Abstract
In this study, we compared two different tumor cell vaccines for their induction of anti-tumor immunity; one was a tumor cell clone expressing a membrane-bound form of IL-12 p35 subunit (mbIL-12 p35 tumor clone), and the other was a tumor clone expressing heterodimeric IL-12 as a single chain (mb-scIL-12 tumor clone). The stimulatory effect of mb-scIL-12 on the proliferation of ConA-activated splenocytes was higher than that of mbIL-12 p35 in vitro. However, the stimulatory effect of mbIL-12 p35 was equivalent to that of recombinant soluble IL-12 (3 ng/ml). Interestingly, both tumor clones (mbIL-12 p35 and mb-scIL-12) showed similar tumorigenicity and induction of systemic anti-tumor immunity in vivo, suggesting that tumor cell expression of the membrane-bound p35 subunit is sufficient to induce anti-tumor immunity in our tumor vaccine model.
IL-12 is a heterodimeric cytokine consisting of p35 and p40 subunits (1) that is produced by phagocytic cells, B cells, and other antigen presenting cells (2). IL-12 has been reported to drive differentiation of naïve T cells into interferon gamma-producing Th1 cells (3) and enhance NK and CTL activity (4). The astonishing anti-tumor effect of recombinant IL-12 has been reported in murine tumor models and human clinical studies (567). Because of its cytotoxicity in vivo or in cancer patients (8910), cytokine gene therapy and DNA vaccines have been adopted to achieve local delivery of cytokines (11121314).
In our previous reports, we attempted a new strategy to manipulate the activity of cytokines by expressing them on tumor cell membranes (151617). We hypothesized that membrane-bound cytokines could selectively activate tumor antigen-specific effector cells. Cytokines on tumor cells may function as co-stimulatory molecules for tumor antigen-specific CTL activation via a juxtacrine effect.
In previous studies, we expressed each of the p35 and p40 subunit molecules of IL-12 as a membrane-bound form on MethA fibrosarcoma cells. Surprisingly, the tumor cell clone expressing only the membrane-bound form of p35 induced anti-tumor immunity (1718). Since endogenous IL-12 p40 is produced excessively in vivo (1920), we envisaged that mbIL-12 p35 on tumor cells might bind the p40 subunit to form a heterodimer.
In this study, we compared mbIL-12 p35- and mb-scIL-12-expressing tumor cells for tumorigenicity and the ability to induce anti-tumor immunity. The stimulatory effect of mb-scIL-12-expressing tumor clones on the proliferation of ConA-activated splenocytes was higher than that of mbIL-12p35 in vitro, whereas tumorigenicity and the ability to induce systemic anti-tumor immunity in mice were similar between the two clones. These results suggest that expression of membrane-bound IL-12 p35 subunit on tumor cells could be a strategy for developing tumor cell vaccines.
The MethA tumor cell line, derived from a BALB/c mouse by treatment with 3-methylcholanthrene, was maintained in RPMI-1640 medium (Welgene, Gyeongsan, Korea) including 10% heat-inactivated fatal bovine serum (FBS) (Atlas Biologicals, Fort Collins, CO) and 1% penicillin-streptomycin (Gibco, Dublin, Ireland). Approximately 6-week-old of female BALB/c mice were obtained from Daehan Biolink (Eumseong, Korea) and all animal procedures were approved and guided by the committee for experimental animal care used at Chungnam National University.
Anti-IL-12 p35 monoclonal mouse IgG1 antibody, (R&D systems, Minneapolis, MN, USA) and FITC-conjugated anti-mouse IgG antibody (Sigma-Aldrich, St. Louis, MO, USA) were used to detect expression of IL-12 fusion proteins on the cell surface of MethA tumor clones. Red blood cell lysis buffer, Concanavalin A (ConA) was purchased from Sigma-Aldrich, Mitomycin-C from Enzo life sciences (Farmingdale, NY, USA), and G418 (gentamycin) from Calbiochem (San Diego, CA, USA).
RNA was extracted from splenocytes treated with LPS for 24 h for use as template to amplify the cDNA encoding membrane-bound single chain IL-12. Mouse TNF-α cDNA was obtained from Dr. Sang Young Chun (Chonnam National University, Korea). Primers, containing a linker sequence, and specific for IL-12 p40 (forward: 5'-GCG GAT CCA TGA GCA CAG AAA GCA TG-3', reverse: 5'-AGA TCC GCC GCC ACC CGA CCC ACC ACC GCC CGA GCC ACC GCC ACC GGA TCG GAC CCT GCA GGG AAC-3') and IL-12 p35 (forward: 5'-GGT GGC GGT GGC TCG GGC GGT GGT GGG TCG GGT GGC GGC GGA TCT AGG GTC ATT CCA GTC TCT GGA CC-3', reverse: 5'-GCG CTC GAG TCA GGC GGA GCT CAG ATA GCC-3') were used to amplify respective cDNA fragments. IL-12 p40 (1062 bp) and IL-12 p35 (636 bp) cDNA fragments were linked by overlap extension PCR using the forward primer of IL-12 p40 and the reverse primer of IL-12 p35 to construct the cDNA encoding mb-scIL-12. The mb-scIL-12 (1653 bp) cDNA fragment was digested with EcoRI/XhoI and subcloned into pcDNA3.1 containing a 225 bp TNF-α cDNA fragment encoding the cytoplasmic region (from –75 to –45), transmembrane region (from –44 to –24), and extracellular region (from –23 to –5) (17). The constructed plasmid was confirmed by DNA sequence analysis (Genotech, Daejeon, Korea). Plasmids encoding mbIL-12 p35 and sc-mbIL-12 were transfected into MethA tumor cells using BioT Transfection reagent (Morganville Scientific, Morganville, NJ, USA). After 48 h, cells were plated in flat-bottom 96-well plates with medium containing 1 mg/ml of G418. Drug-resistant colonies formed 2-3 weeks after transfection.
