Abstract
Respiratory syncytial virus (RSV) infection is recognized by the innate immune system through Toll like receptors (TLRs) and retinoic acid inducible gene I. These pathways lead to the activation of type I interferons and resistance to infection. In contrast to TLRs, very few studies have examined the role of NOD-like receptors in viral recognition and induction of adaptive immune responses to RSV. Caspase-1 plays an essential role in the immune response via the maturation of the proinflammatory cytokines IL-1β and IL-18. However, the role of caspase-1 in RSV infection in vivo is unknown. We demonstrate that RSV infection induces IL-1β secretion and that caspase-1 deficiency in bone marrow derived dendritic cells leads to defective IL-1β production, while normal RSV viral clearance and T cell responses are observed in caspase-1 deficient mice following respiratory infection with RSV. The frequencies of IFN-γ producing or RSV specific T cells in lungs from caspase-1 deficient mice are not impaired. In addition, we demonstrate that caspase-1 deficient neonatal or young mice also exhibit normal immune responses. Furthermore, we find that IL-1R deficient mice infected with RSV exhibit normal Th1 and cytotoxic T lymphocytes (CTL) immune responses. Collectively, these results demonstrate that in contrast to TLR pathways, caspase-1 might not play a central role in the induction of Th1 and CTL immune responses to RSV.
Respiratory syncytial virus (RSV) is classified in the Paramyxoviridae family, and is an enveloped, cytoplasmic virus that contains single-stranded, negative sense RNA (1). RSV is known globally as a common cause for the hospitalization of children younger than two years for lung and respiratory tract infections. In some cases, the infection in children can be severe, but immunocompromised or elderly adults can also be seriously vulnerable to RSV infection, which causes severe lower respiratory tract disease and a high mortality rate (2,3).
Caspase-1 is a component of inflammasomes with NOD-like receptors (NLRs) and their adaptor proteins, and is activated within inflammasomes in response to various stressor molecules such as pathogen-associated molecular patterns and damage-associated molecular patterns (4,5,6,7). Caspase-1 is an intracellular cysteine protease that plays an essential role in proinflammatory responses by processing and secreting IL-1 cytokines including IL-1β and IL-18 via cleavage from inactive pro-forms to bioactive forms (8). IL-1β recruits inflammatory cells to infection sites and generates fever, whereas IL-18 is involved in the production of IFN-γ and improvement of the cytolytic activity of NK cells (9,10). Some studies demonstrated caspase-1 is required for innate immune responses against influenza virus or bacterial infection (11,12,13,14)
Furthermore, caspase-1 is crucial for the adaptive immune response. Previous studies demonstrated that the influenza virus stimulates the NLR protein (NLRP) 3 inflammasome through the M2 protein, and that caspase-1 and apoptosis-associated speck-like protein containing a carboxy-terminal caspase activation and recruitment domain (ASC) is required for antiviral immunity by Th1, CTL responses, and the secretion of IgA and IgG antibodies (15,16). Conversely, a separate study showed that caspase-1 is not required for early virus clearance and adaptive immunity against influenza virus infection (12).
Many studies have indicated that RSV also induces the inflammasome, leading to the activation of caspase-1 and the production of IL-1β. RSV-infected human alveolar epithelial cells and neonatal monocytes induce the expression of caspase-1 or IL-1β genes, implying that the inflammasome is related to RSV infection (17,18). The NLRP3/ASC inflammasome activates caspase-1, leading to IL-1β production during RSV infection (19). However, the role of caspase-1 in the recognition of RSV infection in vivo and generation of protective adaptive immunity remains unknown.
In this study, we examine the role of caspase-1 in the initiation of adaptive immunity after mucosal infection with RSV. We demonstrate that the establishment of Th1 and CTL responses to respiratory RSV infection does not require caspase-1 inflammasomes or IL-1 receptor signals. Furthermore, we demonstrate that caspase-1 is not required for early viral clearance and adaptive immunity against RSV infection, regardless of the age of mice. Collectively, our data indicate that there was no in vivo requirement for caspase-1 in the adaptive immune response to RSV infection.
