabetic Igmu null mice reveals a role for autoantibodies in the loss of secretory function of exocrine tissues in Sjogren's syndrome. Proc Natl Acad Sci U S A. 1998; 95:7538–7543.


4. Smith PM, Dawson LJ. Autoantibodies and Sjogren's syndrome: a physiologist's perspective. Curr Pharm Biotechnol. 2012; 13:2063–2070.


5. Lee BH, Gauna AE, Perez G, Park YJ, Pauley KM, Kawai T, Cha S. Autoantibodies against muscarinic type 3 receptor in Sjogren's syndrome inhibit aquaporin 5 trafficking. PLoS One. 2013; 8:e53113.
6. Proctor GB, Carpenter GH. Salivary secretion: mechanism and neural regulation. Monogr Oral Sci. 2014; 24:14–29.


7. Catalan MA, Nakamoto T, Melvin JE. The salivary gland fluid secretion mechanism. J Med Invest. 2009; 56:Suppl. 192–196.


8. Delporte C, Bryla A, Perret J. Aquaporins in Salivary Glands: From Basic Research to Clinical Applications. Int J Mol Sci. 2016; 17:E166.


9. Beroukas D, Hiscock J, Gannon BJ, Jonsson R, Gordon TP, Waterman SA. Selective down-regulation of aquaporin-1 in salivary glands in primary Sjogren's syndrome. Lab Invest. 2002; 82:1547–1552.


11. Mobasheri A, Marples D. Expression of the AQP-1 water channel in normal human tissues: a semiquantitative study using tissue microarray technology. Am J Physiol Cell Physiol. 2004; 286:C529–C537.


12. Gresz V, Horvath A, Gera I, Nielsen S, Zelles T. Immunolocalization of AQP5 in resting and stimulated normal labial glands and in Sjogren's syndrome. Oral Dis. 2015; 21:e114–e120.
13. Ma T, Song Y, Gillespie A, Carlson EJ, Epstein CJ, Verkman AS. Defective secretion of saliva in transgenic mice lacking aquaporin-5 water channels. J Biol Chem. 1999; 274:20071–20074.


14. Ring T, Kallenbach M, Praetorius J, Nielsen S, Melgaard B. Successful treatment of a patient with primary Sjogren's syndrome with Rituximab. Clin Rheumatol. 2006; 25:891–894.


15. Lai Z, Yin H, Cabrera-Perez J, Guimaro MC, Afione S, Michael DG, Glenton P, Patel A, Swaim WD, Zheng C, Nguyen CQ, Nyberg F, Chiorini JA. Aquaporin gene therapy corrects Sjogren's syndrome phenotype in mice. Proc Natl Acad Sci U S A. 2016; 113:5694–5699.
16. Delporte C, O'Connell BC, He X, Lancaster HE, O'Connell AC, Agre P, Baum BJ. Increased fluid secretion after adenoviral-mediated transfer of the aquaporin-1 cDNA to irradiated rat salivary glands. Proc Natl Acad Sci U S A. 1997; 94:3268–3273.


17. Baum BJ, Alevizos I, Zheng C, Cotrim AP, Liu S, McCullagh L, Goldsmith CM, Burbelo PD, Citrin DE, Mitchell JB, Nottingham LK, Rudy SF, Van WC, Whatley MA, Brahim JS, Chiorini JA, Danielides S, Turner RJ, Patronas NJ, Chen CC, Nikolov NP, Illei GG. Early responses to adenoviral-mediated transfer of the aquaporin-1 cDNA for radiation-induced salivary hypofunction. Proc Natl Acad Sci U S A. 2012; 109:19403–19407.


18. Alam J, Koh JH, Kim N, Kwok SK, Park SH, Song YW, Park K, Choi Y. Detection of autoantibodies against aquaporin-5 in the sera of patients with primary Sjogren's syndrome. Immunol Res. 2016; 64:848–856.


19. Vitali C, Bombardieri S, Jonsson R, Moutsopoulos HM, Alexander EL, Carsons SE, Daniels TE, Fox PC, Fox RI, Kassan SS, Pillemer SR, Talal N, Weisman MH. Classification criteria for Sjogren's syndrome: a revised version of the European criteria proposed by the American-European Consensus Group. Ann Rheum Dis. 2002; 61:554–558.


20. Shiboski SC, Shiboski CH, Criswell L, Baer A, Challacombe S, Lanfranchi H, Schiodt M, Umehara H, Vivino F, Zhao Y, Dong Y, Greenspan D, Heidenreich AM, Helin P, Kirkham B, Kitagawa K, Larkin G, Li M, Lietman T, Lindegaard J, McNamara N, Sack K, Shirlaw P, Sugai S, Vollenweider C, Whitcher J, Wu A, Zhang S, Zhang W, Greenspan J, Daniels T. American college of rheumatology classification criteria for Sjogren's syndrome: a data-driven, expert consensus approach in the Sjogren's International Collaborative Clinical Alliance cohort. Arthritis Care Res(Hoboken). 2012; 64:475–487.


21. Navazesh M, Christensen CM. A comparison of whole mouth resting and stimulated salivary measurement procedures. J Dent Res. 1982; 61:1158–1162.


22. Kingston RE, Chen CA, Okayama H. Calcium phosphate transfection. In : Bonifacino JS, Harford JB, Lippincott-Schwartz J, Yamada KM, Dasso M, Nadkarni A, editors. Current protocols in cell biology. John Wiley and sons, Inc.;2003. p. 19p. 20.3p. 20.3.1–20.3.8.


23. Jarius S, Probst C, Borowski K, Franciotta D, Wildemann B, Stoecker W, Wandinger KP. Standardized method for the detection of antibodies to aquaporin-4 based on a highly sensitive immunofluorescence assay employing recombinant target antigen. J Neurol Sci. 2010; 291:52–56.