Drug-resistant clones were analyzed for expression of IL-12 mRNA by RT-PCR. Total RNA was extracted from MethA clones using an Easy-blue RNA extraction kit (Intron, Sungnam, Korea). RT-PCR was conducted at 42℃ for 1 h using 1 µg of total RNA, oligo (dT) primers (18-mer) and M-MLV reverse transcriptase (Bioneer, Daejeon, Korea). cDNA was amplified using IL-12 specific primers and HS-Tag premix (Geneall, Seoul, Korea) using a DNA thermal Cycler (Biorad, Hercules, CA, USA).
Surface expression of mbIL-12p35 and mb-scIL-12 on transfected tumor cells was analyzed by flow cytometry using specific antibodies to the IL-12 p35 (R&D system, Minneapolis, MN). Tumor cell clones were incubated with antibody diluted in staining buffer (1X PBS containing 1% BSA) for 30 min on ice, and washed once. Then the cells were incubated with FITC-conjugated goat anti-mouse IgG antibody (Sigma-Aldrich) for 30 min on ice. The cells were then washed once with the staining buffer and analyzed on a FACSCalibur flow cytometry using CELLQuest (BD Biosciences, San Jose, CA, USA).
To compare the biological activity of mbIL-12 p35 and mb-scIL-12 on MethA tumor clones, ConA-activated splenocytes were induced by the MethA clones, and proliferation was measured. Splenocytes isolated from a BALB/c mouse were activated by 2 µg/ml of ConA for 72 h. MethA tumor clones were inactivated with 50 µg/ml mitomycin C (MMC) for 30 min, and washed three times with complete medium. Splenocytes (5×104) and tumor cells (1×104) were co-cultured for 48 h, and cells were counted by trypan blue staining.
Syngeneic BALB/c mice were injected subcutaneously on the right flank with 1×106 live tumor cells, to assess the tumorigenicity of MethA tumor clones. An age-matched group of mice were used as a control. Tumor growth and survival were monitored in all mice. Three months later, surviving mice were re-injected intraperitoneally with 1×106 wild type MethA cells in 100 µl of PBS.
In previous studies, we produced stable mbIL-12 p35- or mbIL-12 p40-expressing tumor cells, but failed to produce a tumor cell line expressing both mbIL-12 p35 and mbIL-12 p40. In this approach, we intended to express both p35 and p40 as a type II transmembrane protein. Considering the 3D structure of IL-12, we determined that our construct might not result in the proper orientation between p35 and p40 subunits and the formation of a heterodimeric mbIL-12; therefore, we attempted to produce heterodimeric single chain IL-12 as a membrane-bound form, in this study.
MethA fibrosarcoma cells were transfected with expression vectors encoding mbIL-12 p35 and mb-scIL-12 chimeric cDNA (Fig. 1A). As a control, mock-vector transfection was also performed. Transfected cells were selected with G418, and resistant clones were analyzed for the expression of IL-12 mRNA by RT-PCR, and surface expression by FACS using an IL-12 p35-specific antibody. mbIL-12 expression was obvious by RT-PCR (Fig. 1B), whereas surface expression of mbIL-12 was marginally detectable by FACS analysis (Fig. 2A).
The expression of mbIL-12 p35 and mb-scIL-12 was obvious by RT-PCR analysis, whereas detection of the chimeric IL-12 molecules by FACS analysis showed only marginal expression compared to that of a previous report (21). In that study, a membrane-bound form of single chain IL-12 with the B7 transmembrane (TM) portion was expressed as a type I transmembrane protein (p40-p35-B7). In our study, we constructed the mb-scIL-12 expression vector as a type II transmembrane protein with TNFα (TNFα-p40-p35). The Lode group reported the successful expression of a secreted form of single chain IL-12 (p40-p35) with the same linker sequences used in our study (22). It is possible that the epitope on mbIL-12 p35 is not accessible to the anti-IL-12 p35 antibody used in our FACS analysis.
To examine the biological activity of IL-12 chimeric proteins on the surface of MethA tumor clones, MMC-inactivated tumor cells were co-cultured with ConA-activated splenocytes, and proliferation was measured (Fig. 2B). The mb-scIL-12-expressing clone was approximately two-fold more effective in inducing proliferation of ConA-activated splenocytes compared to the mb-IL-12 p35-expressing clone. Exogenous recombinant IL-12 (3 ng/ml) exerted a similar effect to the mb-IL-12p35 tumor clone. This result indicates that the heterodimeric single chain IL-12 on tumor cells maintain biological function, more so than mbIL-12 p35, even though its expression was difficult to detect by FACS analysis (Fig. 2A).
