Abstract
Dendritic cells (DCs) are professional antigen-presenting cells (APCs) that bridge innate and adaptive immune responses, thereby leading to immune activation. DCs have been known to recognize pathogen-associated molecular patterns such as lipopolysaccharides (LPS) and nucleic acids via their pattern recognition receptors, which trigger signaling of their maturation and effector functions. Furthermore, DCs take up and process antigens as a form of peptide loaded on the major histocompatibility complex (MHC) and present them to T cells, which are responsible for the adaptive immune response. Conversely, DCs can also play a role in inducing immune suppression under specific circumstances. From this perspective, the role of DCs is related to tolerance rather than immunity. Immunologists refer to these special DCs as tolerogenic DCs (tolDCs). However, the definition of tolDCs is controversial, and there is limited information on their development and characteristics. In this review, we discuss the current concept of tolDCs, cutting-edge methods for generating tolDCs in vitro, and future applications of tolDCs, including clinical use.
Dendritic cells (DCs) mediate innate and adaptive immunity of the immune system. A major role of DCs is to present antigens to T cells. As professional antigen-presenting cells (APCs), DCs activate T cells through co-stimulatory molecules such as CD80 and CD86. These are the classical immunogenic characteristics of DCs. In contrast, tolerogenic DCs (tolDCs) contribute to tolerance rather than immunity. TolDCs can induce T cell anergy and generate regulatory T (Treg) cells. TolDCs-mediated immune suppressive responses are achieved by the interaction between co-inhibitory molecules like programmed death ligand 1 (PD-L1) and ligand 2 (PD-L2) on DCs and programmed death 1 (PD-1) on T cells. In addition to these surface molecules, anti-inflammatory cytokines such as interleukin-10 (IL-10) and transforming growth factor beta (TGF-β) from DCs can promote tolerance. How are tolDCs generated or induced and what are the factors involved in this process? It is necessary to first distinguish tolDCs from immature DCs (imDCs). The capacity of imDCs for inducing an immune response is inferior to that of mature DCs (mDCs) because imDCs express a lower frequency of MHC and co-stimulatory molecules on their surface than mature DCs. In addition, imDCs have no ability to secrete pro-inflammatory cytokines such as IL-6 and IL-12 (1). Because of their immature phenotypes, imDCs have limited ability to prime T cells and induce an immune response and thus have been considered tolDCs. However, the phenotype and definition of tolDCs remain controversial.
Here, we define the typical phenotype and the characteristic of tolDCs based on two perspectives: expression of regulatory molecules and secretion of cytokines. In this sequence, we will sum up the various factors that have been widely known to induce tolDCs in vitro. Finally, the importance of tolDCs in clinical trials and their therapeutic application will be discussed in this review.
CD80 and CD86 on the surface of DCs can interact with CD28 on T cells. The interaction can trigger a signal to stimulate a T cell immune response. Therefore, DCs expressing a low level of co-stimulatory molecules may have a decided tolerance advantage.
On the contrary, surface inhibitory molecules such as PD-L1 and PD-L2 on DCs interact with PD-1 on T cells. The interaction between molecules results in anergy or functional inactivation of T cells (123). Although it has been reported that overexpression of PD-L1 or PD-L2 on DCs did not inhibit proliferation of T cells (4), high expression levels of PD-L1 or PD-L2 on the surface of DCs can still be a phenotypical characteristic of tolDCs, as shown in many individual studies (45678910111213). The expression of another death signal molecule CD95, a FAS-ligand, can also be a sign of tolerance (1415).
In addition to the expression of surface molecules, a profile of cytokines secreted from DCs can represent the tolerogenic potential. The pro-inflammatory cytokine IL-12 produced by DCs stimulates the growth and function of T cells (16). Moreover, IL-12 can stimulate the secretion of interferon-gamma (IFN-γ) or tumor necrosis factor alpha (TNF-α) and can activate natural killer (NK) cells (16). Therefore, DCs that have a low ability to secrete such inflammatory cytokines can have 'tolerogenic potential'. DCs that secrete a high dose of anti-inflammatory cytokines such as IL-10 and TGF-β can also be considered tolDCs because IL-10 inhibits the synthesis and induction of pro-inflammatory cytokines such as IL-12, TNF-α, and IFN-γ (171819). Furthermore, IL-10 impairs the activation of NK and T cells (2021), and TGF-β is important for the maintenance and survival of Treg cells (22).
It has been reported that the direct effect of IL-12 on DCs is to promote nuclear localization of nuclear factor kappa- light-chain-enhancer of activated B cells (NF-κB) (23). Therefore, a low expression level of NF-κB, which is widely related to the activation of DCs, could be another sign of tolerogenic characteristics of tolDCs.
Indoleamine 2,3-dioxygenase (IDO), an immune checkpoint molecule produced by tolDCs, is also related to the inhibition of T cell proliferation and survival (2425262728). Moreover, IDO has been reported to promote functional development of Treg cells (29). Thus, the induction or up-regulation of IDO in DCs can tolerate the immune environment of the host.
In summary, tolerogenic potential in DCs is indicated by the presence of down-regulation of factors related to boosting the immune response and up-regulation of inhibitory molecules, anti-inflammatory cytokines, and related enzymes (Fig. 1).