The caspase-1-/- and IL-1R-/- mice used in this study have been previously reported (20,21). These mice with C57BL/6 background were obtained from A. Iwasaki (Yale University, New Haven, CT, USA) and maintained in a specific pathogen-free facility at the Korea Advanced Institute of Science and Technology (KAIST). All animal procedures were performed in accordance with the guidelines and policies for animal experimentation provided by the KAIST Institutional Animal Care and Use Committee (IACUC). The study protocol was approved by the KAIST IACUC.
The A2 strain of RSV was kindly provided by Dr. Jun Chang (Ewha Womans University, Seoul, Korea). The RSV A2 strain propagated in HEp-2 cells was used in all experiments. Eight to twelve- and three-week mice were anesthetized with ketamine (100 mg/kg) and xylazine (5.83 mg/kg) via intraperitoneal injection, and were injected intranasally with 1×107 pfu/20 µl or 1×106 pfu/10 µl of RSV, respectively. One-week old mice were anesthetized with isoflurane and inoculated intranasally with 1×106 pfu/5 µl of RSV.
GM-CSF-derived BM dendritic cells (BMDCs), or M-CSF-derived BM macrophages (BMMs) were prepared according to published procedures (15). For the differentiation of BMDCs and BMMs, bone marrow cells were cultured in the presence of GM-CSF or M-CSF in RPMI 1640 media containing penicillin/streptomycin and 10% FBS (Hyclone, Logan, UT, USA), respectively, for 6 days by replenishing with fresh media. BMDCs (2×105) or BMMs (5×105) were stimulated with RSV (moi=1, 5, respectively), 2.5 µg/ml CpG2216 (Invitrogen, Carlsbad, CA, USA) and 100 ng/ml LPS (Sigma-Aldrich, St. Louis, MO, USA) in a 96-well flat bottom plate (NUNC, Denmark) or 24-well plate (NUNC) at 37℃ and 5% CO2. After 20 hr, the supernatants and cells were harvested and analyzed by ELISA and FACS, respectively.
The concentration of IL-6, IL-12p40, and IL-1β in supernatants from stimulated BMDCs and BMMs were measured in duplicate by ELISA in accordance with the manufacturer's instructions. Anti-mouse IL-6 (BD Bioscience, San Diego, CA, USA, MP5-20F3), IL-12 (BD Bioscience, C15.6), or IL-1β (BD Bioscience, B122) was used as the capture antibody, and biotin-labeled anti-mouse IL-6 (BD Bioscience, MP5-32C11), IL-12p40 (BD Bioscience, C17.8), or IL-1β (eBioscience, San Diego, CA, USA, polyclonal) was used as the secondary antibody.
Eight days following RSV infection, CD4+ T cells and CD8+ T cells were isolated from the mouse spleens using anti-CD4 micro beads or anti-CD8 micro beads (Miltenyi Biotec, Auburn, CA, USA) in accordance with the manufacturer's instructions. CD4+ T and CD8+ T cells (2×105) were re-stimulated with heat-inactivated RSV or RSV M197-195 peptide, pulsed with irradiated APCs (3×105), and cultured for 72 hr at 37℃ in 96-well U-bottom plates (BD falcon, Durham, NC, USA). IFN-γ production in the supernatants was measured by ELISA with anti-mouse IFN-γ (eBioscience, XMG1.2) as the capture antibody and biotin-labeled anti-mouse IFN-γ (eBioscience, R4-6A2) as the secondary antibody.
The lungs from RSV infected mice were minced using a razor blade and incubated for 30 min in FACS buffer (1% FBS) containing collagenase IV (2 mg/ml) and DNase I (30 µg/ml) in a water bath at 37℃. The lungs were then incubated in HBSS media containing 5% FBS (Hyclone) and 0.5 M EDTA. Tissue fragments were disrupted using a syringe and passed through a 70 µm cell strainer (SPL, Pocheon, Korea). Using Percoll (GE Healthcare, Amersham, UK), the lung cells were collected and treated with ACK lysis buffer for 5 min at room temperature to remove RBCs.
The stimulated BMDC and BMM cells were collected and stained with FITC-labeled anti-MHC II (M5/114.15.2), PE-labeled anti-CD86 (GL1), Percp-cy5.5 labeled anti-CD11b (M1/70) and APC labeled anti-CD11c (HL3). In addition, the PE-labeled anti-Rat IgG2a was used as an isotype control.
To visualize the IFN-γ+ T cells, 1×106 lung cells were placed in a 96-well U-bottom plate (BD falcon) and re-stimulated with PMA (50 ng/ml), ionomycin (1 µg/ml), or RSV M187-195 peptide (5 µg/ml) with Golgi stop (0.7 µl/ml) and incubated at 37℃ in 5% CO2. The cells were harvested after 5 hr. Single cells were first stained on the surface with FITC-labeled anti-CD8 (BD Bioscience, 53-6.7), PE-labeled anti-CD44 (BD Bioscience, IM7), and PerCP-Cy5.5 labeled anti-CD4 (Biolegend, San Diego, CA, USA, GX1.5), then fixed and permeabilized using a BD Cytofix/Cytoperm buffer for 30 min on ice. Finally, cells were stained for intracellular IFN-γ using APC-labeled anti-IFN-γ* (XMG1.2).