To compare the tumorigenicity of the stable transfectants, the tumor clones expressing IL-12 chimeric proteins were subcutaneously injected into BALB/c syngeneic mice, and tumor growth was monitored (Fig. 3A). All mice injected with wild type cells displayed rapid tumor growth and died within 2 months of injection (Fig. 3A and B). Tumorigenicity of mbIL-12 p35 and mb-scIL-12 tumor cells was similar, but was lower than that of wild type MethA cells. Four of five mice injected with mbIL-12 p35- and mb-scIL-12-expressing tumor clones were tumor-free for 3 months and completely survived for the duration of the experiment (Fig. 3B). Furthermore, in one of five mice injected with mbIL-12 p35- or mbscIL-12-expressing tumor clones, tumor formation was delayed compared to mice injected with wild type MethA cells (Fig. 3A) (tumor diameters at 30 days: wild type tumor cells, average 19.4 mm; mbIL-12p35 clone, 8.4 mm; mb-scIL-12 clone, 10.1 mm). However, after tumor formation, the growth rate was similar regardless of the presence of ectopic IL-12 expression.
To verify anti-tumor immunity in IL-12 tumor clone-injected mice, the surviving mice (four mice from each group) were injected with wild type MethA cells intraperitoneally (IP), 3 months after the first tumor cell injection. As a control, untreated age-matched mice were also injected, and survival was monitored. All mice in the control group formed tumors and died within 1 month, whereas all mice in the mbIL-12 p35 and mb-scIL-12 p35 groups remained tumor-free for 40 days after IP injection (Fig. 4). These results clearly demonstrate that although the mb-scIL-12-expressing clone was more effective in inducing the proliferation of ConA-stimulated splenocytes, compared to the mbIL-12 p35-expressing clone (Fig. 2B), there was no obvious difference in tumorigenicity and induction of anti-tumor immunity between the two clones. Collectively, mbIL-12 p35 expression is as effective as heterodimeric single chain mb-scIL-12 expression for both inhibiting tumor growth and inducing immunological memory against cancer cells.
Among the IL-12 family, IL-12 shares its p40 subunit with IL-23, and p35 subunit with IL-35 (23). Both IL-12 and IL-23 exert anti-tumor activity, whereas IL-35, preferentially secreted by Tregs, decreases anti-tumor immunity; in addition, IL-35 expression in the tumor microenvironment enhances tumor growth (2324). Hypothetically, mbIL-12 p35 on tumor clones might have contrasting functions, specifically, immunostimulatory similar to IL-12 and immunosuppressive similar to IL-35. Furthermore, IL-23 should compete with IL-12 for the p40 subunit. Thus, the complex biological network of IL-12 family cytokines should be studied thoroughly in the context of anti-tumor immune responses to design new strategies for cytokine gene therapy.
Figures and Tables
Figure 1
Structure of bioactive mbIL-12 p35 and mb-scIL-12 chimeric DNA, and expression in MethA tumor cells. (A) Structure of mbIL-12 p35 and mb-scIL-12 chimeric DNA. mbIL-12 p35 has a 225 bp TNF-α cDNA fragment encoding the cytoplasmic region (from –75 to –45), the transmembrane region (from –44 to –24), and the extracellular region (from –23 to –5) at the N-terminus, a three amino acid sequence spacer (GGI), and the entire sequence of IL-12 p35. In mb-scIL-12, the p35 region was replaced by the entire sequence of IL-12 p40 linked to the IL-12 p35 mature sequence by a flexible 15 amino acid spacer sequence (GGGGS3). (B) mRNA expression of mbIL-12 p35 and mbscIL-12 in each of the transfected MethA tumor cells.
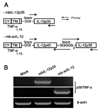
Figure 2
Expression of chimeric IL-12 on the MethA cell surface and proliferation of ConA-activated murine splenocytes after co-culture with MethA clones expressing mbIL-12 p35 and mb-scIL-12. (A) Surface expression of chimeric IL-12 proteins on MethA tumor cells. Cells were stained with an IL-12 p35 specific antibody and analyzed by flow cytometry. A fluorescent-conjugated secondary antibody, without the primary antibody, was used as a control. (B) Splenocytes were treated with 2 µg/ml of ConA for 72 h to activate T cells and MethA cells stably transfected with a mock vector, mbIL-12 p35, or mb-scIL-12, and were treated with 50 µg/ml of MMC for 30 min. After treatment, 5×104 splenocytes and 1×104 cells from each tumor clone were co-cultured for 48 h. ConA-activated splenocytes were treated with 3 ng/ml rIL-12 as a positive control. Cells were then counted by trypan blue staining (*p <0.05; **p <0.01; ***p <0.001).
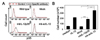
Figure 3
Tumor growth and survival of mice injected MethA tumor clones expressing membrane bound IL-12. BALB/c mice (n=5) were injected with 1×106 stable MethA transfectants (wild type, mbIL-12p35, or mb-scIL-12) subcutaneously on the right flank. (A) Tumor growth and (B) survival were monitored for 3 months. The tumor diameter was determined using calipers, and data represent the means±SD.
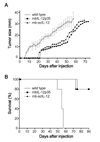
ACKNOWLEDGEMENTS
This research was supported by Basic Science Research Program through the National Research Foundation of Korea (NRF) funded by the Ministry of Education (2013R1A1A4A01013202) and a grant from Chungnam National University (2014).
References
1. Schoenhaut DS, Chua AO, Wolitzky AG, Quinn PM, Dwyer CM, McComas W, Familletti PC, Gately MK, Gubler U. Cloning and expression of murine IL-12. J Immunol. 1992; 148:3433–3440.
2. Trinchieri G. Interleukin-12: a cytokine produced by antigen-presenting cells with immunoregulatory functions in the generation of T-helper cells type 1 and cytotoxic lymphocytes. Blood. 1994; 84:4008–4027.