It is necessary to know the factors that are responsible for the induction of DCs with tolerogenic characteristics. Because of the scarcity of DCs and their various subsets in vivo, many in vitro DC studies have been performed. In a murine system, the classic method of investigating DCs is using bone marrow-derived DCs (BMDCs). BMDCs can be generated by 6~10 days culture in vitro (3031). In this system, DCs are differentiated from bone marrow stem cells, and the culture medium must contain granulocyte-macrophage colony-stimulating factor (GM-CSF) (32). After reaching one-third of the total culture period, fresh medium is added at the same volume as the pre-existing medium. After reaching two-thirds of the total culture period, half of the culture medium is replaced with fresh medium. At the end of the culture period, BMDCs can be harvested by collecting suspended cells. These harvested 'untouched BMDCs' are imDCs. Additional treatment of LPS, TNF-α, or IFN-γ causes imDCs to become fully mature DCs (Fig. 2A). Though the culture period and concentration of GM-CSF can vary, this method is highly standardized. The other technique for generating DCs, especially from humans, is differentiating DCs from peripheral monocytes (33343536). DCs differentiated in this system are called monocyte-derived DCs (MoDCs). Except that the origin of the DC is a monocyte, the other processes and principles of culture are almost identical to those of BMDCs (Fig. 2B). Incidentally, trials for differentiating human DCs from bone marrow have also been performed (3738).
When BMDCs or MoDCs are stimulated with certain factors, the DCs are able to turn into tolDCs (Fig. 3). While a variety of factors with the ability to induce tolDCs have been reported (39), there is no standardized protocol with the optimal concentration and treatment time. In the next section, we will introduce certain factors that are universally known as inducers of tolDCs.
There are several cytokines known to induce tolDCs from BMDCs or MoDCs in vitro. Among them, the most well-known cytokines are IL-10, IFN-γ, and TGF-β. IL-10-treated BMDCs have a low capacity to secrete inflammatory cytokines and to prime T cells (40). Moreover, after IL-10 treatment, BMDCs are inhibited in their maturation (4142). The effects of IL-10 on BMDCs also correspond to DCs isolated from mouse spleen ex vivo (43). In addition to IL-10, IFN-γ has been demonstrated to induce tolerogenicity in DCs. IFN-γ-treated MoDCs cannot efficiently present alloantigens to T cells. In addition, when co-cultured with naïve CD4+ T cells, IFN-γ-treated MoDCs induce a high level of FoxP3 expression in CD4+ T cells and trigger the regulatory function of T cells (44). Notably, the destiny of DCs, whether they become suppressors or activators, depends on the concentration of IFN-γ (454647). High doses of IFN-γ inhibit maturation of MoDCs and induce their regulatory functions. For example, as the concentration of IFN-γ increases, the ratio of IL-10 to IL-12 produced by DCs increases but the cytotoxicity of CD8+ T cells that are co-cultured with the DCs decreases (4546). On the contrary, Kerkar and his colleagues reported that IFN-γ triggers differentiation of MoDCs and the expression of co-stimulatory molecules as well as the MHC I molecule (47). IFN-γ treatment, when added to monocytes in the early differentiation stage, often results in the induction of macrophages rather than DCs (48). However, after exposure to a low dosage of IFN-γ, MoDCs are differentiated to tolDCs, thereby hindering the T cell response (47). Taken together, the treatment time and the concentration of IFN-γ are crucial for generating tolDCs, which suggests that a more defined protocol is still needed. Another cytokine, TGF-β, when added at the final stages of BMDC differentiation, has been reported to down-regulate the expression of co-stimulatory molecules and inhibit the production of inflammatory cytokines in BMDCs (4049).
Dexamethasone (Dexa) is a steroid medication widely used for the treatment of various diseases. There are many reports showing that Dexa is a tolerogenic compound. Dexa-stimulated DCs tend to exhibit down-regulated expression of co-stimulatory and MHC molecules, and subsequently an impaired T cell immune response (405051525354). Furthermore, in vitro, Dexa treatment of DCs causes T cells to produce high amounts of IL-10 (54). While Dexa treatment methods for generating tolDCs differ among studies, Dexa is a tolerogenic reagent.
Vitamin D3 (VitD3) is another well-known tolerogenic reagent. The addition of 1,25(OH)2D3, an active form of VitD3, into DC culture results in the induction of tolerogenic surface molecules and the production of anti-inflammatory cytokines. In VitD3-treated DCs, the expression levels of CD80, CD86, and MHC II decrease and also the production of IL-6 and IL-12 are limited, compared to non-treated DCs (5556). Functionally, VitD3 contributes to the down-regulation of IFN-γ in DCs, thereby suppressing the T cell immune response (56). Because of their practical use and easy handling, Dexa and VitD3 have also been utilized in vivo (57) and are often used in combination (5859).
Interestingly, DCs co-cultured with mesenchymal stem cells (MSCs) have immune-regulatory characteristics. In this system, DCs are overlaid on MSCs and cultured together (60). Under the influence of MSCs, DCs decrease IL-12 production and down-regulate expression of co-stimulatory and MHC molecules, resulting in the induction of tolDCs and T cell unresponsiveness (61). For this reason, there are quite a few trials using MSCs to suppress immune responses in vivo (6263).
TolDCs are now being tested for their therapeutic use in various types of diseases where immune suppression is needed. Diversely generated tolDCs are used for the treatment of hyper-immune and autoimmune disorders such as rheumatoid arthritis (RA), multiple sclerosis (MS), and excessive tissue destruction and can be used to alleviate transplantation rejection.
RA is the most representative and common autoimmune disease and has typical symptoms: synovitis and progressive cartilage destruction. In a murine system, the collagen-induced arthritis (CIA) model is widely used for the study of RA. Until now, common therapies for RA have included administration of immunosuppressive drugs and biomolecules even though these methods have fatal side effects causing general suppression of the host immune system (536465). Thus, adoptive transfer of tolDCs into a pathologic area can be a more effective treatment for RA patients. Adoption of tolDCs ameliorates inflammatory arthritis by inducing the production of anti-inflammatory cytokines and the generation of Treg cells, subsequently impairing the T cell immune response (666768697071727374757677). In addition to RA, other clinical trials targeting autoimmune disorders have recently adopted tolDCs. The disorders include MS (78), primary Sjögren's syndrome (59), immune thrombocytopenic purpura (79), diabetes (80), uveoretinitis (81), and experimental autoimmune encephalomyelitis (57).