For the RSV tetramer staining, 1×106 lung cells were first stained with FITC-labeled anti-CD3 (BD Bioscience, 17A2) and PE-labeled anti-CD8 (Biolegend, 53-6.7), followed by staining with APC-labeled RSV tetramer (H-2Db M187-195) for 30 min on ice. After staining, the cells were washed with FACS buffer three times. Flow cytometry was performed using a FACS Calibur™ cell analyzer (BD Biosciences). All samples were pretreated with FcR blocker (Tonbo, San Diego, CA, USA, 2.4G2) and flow cytometry was performed using FACS Calibur™. The data were analyzed using FlowJo software for the Mac.
On days 5 and 10 after RSV infection, the mice were sacrificed and the lungs were collected. After obtaining the weight, the lungs were disrupted by a syringe and passed through a 70 µm cell strainer (SPL) in 2 ml PBS, and the supernatants were collected. HEp2 cells were infected with supernatants, and incubated for 6 days as previously described (22). The RSV titer was calculated by counting the plaques on HEp2 cells.
All data were analyzed using Prism 9 (Graph-pad software Inc. La Jolla, CA, USA). Error bars represent the standard error of the mean (SEM) or the standard deviation (SD). The p-values were calculated using a two-sided, unpaired Student t-test. Differences were considered statistically significant when p<0.05, *, p<0.05; **, p<0.01; ns, not significant.
The primary target cells of RSV infection in the lung are the respiratory epithelial cells that can subsequently infect alveolar macrophages and DCs (6). In order to dissect the importance of caspase-1 in response to RSV infection, we first examined IL-1β secretion in candidate cell types such as BMDCs and BMMs. BMDCs and BMMs were differentiated from the bone marrow of caspase-1+/- and caspase-1-/- mice and stimulated with RSV. While normal BMDCs stimulated with RSV produced a high level of IL-1β, caspase-1-deficient BMDCs rarely produced IL-1β (Fig. 1A). Unlike BMDCs, BMMs did not produce IL-1β, regardless of caspase-1 (Fig. 1D). This result suggests that RSV infection leads to the production of IL-1β, and that caspase-1 plays an essential role in IL-1β secretion.
Next, we analyzed BMDC and BMM CD86 costimulatory molecules. The expression level of CD86 on BMDCs and BMMs was increased, but the degree of CD86 up-regulation was similar between caspase-1+/- and caspase-1-/- mice (Fig. 1G and H). The expression of the proinflammatory cytokines IL-6 and IL-12p40 in stimulated BMDCs (Fig. 1B and C) and BMMs (Fig. 1E and F) were also comparable, regardless of caspase-1. These experiments demonstrated that caspase-1 is not involved in the up-regulation of costimulatory molecules and the production of proinflammatory cytokines, except for IL-1β.
Inflammasomes play a crucial role in the innate immune response to viral infection through the recognition of viral RNA. In addition, IL-lβ activates lymphocytes and recruits leukocytes to the site of infection (23). Therefore, to investigate whether caspase-1 is related to RSV viral clearance, we measured viral replication in the lungs via a plaque assay at early and late time points after infection. No differences were detected in the viral titer at either day 5 or day 10 post infection (Fig. 2A).
To determine the effect of capase-1 in the adaptive immune response against RSV infection, we performed a co-culture assay. Mice 10~12 weeks in age were infected with 1×107 pfu of RSV, and after 8 days, CD4+ and CD8+ T cells in the spleen were re-stimulated with heat-inactivated RSV or RSV M197-195 peptide pulse-irradiated APCs. IFN-γ production of CD4+ T cells was normal, but IFN-γ from the cytotoxic CD8+ T cells appeared higher in capase-1-/- mice than in littermate control mice (Fig. 2B). However, the CTL responses were similar in caspase-1 deficient mice following infection with 5×106 pfu of RSV (Data not shown).
Next, we measured IFN-γ-producing T cells in lungs by intracellular staining after re-stimulation with either PMA/inomycin or RSV M197-195 peptide. The frequencies of IFN-γ-producing CD4+ and CD8+ T cells in the lungs from caspase-1-/- mice were comparable to frequencies observed in lungs from caspase-1+/- mice (Fig. 2C and D). In addition, the frequency of RSV-specific CD8+ T cells in the lungs was normal in caspase-1-/- mice (Fig. 2E). These results indicated that caspase-1 is not required for either the innate or adaptive immune response to RSV.
RSV infections in the lungs and airway tracks of infants and young children aged two years are more common than infections in adults. Consequently, we used 1-week or 3-week old mice as a model of neonatal and young mice, respectively, instead of adult mice, which are older than 8 weeks. No significant differences were identified in the viral titer of lungs from 3-week old mice (Fig. 3A). The viral titer in 1-week old caspase-1-/- mice was lower than caspase-1+/- mice at day 5 (Fig. 3B), but RSV was almost entirely cleared by day 10 in both 3-week or 1-week old mice (Fig. 3A and B).