3. Hsieh CS, Macatonia SE, Tripp CS, Wolf SF, O'Garra A, Murphy KM. Development of TH1 CD4+ T cells through IL-12 produced by Listeria-induced macrophages. Science. 1993; 260:547–549.


4. Gately MK, Warrier RR, Honasoge S, Carvajal DM, Faherty DA, Connaughton SE, Anderson TD, Sarmiento U, Hubbard BR, Murphy M. Administration of recombinant IL-12 to normal mice enhances cytolytic lymphocyte activity and induces production of IFN-gamma in vivo. Int Immunol. 1994; 6:157–167.


5. Hendrzak JA, Brunda MJ. Antitumor and antimetastatic activity of interleukin-12. Curr Top Microbiol Immunol. 1996; 213(Pt 3):65–83.
6. Nastala CL, Edington HD, McKinney TG, Tahara H, Nalesnik MA, Brunda MJ, Gately MK, Wolf SF, Schreiber RD, Storkus WJ, Recombinant IL-12. Recombinant IL-12 administration induces tumor regression in association with IFN-gamma production. J Immunol. 1994; 153:1697–1706.
7. Brunda MJ, Luistro L, Warrier RR, Wright RB, Hubbard BR, Murphy M, Wolf SF, Gately MK. Antitumor and antimetastatic activity of interleukin 12 against murine tumors. J Exp Med. 1993; 178:1223–1230.