TolDCs also play an important role in maintaining grafted organs (82838485). As in the treatment of autoimmune disorders, the use of immunosuppressive drugs has been a dominant treatment method for transplantation recipients. However, as mentioned above, the use of these suppressors often causes a breakdown of the host immune system. Recently, the administration of regulatory cells has been investigated (86). Although there is still a long way to go before achieving the ideal application, therapy using tolDCs is potentially the most promising and powerful tool to escape graft destruction in transplantation patients compared with therapy using other regulatory cells. As immunoregulatory APCs, tolDCs may be able to regulate and control other immune cells, which together give rise to tolerance. Although there have been many trials in animal transplantation models (blood, skin, heart, kidney, and pancreatic islet transplantations), clinical trials in humans have not been widely reported (87). It is obvious that injection of tolDCs can effectively suppress host immune system by inhibition of Ag-specific T cells and induction of Ag-specific Treg cells, but there are still many questions to be solved for safe and effective clinical goal:
Which tolDCs should be used: donor or recipient? Which time-point is appropriate for injecting tolDCs: prior-, peri-, or post-transplantation? Which route is more effective for injecting tolDCs: intravenous, intradermal, subcutaneous, or another route? What are the ideal dosage and frequency of administration (87)?
We have discussed the generation, characteristics, and therapeutic applications of tolDCs. The definite phenotype and biomarkers for tolDCs have not been defined and opinions regarding tolDCs are diverse. Sometimes, even immature or semi-mature DCs are simply regarded as tolDCs. Furthermore, conditioning tolDCs is also very complicated because tolerance-inducing factors and manipulations for generating tolDCs are extremely varied.
TolDCs are a promising tool to suppress immune responses in autoimmune disorders and transplantations. However, additional research is needed for clinical trials. First, in the preparation stage of conditioned DCs, specific protocols to generate tolDCs suitable for each application must be established. Establishing the appropriate timing and intensity of growth factor and tolerogenic reagent treatment is crucial. Furthermore, the migration, and toxicity issue of tolDCs in the host after injection should also be considered. As another concern, the stability of administered tolDCs in vivo must be also considered for effective therapeutic applications. Various types of tolDCs generated with diverse factors mentioned above were known to keep stably their tolerogenic phenotype and function in vivo (538889), whereas there have been some reports showing that semi-mature DCs, which have been usually considered as tolDCs (909192), became immunogenic cells when they were administered to in vivo (9394). Even if there are a lot of things to be investigated, tolDCs could be powerful immune cells with immense potential for innovative clinical therapy in the future.
Figures and Tables
Figure 1
Typical characteristics of tolerogenic dendritic cells. Down-regulation of co-stimulatory molecules (CD40, CD80, and CD86), which contribute to T cell priming and further immune responses, can be regarded as the tolerogenic phenotype of DCs. In contrast, up-regulation of inhibitory molecules (PD-L1, PD-L2, and FasL) of DCs can be a sign of tolerance because functional anergy or cell death can be induced in immune cells that have interacted with those inhibitory molecules. In terms of the cytokine-secreting profile, DCs secreting anti-inflammatory cytokines can be considered tolerogenic DCs by regulatory T cells; these DCs inhibit synthesis of inflammatory cytokines. Additionally, high expression of the immunomodulatory enzyme IDO and low expression of NF-κB allow DCs to acquire tolerogenic potential.
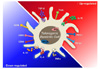
Figure 2
Generation of dendritic cells in vitro: at-a-glance. Substantial numbers of DCs can be obtained using in vitro culture systems with GM-CSF. DCs generated in culture with GM-CSF are imDCs, which can reach the mature stage by further stimulation with LPS or TNF-α. (A) In the case of mouse DCs, a large amount of DCs can be generated in the culture of murine BM cells treated with GM-CSF. BM cells are flushed out from the femur and tibia. After lysis of erythrocytes, the cells are cultured in medium containing GM-CSF for 6 to 10 days. In this murine BM culture system, addition and replacement of medium are conducted on the one-third and two-third days of whole culture, respectively. DCs generated by this method in vitro are called BMDCs. (B) For generating human DCs in vitro, monocytes are isolated from peripheral blood mononuclear cells (PBMCs) and are cultured in the presence of GM-CSF. DCs differentiated from monocytes using this system are called MoDCs.
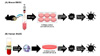
Figure 3
Factors for tolDC induction. Various factors contribute to the induction of tolDCs in vitro. These tolerogenic factors are categorized into four groups: cytokines, chemicals and drugs, organic molecules, and other factors. TSLP, thymic stromal lymphopoietin; IVIG, intravenous immunoglobulin; VIP, vasoactive intestinal peptide; HGF, hepatocyte growth factor.
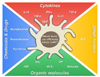
ACKNOWLEDGEMENTS
This research was supported by the Bio & Medical Technology Development Program of the National Research Foundation of Korea (NRF) grant funded by the Korean government, MSIP (2012M3A9B4028264 and 2015R1A2 A1A10056084).
References
1. Freeman GJ, Long AJ, Iwai Y, Bourque K, Chernova T, Nishimura H, Fitz LJ, Malenkovich N, Okazaki T, Byrne MC, Horton HF, Fouser L, Carter L, Ling V, Bowman MR, Carreno BM, Collins M, Wood CR, Honjo T. Engagement of the PD-1 immunoinhibitory receptor by a novel B7 family member leads to negative regulation of lymphocyte activation. J Exp Med. 2000; 192:1027–1034.