Consistent with the results observed in adult mice, the Th1 and CTL responses detected in 3- or 1-week old caspase-1-deficient mice were normal (Fig. 4A and B). Further, when compared to littermate controls, caspase-1-/- mice exhibited a similar frequency of IFN-γ-producing T cells re-stimulated with PMA/inomycin or RSV M197-195 peptide (Fig. 4C-F), as well as RSV-specific CD8+ T cells (Fig. 4G and H). Collectively, the results indicated that immune responses in young and neonatal mice were unaffected by caspase-1 deficiency during RSV infection.
Following activation by caspase-1, secreted IL-1β binds to the IL-1R receptor (IL-1R) with the IL-1R accessory protein, and activates NF-κB, which is a transcription factor for the expression of proinflammatory cytokines through MyD88 dependent IL-1R signaling (24). To investigate whether IL-1R signaling was required for RSV infection, we analyzed adaptive immune responses in RSV-infected IL-1R-/- mice. The Th1 response was slightly decreased in IL-1R-/- mice, but the CTL response was normal compared to IL-1R+/- mice (Fig. 5A and 5B). Similar to the results obtained in caspase-1-/- mice, a comparable number of IFN-γ producing CD4+ and CD8+ T cells existed in the lungs of both the IL-1R+/- and IL-1R-/- mice (Fig. 5C and D). The results suggested that IL-1R signaling does not affect adaptive antiviral immunity against RSV infection.
In this study, we have demonstrated that caspase-1 is not required in the antiviral immune response against RSV infection. Several previous studies have demonstrated that RSV induces IL-1β secretion from various cell types (17,18,19). Using an in vitro assay, we confirmed that BMDCs infected with RSV produced IL-1β. Also, we demonstrated that IL-1β production from caspase-1 deficient BMDCs was significantly reduced compared to normal BMDCs, but the production of the proinflammatory cytokines IL-6 and IL-12p40 was normal. In addition, the expression of the costimulatory molecule CD86 was comparably up-regulated in response to RSV. Thus, caspase-1 is required for IL-1β production, but not for the production of IL-6, IL-12p40, or the up-regulation of costimulatory molecules during RSV infection.
Previous research has established that caspase-1 is essential for protection against infection by influenza, which is negative-sense ssRNA virus (11,12,15,25). Interestingly, caspase-1 has no effect on either innate or adaptive immunity in subsequent RSV challenges. The RSV titer in the lungs peaks on days 4 to 6 (26). However, the RSV titer in the current study was not different on day 5. Further, RSV was almost eliminated by day 10 in both normal and caspase-1 deficient mice. T cells, especially CD8+ T cells, play a major role in RSV clearance during primary infections (27). Additionally, IFN-γ is a primary molecule in CD8+ T cell-mediated immune responses to RSV infection (28). As expected, the frequency of IFN-γ-producing or RSV-specific T cells in the lungs of caspase-1-/- mice were no different to frequencies observed in caspase-1+/- mice. The CTL response appeared slightly higher in caspase-1-/- mice, but the results differed depending on the RSV infection dose. Overall, the adaptive responses against RSV infection were unaffected by caspase-1.
IL-18 processed by caspase-1 is essential for the induction of IFN-γ production by T cells and NK cells and for Th1 responses (29,30). However, based on the current results, IL-18 may not be responsible for the immune response to RSV.
Next, we determined that caspase-1 deficient neonatal and young mice also exhibit normal viral clearance and T cell immune responses to RSV. Neonatal mice have fewer immune cells, which lead to poor protective immune responses because of their developmentally immature immune systems, as well as CD4+ T cell responses that are skewed towards a Th2 response (31). The immune response in RSV-infected neonatal mice also shows a stronger Th2 than Th1 response, and fewer IFN-γ-producing CD8+ T cells (32). Consistent with previous studies, we confirmed that younger mice have reduced T cell-induced IFN-γ secretion in the lungs following RSV infection. Using IL-1R-/- mice, we likewise demonstrated that the IL-1R signaling pathway by IL-1β is not required following RSV infection, which confirmed the notion that caspase-1 does not play an important role in the immune response to RSV.