8. Leonard JP, Sherman ML, Fisher GL, Buchanan LJ, Larsen G, Atkins MB, Sosman JA, Dutcher JP, Vogelzang NJ, Ryan JL. Effects of single-dose interleukin-12 exposure on interleukin-12-associated toxicity and interferon-gamma production. Blood. 1997; 90:2541–2548.
9. Atkins MB, Robertson MJ, Gordon M, Lotze MT, DeCoste M, DuBois JS, Ritz J, Sandler AB, Edington HD, Garzone PD, Mier JW, Canning CM, Battiato L, Tahara H, Sherman ML. Phase I evaluation of intravenous recombinant human interleukin 12 in patients with advanced malignancies. Clin Cancer Res. 1997; 3:409–417.
10. Car BD, Eng VM, Lipman JM, Anderson TD. The toxicology of interleukin-12: a review. Toxicol Pathol. 1999; 27:58–63.


12. Chang CJ, Tai KF, Roffler S, Hwang LH. The immunization site of cytokine-secreting tumor cell vaccines influences the trafficking of tumor-specific T lymphocytes and antitumor efficacy against regional tumors. J Immunol. 2004; 173:6025–6032.


13. Ji J, Li J, Holmes LM, Burgin KE, Yu X, Wagner TE, Wei Y. Synergistic anti-tumor effect of glycosylphosphatidylinositol-anchored IL-2 and IL-12. J Gene Med. 2004; 6:777–785.


14. Tahara H, Lotze MT. Antitumor effects of interleukin-12 (IL-12): applications for the immunotherapy and gene therapy of cancer. Gene Ther. 1995; 2:96–106.
15. Kim YS, Sonn CH, Paik SG, Bothwell AL. Tumor cells expressing membrane-bound form of IL-4 induce antitumor immunity. Gene Ther. 2000; 7:837–843.


16. Chang MR, Lee WH, Choi JW, Park SO, Paik SG, Kim YS. Antitumor immunity induced by tumor cells engineered to express a membrane-bound form of IL-2. Exp Mol Med. 2005; 37:240–249.


17. Lim HY, Ju HY, Chung HY, Kim YS. Antitumor effects of a tumor cell vaccine expressing a membrane-bound form of the IL-12 p35 subunit. Cancer Biol Ther. 2010; 10:336–343.


18. Lim H, Do SA, Park SM, Kim YS. Tumor Cell Clone Expressing the Membrane-bound Form of IL-12p35 Subunit stimulates antitumor immune responses dominated by CD8(+) T Cells. Immune Netw. 2013; 13:63–69.


19. D'Andrea A, Rengaraju M, Valiante NM, Chehimi J, Kubin M, Aste M, Chan SH, Kobayashi M, Young D, Nickbarg E. Production of natural killer cell stimulatory factor (interleukin 12) by peripheral blood mononuclear cells. J Exp Med. 1992; 176:1387–1398.
20. Podlaski FJ, Nanduri VB, Hulmes JD, Pan YC, Levin W, Danho W, Chizzonite R, Gately MK, Stern AS. Molecular characterization of interleukin 12. Arch Biochem Biophys. 1992; 294:230–237.


21. Pan WY, Lo CH, Chen CC, Wu PY, Roffler SR, Shyue SK, Tao MH. Cancer immunotherapy using a membrane-bound interleukin-12 with B7-1 transmembrane and cytoplasmic domains. Mol Ther. 2012; 20:927–937.


22. Lode HN, Dreier T, Xiang R, Varki NM, Kang AS, Reisfeld RA. Gene therapy with a single chain interleukin 12 fusion protein induces T cell-dependent protective immunity in a syngeneic model of murine neuroblastoma. Proc Natl Acad Sci U S A. 1998; 95:2475–2480.