2. Latchman Y, Wood CR, Chernova T, Chaudhary D, Borde M, Chernova I, Iwai Y, Long AJ, Brown JA, Nunes R, Greenfield EA, Bourque K, Boussiotis VA, Carter LL, Carreno BM, Malenkovich N, Nishimura H, Okazaki T, Honjo T, Sharpe AH, Freeman GJ. PD-L2 is a second ligand for PD-1 and inhibits T cell activation. Nat Immunol. 2001; 2:261–268.


3. Martin-Orozco N, Wang YH, Yagita H, Dong C. Cutting Edge: Programmed death (PD) ligand-1/PD-1 interaction is required for CD8+ T cell tolerance to tissue antigens. J Immunol. 2006; 177:8291–8295.


4. Kuipers H, Muskens F, Willart M, Hijdra D, van Assema FB, Coyle AJ, Hoogsteden HC, Lambrecht BN. Contribution of the PD-1 ligands/PD-1 signaling pathway to dendritic cell-mediated CD4+ T cell activation. Eur J Immunol. 2006; 36:2472–2482.


5. Brown JA, Dorfman DM, Ma FR, Sullivan EL, Munoz O, Wood CR, Greenfield EA, Freeman GJ. Blockade of programmed death-1 ligands on dendritic cells enhances T cell activation and cytokine production. J Immunol. 2003; 170:1257–1266.


6. Chen C, Qu QX, Huang JA, Zhu YB, Ge Y, Wang Q, Zhang XG. Expression of programmed-death receptor ligands 1 and 2 may contribute to the poor stimulatory potential of murine immature dendritic cells. Immunobiology. 2007; 212:159–165.


7. Chen L, Zhang Z, Chen W, Zhang Z, Li Y, Shi M, Zhang J, Chen L, Wang S, Wang FS. B7-H1 up-regulation on myeloid dendritic cells significantly suppresses T cell immune function in patients with chronic hepatitis B. J Immunol. 2007; 178:6634–6641.


8. Curiel TJ, Wei S, Dong H, Alvarez X, Cheng P, Mottram P, Krzysiek R, Knutson KL, Daniel B, Zimmermann MC, David O, Burow M, Gordon A, Dhurandhar N, Myers L, Berggren R, Hemminki A, Alvarez RD, Emilie D, Curiel DT, Chen L, Zou W. Blockade of B7-H1 improves myeloid dendritic cell-mediated antitumor immunity. Nat Med. 2003; 9:562–567.


9. Kim HK, Guan H, Zu G, Li H, Wu L, Feng X, Elmets C, Fu Y, Xu H. High-level expression of B7-H1 molecules by dendritic cells suppresses the function of activated T cells and desensitizes allergen-primed animals. J Leukoc Biol. 2006; 79:686–695.


10. Latchman YE, Liang SC, Wu Y, Chernova T, Sobel RA, Klemm M, Kuchroo VK, Freeman GJ, Sharpe AH. PD-L1-deficient mice show that PD-L1 on T cells, antigen-presenting cells, and host tissues negatively regulates T cells. Proc Natl Acad Sci U S A. 2004; 101:10691–10696.


11. Selenko-Gebauer N, Majdic O, Szekeres A, Hofler G, Guthann E, Korthauer U, Zlabinger G, Steinberger P, Pickl WF, Stockinger H, Knapp W, Stockl J. B7-H1 (programmed death-1 ligand) on dendritic cells is involved in the induction and maintenance of T cell anergy. J Immunol. 2003; 170:3637–3644.


12. Tokita D, Mazariegos GV, Zahorchak AF, Chien N, Abe M, Raimondi G, Thomson AW. High PD-L1/CD86 ratio on plasmacytoid dendritic cells correlates with elevated T-regulatory cells in liver transplant tolerance. Transplantation. 2008; 85:369–377.


13. Waisman A, Yogev N. B7-H1 and CD8+ Treg: the enigmatic role of B7-H1 in peripheral tolerance. Eur J Immunol. 2009; 39:1448–1451.