In conclusion, our study demonstrated that caspase-1 inflammasomes are not required for the development of adaptive immune responses to respiratory RSV infection. In addition, our data suggested that stimulants of caspase-1 inflammasomes may not provide a candidate adjuvant for the development of an RSV vaccine.
Figures and Tables
Figure 1
The upregulation of CD86 and proinflammatory cytokines, but not IL-1β, are not impaired in caspase-1 deficient BMDCs and BMMs. BMDCs and BMMs were differentiated from the bone marrow of wild type and caspase-1-/- mice and stimulated with RSV (moi=1, 5), 2.5 µg/ml CpG2216, and 100 ng/ml LPS. After 20 hr, IL-1β, IL-6, and IL-12p40 in supernatants from BMDCs (A~C) and BMMs (D~F) were measured by ELISA. CD86 levels from BMDCs (G) and BMMs (H) were analyzed by flow cytometry (red line=caspase-1+/-; blue line=caspase-1-/-). Data are representative of two independent experiments. Error bars indicate SD.
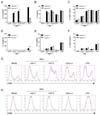
Figure 2
Caspase-1 does not affect RSV viral clearance or the adaptive immune response in adult mice. Caspase-1+/- and caspase-1-/- mice (10~12 weeks) were infected intranasally with 1×107 pfu of RSV. Caspase-1+/- mice were infected with PBS as a mock control (C, D). (A) On days 5 or 10 post infection, the RSV titer in the lungs was measured by plaque assay on HEp2 cells. (B~E) On day 8 post RSV infection, mice were sacrificed. (B) Isolated CD4+ and CD8+ T cells from the mouse spleens were stimulated with the indicated heat-inactivated RSV or RSV M187-195 peptide, respectively, and pulsed with irradiated APCs. After 72 hr, IFN-γ in the supernatants from CD4+ T cells (left) and CD8+ T cells (right) was measured by ELISA. (C, D) IFN-γ-producing CD4+ T cells (C, up) and CD8+ T cells (C, down and D) were detected in the lungs by intracellular staining after re-stimulation with (C) PMA and ionomycin or (D) RSV M187-195 peptide. (E) RSV-specific CD8+ T cells were stained with RSV tetramer (H-2Db M187-195). Data are representative of three independent experiments, and error bars indicate SEM.
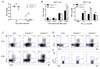
Figure 3
Caspase-1 is not required for RSV viral clearance in young mice. Caspase-1+/- and caspase-1-/- mice (1- or 3-weeks) were infected intranasally with 1×106 pfu of RSV. On days 5 or 10 post infection, the RSV titer in the lungs from 3 week mice (A) and 1 week mice (B) were measured by plaque assay on HEp2 cells.
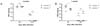
Figure 4
In 3-week- and 1-week-old mice, the caspase-1-/- mice T cell responses are normal. Three week (A, C, E, G) or one week (B, D, F, H) old caspase-1+/- and caspase-1-/- mice were intranasally infected with 1×106 pfu of RSV. Caspase-1+/- mice were infected with PBS as a mock control (C~H). On day 8 after RSV infection, the mice were sacrificed. (A, B) CD4+ and CD8+ T cells from the mouse spleens were stimulated with the indicated heat-inactivated RSV or RSV M187-195 peptide, respectively, and pulsed with irradiated APCs. After 72 hr, IFN-γ in supernatants from the CD4+ T cells (left) and CD8+ T cells (right) were measured by ELISA. (C, D) IFN-γ-producing CD4+ T cells (up) and CD8+ T cells (down) were detected in the lungs by intracellular staining after re-stimulation with PMA and ionomycin or (E, F) RSV M187-195 peptide. (G, H) RSV-specific CD8+ T cells were stained with RSV tetramer (H-2Db M187-195) (G: 3-week, H: 1-week). Data are representative of two to three independent experiments. Error bars indicate SD.
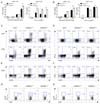
Figure 5
IL-1R signaling is not required for the induction of Th1 and CTL priming against RSV infection. IL-1R+/- and IL-1R-/- mice were intranasally infected with 1×107 pfu of RSV. IL-1R+/- mice were infected with PBS as a mock control (C, D). On day 8 after RSV infection, mice were sacrificed. Isolated CD4+ and CD8+ T cells from the mouse spleens were stimulated with the indicated heat-inactivated RSV or RSV M187-195 peptide, respectively, and pulsed with irradiated APCs. After 72 hr, the IFN-γ in the supernatants from CD4+ T cells (A) and CD8+ T cells (B) were measured by ELISA. The absolute numbers of IFN-γ producing CD4+ T cells (C) and CD8+ T cells (D) were detected in the lungs by intracellular staining after re-stimulation with PMA and ionomycin. Data are representative of two to three independent experiments. Error bars indicate SD.
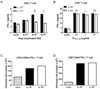
References
1. Collins PL. The molecular biology of human respiratory syncytial virus (RSV) of the genus Pneumovirus. New York: Springer. Plenum Press;1991. p. 103–162.
2. Collins PL, Graham BS. Viral and host factors in human respiratory syncytial virus pathogenesis. J Virol. 2008; 82:2040–2055.