15. Klas C, Debatin KM, Jonker RR, Krammer PH. Activation interferes with the APO-1 pathway in mature human T cells. Int Immunol. 1993; 5:625–630.


18. Spits H, de Waal MR. Functional characterization of human IL-10. Int Arch Allergy Immunol. 1992; 99:8–15.


19. Taga K, Tosato G. IL-10 inhibits human T cell proliferation and IL-2 production. J Immunol. 1992; 148:1143–1148.
20. Rennick D, Berg D, Holland G. Interleukin 10: an overview. Prog Growth Factor Res. 1992; 4:207–227.


21. de Waal Malefyt R, Haanen J, Spits H, Roncarolo MG, te VA, Figdor C, Johnson K, Kastelein R, Yssel H, de Vries JE. Interleukin 10 (IL-10) and viral IL-10 strongly reduce antigen-specific human T cell proliferation by diminishing the antigen-presenting capacity of monocytes via downregulation of class II major histocompatibility complex expression. J Exp Med. 1991; 174:915–924.


22. Sanjabi S, Zenewicz LA, Kamanaka M, Flavell RA. Anti-inflammatory and pro-inflammatory roles of TGF-beta, IL-10, and IL-22 in immunity and autoimmunity. Curr Opin Pharmacol. 2009; 9:447–453.


23. Grohmann U, Belladonna ML, Bianchi R, Orabona C, Ayroldi E, Fioretti MC, Puccetti P. IL-12 acts directly on DC to promote nuclear localization of NF-kappaB and primes DC for IL-12 production. Immunity. 1998; 9:315–323.


24. Xie FT, Cao JS, Zhao J, Yu Y, Qi F, Dai XC. IDO expressing dendritic cells suppress allograft rejection of small bowel transplantation in mice by expansion of Foxp3+ regulatory T cells. Transpl Immunol. 2015; 33:69–77.


25. Hwu P, Du MX, Lapointe R, Do M, Taylor MW, Young HA. Indoleamine 2,3-dioxygenase production by human dendritic cells results in the inhibition of T cell proliferation. J Immunol. 2000; 164:3596–3599.


26. Frumento G, Rotondo R, Tonetti M, Damonte G, Benatti U, Ferrara GB. Tryptophan-derived catabolites are responsible for inhibition of T and natural killer cell proliferation induced by indoleamine 2,3-dioxygenase. J Exp Med. 2002; 196:459–468.


27. Munn DH, Sharma MD, Lee JR, Jhaver KG, Johnson TS, Keskin DB, Marshall B, Chandler P, Antonia SJ, Burgess R, Slingluff CL Jr, Mellor AL. Potential regulatory function of human dendritic cells expressing indoleamine 2,3-dioxygenase. Science. 2002; 297:1867–1870.


28. Terness P, Bauer TM, Rose L, Dufter C, Watzlik A, Simon H, Opelz G. Inhibition of allogeneic T cell proliferation by indoleamine 2,3-dioxygenase-expressing dendritic cells: mediation of suppression by tryptophan metabolites. J Exp Med. 2002; 196:447–457.


29. van der Marel AP, Samsom JN, Greuter M, van Berkel LA, O'Toole T, Kraal G, Mebius RE. Blockade of IDO inhibits nasal tolerance induction. J Immunol. 2007; 179:894–900.


30. Inaba K, Inaba M, Romani N, Aya H, Deguchi M, Ikehara S, Muramatsu S, Steinman RM. Generation of large numbers of dendritic cells from mouse bone marrow cultures supplemented with granulocyte/macrophage colony-stimulating factor. J Exp Med. 1992; 176:1693–1702.


31. Inaba K, Inaba M, Deguchi M, Hagi K, Yasumizu R, Ikehara S, Muramatsu S, Steinman RM. Granulocytes, macrophages, and dendritic cells arise from a common major histocompatibility complex class II-negative progenitor in mouse bone marrow. Proc Natl Acad Sci U S A. 1993; 90:3038–3042.


32. Witmer-Pack MD, Olivier W, Valinsky J, Schuler G, Steinman RM. Granulocyte/macrophage colony-stimulating factor is essential for the viability and function of cultured murine epidermal Langerhans cells. J Exp Med. 1987; 166:1484–1498.


33. Caux C, zutter-Dambuyant C, Schmitt D, Banchereau J. GM-CSF and TNF-alpha cooperate in the generation of dendritic Langerhans cells. Nature. 1992; 360:258–261.


34. Santiago-Schwarz F, Belilos E, Diamond B, Carsons SE. TNF in combination with GM-CSF enhances the differentiation of neonatal cord blood stem cells into dendritic cells and macrophages. J Leukoc Biol. 1992; 52:274–281.


35. Caux C, Vanbervliet B, Massacrier C, zutter-Dambuyant C, de Saint-Vis B, Jacquet C, Yoneda K, Imamura S, Schmitt D, Banchereau J. CD34+ hematopoietic progenitors from human cord blood differentiate along two independent dendritic cell pathways in response to GM-CSF+TNF alpha. J Exp Med. 1996; 184:695–706.


36. Rosenzwajg M, Canque B, Gluckman JC. Human dendritic cell differentiation pathway from CD34+ hematopoietic precursor cells. Blood. 1996; 87:535–544.


37. Reid CD, Stackpoole A, Meager A, Tikerpae J. Interactions of tumor necrosis factor with granulocyte-macrophage colony-stimulating factor and other cytokines in the regulation of dendritic cell growth in vitro from early bipotent CD34+ progenitors in human bone marrow. J Immunol. 1992; 149:2681–2688.
38. Szabolcs P, Moore MA, Young JW. Expansion of immunostimulatory dendritic cells among the myeloid progeny of human CD34+ bone marrow precursors cultured with c-kit ligand, granulocyte-macrophage colony-stimulating factor, and TNF-alpha. J Immunol. 1995; 154:5851–5861.
39. Torres-Aguilar H, Blank M, Jara LJ, Shoenfeld Y. Tolerogenic dendritic cells in autoimmune diseases: crucial players in induction and prevention of autoimmunity. Autoimmun Rev. 2010; 10:8–17.
40. Boks MA, Kager-Groenland JR, Haasjes MS, Zwaginga JJ, van Ham SM, ten BA. IL-10-generated tolerogenic dendritic cells are optimal for functional regulatory T cell induction--a comparative study of human clinical-applicable DC. Clin Immunol. 2012; 142:332–342.


41. Haase C, Jorgensen TN, Michelsen BK. Both exogenous and endogenous interleukin-10 affects the maturation of bone-marrow-derived dendritic cells in vitro and strongly influences T-cell priming in vivo. Immunology. 2002; 107:489–499.


42. Muller G, Muller A, Tuting T, Steinbrink K, Saloga J, Szalma C, Knop J, Enk AH. Interleukin-10-treated dendritic cells modulate immune responses of naive and sensitized T cells in vivo. J Invest Dermatol. 2002; 119:836–841.
43. De Smedt T, Van Mechelen M, De Becker G, Urbain J, Leo O, Moser M. Effect of interleukin-10 on dendritic cell maturation and function. Eur J Immunol. 1997; 27:1229–1235.


44. Eljaafari A, Li YP, Miossec P. IFN-gamma, as secreted during an alloresponse, induces differentiation of monocytes into tolerogenic dendritic cells, resulting in FoxP3+ regulatory T cell promotion. J Immunol. 2009; 183:2932–2945.


45. Svajger U, Obermajer N, Jeras M. IFN-gamma-rich environment programs dendritic cells toward silencing of cytotoxic immune responses. J Leukoc Biol. 2014; 95:33–46.


46. Della Bella S, Mavilio D. IFN-gamma: a Janus-faced cytokine in dendritic cell programming. J Leukoc Biol. 2014; 95:6–8.
47. Kerkar SP, Chinnasamy D, Hadi N, Melenhorst J, Muranski P, Spyridonidis A, Ito S, Weber G, Yin F, Hensel N, Wang E, Marincola FM, Barrett AJ. Timing and intensity of exposure to interferon-gamma critically determines the function of monocyte-derived dendritic cells. Immunology. 2014; 143:96–108.


48. Delneste Y, Charbonnier P, Herbault N, Magistrelli G, Caron G, Bonnefoy JY, Jeannin P. Interferon-gamma switches monocyte differentiation from dendritic cells to macrophages. Blood. 2003; 101:143–150.


49. Thomas DC, Wong FS, Zaccone P, Green EA, Wallberg M. Protection of islet grafts through transforming growth factor-beta-induced tolerogenic dendritic cells. Diabetes. 2013; 62:3132–3142.


50. O'Flynn L, Treacy O, Ryan AE, Morcos M, Cregg M, Gerlach J, Joshi L, Nosov M, Ritter T. Donor bone marrow-derived dendritic cells prolong corneal allograft survival and promote an intragraft immunoregulatory milieu. Mol Ther. 2013; 21:2102–2112.
51. Anderson AE, Sayers BL, Haniffa MA, Swan DJ, Diboll J, Wang XN, Isaacs JD, Hilkens CM. Differential regulation of naive and memory CD4+ T cells by alternatively activated dendritic cells. J Leukoc Biol. 2008; 84:124–133.


52. Anderson AE, Swan DJ, Sayers BL, Harry RA, Patterson AM, von Delwig A, Robinson JH, Isaacs JD, Hilkens CM. LPS activation is required for migratory activity and antigen presentation by tolerogenic dendritic cells. J Leukoc Biol. 2009; 85:243–250.


53. Harry RA, Anderson AE, Isaacs JD, Hilkens CM. Generation and characterisation of therapeutic tolerogenic dendritic cells for rheumatoid arthritis. Ann Rheum Dis. 2010; 69:2042–2050.


54. Bosma BM, Metselaar HJ, Nagtzaam NM, de Haan R, Mancham S, van der Laan LJ, Kuipers EJ, Kwekkeboom J. Dexamethasone transforms lipopolysaccharide-stimulated human blood myeloid dendritic cells into myeloid dendritic cells that prime interleukin-10 production in T cells. Immunology. 2008; 125:91–100.


55. Ferreira GB, van Etten E, Verstuyf A, Waer M, Overbergh L, Gysemans C, Mathieu C. 1,25-Dihydroxyvitamin D3 alters murine dendritic cell behaviour in vitro and in vivo. Diabetes Metab Res Rev. 2011; 27:933–941.