3. Falsey AR, Hennessey PA, Formica MA, Cox C, Walsh EE. Respiratory syncytial virus infection in elderly and high-risk adults. N Engl J Med. 2005; 352:1749–1759.


4. Sollberger G, Strittmatter GE, Garstkiewicz M, Sand J, Beer HD. Caspase-1: the inflammasome and beyond. Innate Immun. 2014; 20:115–125.


5. Case CL. Regulating caspase-1 during infection: roles of NLRs, AIM2, and ASC. Yale J Biol Med. 2011; 84:333–343.
6. Kim TH, Lee HK. Differential roles of lung dendritic cell subsets against respiratory virus infection. Immune Netw. 2014; 14:128–137.


7. Kim TH, Lee HK. Innate immune recognition of respiratory syncytial virus infection. BMB Rrep. 2014; 47:184–191.


8. Franchi L, Eigenbrod T, Munoz-Planillo R, Nunez G. The inflammasome: a caspase-1-activation platform that regulates immune responses and disease pathogenesis. Nat Immunol. 2009; 10:241–247.


9. Arend WP, Palmer G, Gabay C. IL-1, IL-18, and IL-33 families of cytokines. Immunol Rev. 2008; 223:20–38.


10. Dinarello CA. Immunological and inflammatory functions of the interleukin-1 family. Annu Rev Immunol. 2009; 27:519–550.


11. Allen IC, Scull MA, Moore CB, Holl EK, Elvania-TeKippe E, Taxman DJ, Guthrie EH, Pickles RJ, Ting JP. The NLRP3 inflammasome mediates in vivo innate immunity to influenza A virus through recognition of viral RNA. Immunity. 2009; 30:556–565.


12. Thomas PG, Dash P, Aldridge JR Jr, Ellebedy AH, Reynolds C, Funk AJ, Martin WJ, Lamkanfi M, Webby RJ, Boyd KL, Doherty PC, Kanneganti TD. The intracellular sensor NLRP3 mediates key innate and healing responses to influenza A virus via the regulation of caspase-1. Immunity. 2009; 30:566–575.


13. Fang R, Tsuchiya K, Kawamura I, Shen Y, Hara H, Sakai S, Yamamoto T, Fernandes-Alnemri T, Yang R, Hernandez-Cuellar E, Dewamitta SR, Xu Y, Qu H, Alnemri ES, Mitsuyama M. Critical roles of ASC inflammasomes in caspase-1 activation and host innate resistance to Streptococcus pneumoniae infection. J Immunol. 2011; 187:4890–4899.