56. Matsuzaki J, Tsuji T, Zhang Y, Wakita D, Imazeki I, Sakai T, Ikeda H, Nishimura T. 1alpha,25-Dihydroxyvitamin D3 downmodulates the functional differentiation of Th1 cytokine-conditioned bone marrow-derived dendritic cells beneficial for cytotoxic T lymphocyte generation. Cancer Sci. 2006; 97:139–147.


57. Farias AS, Spagnol GS, Bordeaux-Rego P, Oliveira CO, Fontana AG, de Paula RF, Santos MP, Pradella F, Moraes AS, Oliveira EC, Longhini AL, Rezende AC, Vaisberg MW, Santos LM. Vitamin D3 induces IDO+ tolerogenic DCs and enhances Treg, reducing the severity of EAE. CNS Neurosci Ther. 2013; 19:269–277.


58. Nikolic T, Roep BO. Regulatory multitasking of tolerogenic dendritic cells - lessons taken from vitamin d3-treated tolerogenic dendritic cells. Front Immunol. 2013; 4:113.


59. Volchenkov R, Brun JG, Jonsson R, Appel S. In vitro suppression of immune responses using monocyte-derived tolerogenic dendritic cells from patients with primary Sjogren's syndrome. Arthritis Res Ther. 2013; 15:R114.
60. Jiang XX, Zhang Y, Liu B, Zhang SX, Wu Y, Yu XD, Mao N. Human mesenchymal stem cells inhibit differentiation and function of monocyte-derived dendritic cells. Blood. 2005; 105:4120–4126.


61. Beyth S, Borovsky Z, Mevorach D, Liebergall M, Gazit Z, Aslan H, Galun E, Rachmilewitz J. Human mesenchymal stem cells alter antigen-presenting cell maturation and induce T-cell unresponsiveness. Blood. 2005; 105:2214–2219.


62. Ge W, Jiang J, Baroja ML, Arp J, Zassoko R, Liu W, Bartholomew A, Garcia B, Wang H. Infusion of mesenchymal stem cells and rapamycin synergize to attenuate alloimmune responses and promote cardiac allograft tolerance. Am J Transplant. 2009; 9:1760–1772.


63. Wang H, Qi F, Dai X, Tian W, Liu T, Han H, Zhang B, Li H, Zhang Z, Du C. Requirement of B7-H1 in mesenchymal stem cells for immune tolerance to cardiac allografts in combination therapy with rapamycin. Transpl Immunol. 2014; 31:65–74.


64. Guyot P, Taylor P, Christensen R, Pericleous L, Poncet C, Lebmeier M, Drost P, Bergman G. Abatacept with methotrexate versus other biologic agents in treatment of patients with active rheumatoid arthritis despite methotrexate: a network meta-analysis. Arthritis Res Ther. 2011; 13:R204.


65. Hilkens CM, Isaacs JD. Tolerogenic dendritic cell therapy for rheumatoid arthritis: where are we now? Clin Exp Immunol. 2013; 172:148–157.


66. Bianco NR, Kim SH, Ruffner MA, Robbins PD. Therapeutic effect of exosomes from indoleamine 2,3-dioxygenase-positive dendritic cells in collagen-induced arthritis and delayed-type hypersensitivity disease models. Arthritis Rheum. 2009; 60:380–389.


67. Chorny A, Gonzalez-Rey E, Fernandez-Martin A, Pozo D, Ganea D, Delgado M. Vasoactive intestinal peptide induces regulatory dendritic cells with therapeutic effects on autoimmune disorders. Proc Natl Acad Sci U S A. 2005; 102:13562–13567.


68. Healy LJ, Collins HL, Thompson SJ. Systemic administration of tolerogenic dendritic cells ameliorates murine inflammatory arthritis. Open Rheumatol J. 2008; 2:71–80.


69. Jaen O, Rulle S, Bessis N, Zago A, Boissier MC, Falgarone G. Dendritic cells modulated by innate immunity improve collagen-induced arthritis and induce regulatory T cells in vivo. Immunology. 2009; 126:35–44.


70. Kim SH, Kim S, Evans CH, Ghivizzani SC, Oligino T, Robbins PD. Effective treatment of established murine collagen-induced arthritis by systemic administration of dendritic cells genetically modified to express IL-4. J Immunol. 2001; 166:3499–3505.


71. Morita Y, Yang J, Gupta R, Shimizu K, Shelden EA, Endres J, Mule JJ, McDonagh KT, Fox DA. Dendritic cells genetically engineered to express IL-4 inhibit murine collagen-induced arthritis. J Clin Invest. 2001; 107:1275–1284.


72. Ning B, Wei J, Zhang A, Gong W, Fu J, Jia T, Yang SY. Antigen-specific tolerogenic dendritic cells ameliorate the severity of murine collagen-induced arthritis. PLoS One. 2015; 10:e0131152.


73. Popov I, Li M, Zheng X, San H, Zhang X, Ichim TE, Suzuki M, Feng B, Vladau C, Zhong R, Garcia B, Strejan G, Inman RD, Min WP. Preventing autoimmune arthritis using antigen-specific immature dendritic cells: a novel tolerogenic vaccine. Arthritis Res Ther. 2006; 8:R141.
74. Salazar L, Aravena O, Abello P, Escobar A, Contreras-Levicoy J, Rojas-Colonelli N, Catalan D, Aguirre A, Zuniga R, Pesce B, Gonzalez C, Cepeda R, Cuchacovich M, Molina MC, Salazar-Onfray F, Delgado M, Toes RE, Aguillon JC. Modulation of established murine collagen-induced arthritis by a single inoculation of short-term lipopolysaccharide-stimulated dendritic cells. Ann Rheum Dis. 2008; 67:1235–1241.


75. Steinbrink K, Wolfl M, Jonuleit H, Knop J, Enk AH. Induction of tolerance by IL-10-treated dendritic cells. J Immunol. 1997; 159:4772–4780.
76. van Duivenvoorde LM, Han WG, Bakker AM, Louis-Plence P, Charbonnier LM, Apparailly F, van der Voort EI, Jorgensen V, Huizinga TW, Toes RE. Immunomodulatory dendritic cells inhibit Th1 responses and arthritis via different mechanisms. J Immunol. 2007; 179:1506–1515.


77. van Duivenvoorde LM, Louis-Plence P, Apparailly F, van der Voort EI, Huizinga TW, Jorgensen C, Toes RE. Antigen-specific immunomodulation of collagen-induced arthritis with tumor necrosis factor-stimulated dendritic cells. Arthritis Rheum. 2004; 50:3354–3364.


78. Xiao BG, Zhu WH, Lu CZ. The presence of GM-CSF and IL-4 interferes with effect of TGF-beta1 on antigen presenting cells in patients with multiple sclerosis and in rats with experimental autoimmune encephalomyelitis. Cell Immunol. 2007; 249:30–36.


79. Fu J, Zhang A, Ju X. Tolerogenic dendritic cells as a target for the therapy of immune thrombocytopenia. Clin Appl Thromb Hemost. 2012; 18:469–475.


80. Adorini L. Tolerogenic dendritic cells induced by vitamin D receptor ligands enhance regulatory T cells inhibiting autoimmune diabetes. Ann N Y Acad Sci. 2003; 987:258–261.


81. Lau AW, Biester S, Cornall RJ, Forrester JV. Lipopolysaccharide-activated IL-10-secreting dendritic cells suppress experimental autoimmune uveoretinitis by MHCII-dependent activation of CD62L-expressing regulatory T cells. J Immunol. 2008; 180:3889–3899.


82. Silva Pde M, Bier J, Paiatto LN, Galdino AC, Lopes SC, Fernandes LG, Tamashiro WM, Simioni PU. Tolerogenic Dendritic Cells on Transplantation: Immunotherapy Based on Second Signal Blockage. J Immunol Res. 2015; 2015:856707.
83. Ezzelarab M, Thomson AW. Tolerogenic dendritic cells and their role in transplantation. Semin Immunol. 2011; 23:252–263.


84. Li GP, Yang J, Hao J, Yang YM, Ren YN, Xie RF, Fan HH, Qian KC. [The role of third-party tolerogenic dendritic cells in the prevention of acute graft-versus-host-disease following allogeneic bone marrow transplantation in mice]. Zhonghua Xue Ye Xue Za Zhi. 2012; 33:461–466.
85. Moreau A, Varey E, Bouchet-Delbos L, Cuturi MC. Cell therapy using tolerogenic dendritic cells in transplantation. Transplant Res. 2012; 1:13.


86. Cobbold SP, Waldmann H. Regulatory cells and transplantation tolerance. Cold Spring Harb Perspect Med. 2013; 3:pii: a015545.


87. Moreau A, Varey E, Beriou G, Hill M, Bouchet-Delbos L, Segovia M, Cuturi MC. Tolerogenic dendritic cells and negative vaccination in transplantation: from rodents to clinical trials. Front Immunol. 2012; 3:218.


88. Machen J, Harnaha J, Lakomy R, Styche A, Trucco M, Giannoukakis N. Antisense oligonucleotides down-regulating costimulation confer diabetes-preventive properties to nonobese diabetic mouse dendritic cells. J Immunol. 2004; 173:4331–4341.


89. Stoop JN, Harry RA, von Delwig A, Isaacs JD, Robinson JH, Hilkens CM. Therapeutic effect of tolerogenic dendritic cells in established collagen-induced arthritis is associated with a reduction in Th17 responses. Arthritis Rheum. 2010; 62:3656–3665.


90. Morel PA, Turner MS. Dendritic cells and the maintenance of self-tolerance. Immunol Res. 2011; 50:124–129.


91. Lutz MB, Schuler G. Immature, semi-mature and fully mature dendritic cells: which signals induce tolerance or immunity? Trends Immunol. 2002; 23:445–449.


92. Dudek AM, Martin S, Garg AD, Agostinis P. Immature, Semi-Mature, and Fully Mature Dendritic Cells: Toward a DC-Cancer Cells Interface That Augments Anticancer Immunity. Front Immunol. 2013; 4:438.


93. Voigtlander C, Rossner S, Cierpka E, Theiner G, Wiethe C, Menges M, Schuler G, Lutz MB. Dendritic cells matured with TNF can be further activated in vitro and after subcutaneous injection in vivo which converts their tolerogenicity into immunogenicity. J Immunother. 2006; 29:407–415.