14. Miao EA, Leaf IA, Treuting PM, Mao DP, Dors M, Sarkar A, Warren SE, Wewers MD, Aderem A. Caspase-1-induced pyroptosis is an innate immune effector mechanism against intracellular bacteria. Nat Immunol. 2010; 11:1136–1142.


15. Ichinohe T, Lee HK, Ogura Y, Flavell R, Iwasaki A. Inflammasome recognition of influenza virus is essential for adaptive immune responses. J Exp Med. 2009; 206:79–87.


16. Ichinohe T, Pang IK, Iwasaki A. Influenza virus activates inflammasomes via its intracellular M2 ion channel. Nat Immunol. 2010; 11:404–410.


17. Takeuchi R, Tsutsumi H, Osaki M, Haseyama K, Mizue N, Chiba S. Respiratory syncytial virus infection of human alveolar epithelial cells enhances interferon regulatory factor 1 and interleukin-1beta-converting enzyme gene expression but does not cause apoptosis. J Virol. 1998; 72:4498–4502.


18. Takeuchi R, Tsutsumi H, Osaki M, Sone S, Imai S, Chiba S. Respiratory syncytial virus infection of neonatal monocytes stimulates synthesis of interferon regulatory factor 1 and interleukin-1beta (IL-1beta)-converting enzyme and secretion of IL-1beta. J Virol. 1998; 72:837–840.


19. Segovia J, Sabbah A, Mgbemena V, Tsai SY, Chang TH, Berton MT, Morris IR, Allen IC, Ting JP, Bose S. TLR2/MyD88/NF-kappaB pathway, reactive oxygen species, potassium efflux activates NLRP3/ASC inflammasome during respiratory syncytial virus infection. PLoS One. 2012; 7:e29695.
20. Kuida K, Lippke JA, Ku G, Harding MW, Livingston DJ, Su MS, Flavell RA. Altered cytokine export and apoptosis in mice deficient in interleukin-1 beta converting enzyme. Science. 1995; 267:2000–2003.


21. Glaccum MB, Stocking KL, Charrier K, Smith JL, Willis CR, Maliszewski C, Livingston DJ, Peschon JJ, Morrissey PJ. Phenotypic and functional characterization of mice that lack the type I receptor for IL-1. J Immunol. 1997; 159:3364–3371.
22. Kim S, Joo DH, Lee JB, Shim BS, Cheon IS, Jang JE, Song HH, Kim KH, Song MK, Chang J. Dual role of respiratory syncytial virus glycoprotein fragment as a mucosal immunogen and chemotactic adjuvant. PLoS One. 2012; 7:e32226.


25. Pang IK, Iwasaki A. Inflammasomes as mediators of immunity against influenza virus. Trends Immunol. 2011; 32:34–41.


26. Taylor G, Stott EJ, Hughes M, Collins AP. Respiratory syncytial virus infection in mice. Infect Immun. 1984; 43:649–655.


27. Graham BS, Bunton LA, Wright PF, Karzon DT. Role of T lymphocyte subsets in the pathogenesis of primary infection and rechallenge with respiratory syncytial virus in mice. J Clin Invest. 1991; 88:1026–1033.


28. Ostler T, Davidson W, Ehl S. Virus clearance and immunopathology by CD8+ T cells during infection with respiratory syncytial virus are mediated by IFN-γ. Eur J Immunol. 2002; 32:2117–2123.


29. Takeda K, Tsutsui H, Yoshimoto T, Adachi O, Yoshida N, Kishimoto T, Okamura H, Nakanishi K, Akira S. Defective NK cell activity and Th1 response in IL-18-deficient mice. Immunity. 1998; 8:383–390.


30. Ghayur T, Banerjee S, Hugunin M, Butler D, Herzog L, Carter A, Quintal L, Sekut L, Talanian R, Paskind M, Wong W, Kamen R, Tracey D, Allen H. Caspase-1 processes IFN-γ-inducing factor and regulates LPS-induced IFN-γ production. Nature. 1997; 386:619–623.


ACKNOWLEDGEMENTS
The authors would like to thank the members of the Laboratory of Host Defense for helpful advice on experiments and data discussions. We thank Dr. J. Chang for critical reagents and technical support. This work was supported by the National Research Foundation (NRF-2014M3A9A5044964, NRF-2013R1A1A2063347, NRF-2012M3A9B4028274) and the KAIST Future Systems Healthcare project from the Ministry of Science, ICT and Future Planning of Korea.